html xmlns=”http://www.w3.org/1999/xhtml” xmlns:mml=”http://www.w3.org/1998/Math/MathML” xmlns:epub=”http://www.idpf.org/2007/ops”>
Introduction
Major depressive disorder (MDD) is an affective disorder characterized by pervasive low mood, which is associated with vegetative, behavioral, physical, and cognitive features. The World Health Organization (WHO) report listed MDD as the fourth largest contributor to global burden of disease (WHO, 2001). While there has been long-standing interest in examining brain gray matter changes in patients with MDD (Drevets, Savitz, & Trimble, 2008; Rigucci, Serafini, Pompili, Kotzalidis, & Tatarelli, 2010), recent studies have sought to investigate the white matter changes underlying MDD and their effects on cognition (Qin et al., 2014; Shizukuishi, Abe, & Aoki, 2013). This approach holds the potential to enhance the detection, assessment, and management of this debilitating condition (Millan et al., 2012). The purpose of this chapter is to provide an overview of white matter neurobiology in MDD. Available evidence from neuroimaging literature for neural circuitry changes underlying MDD is presented with a focus on its impact on cognition. Finally, we conclude the chapter with translational implications of white matter neurobiology and cognitive dysfunction in MDD.
Brain white matter findings in major depressive disorder
Vascular depression
The vascular depression hypothesis by Alexopoulos and colleagues proposed that “cerebrovascular disease may predispose, precipitate, or perpetuate towards the development of certain geriatric depressive syndromes” (Alexopoulos et al., 1997: 918). Evidence in support of this hypothesis includes findings that patients with late-onset MDD exhibit higher rates of, and more widespread, vascular disease-related white matter hyperintensities (WMH), which were associated with greater neuropsychological impairment compared with early-onset MDD. Furthermore, these were associated with poorer response to treatment (Taylor, Aizenstein, & Alexopoulos, 2013). It was also suggested that vascular risk factors can contribute to subcortical ischemic depression whereby cerebrovascular disease affects fronto-striatal circuits underlying the depressive illness (Krishnan et al., 2004). The involvement of the frontolimbic circuits leads to hypometabolism of dorsal cortical regions and hypermetabolism of ventral limbic regions with consequent loss of top-down emotional regulation, focal fiber tract disruptions, altered functional connectivity, and altered regional brain function which can result in MDD (Taylor et al., 2013). The presence of this vascular subtype of depression provides evidence that alterations in cerebral white matter and associated circuits may have an etiological role in MDD.
White matter pathology
Studies comparing the neuropathology of white matter lesions have found a preponderance of ischemic lesions in MDD. In a study by Thomas and colleagues, the WMH in patients with MDD were stained for pro-inflammatory intracellular cell adhesion molecules to assess the extent of ischemia-induced white matter lesions (Thomas et al., 2002). They found that in patients with MDD, 75 percent of WMH lesions were in the dorsolateral prefrontal cortex (DLPFC) and 25 percent occurred in the anterior cingulate, in contrast to controls in which none of the ischemia-induced white matter lesions were located in the DLPFC. The findings were suggestive of neuroinflammation which was supported by several observations including dilated perivascular spaces, oligemic demyelination, and ischemic demyelination.
In younger adults, preliminary evidence suggests white matter pathology, including, but not limited to, reductions in the density and number of glial cells in the frontolimbic brain regions (e.g. subgenual region of the anterior cortex, DLPFC, and orbitofrontal cortex). In addition, there was an increase in glial cell density in hippocampal cornu ammonis (CA) subfields and the granule cell layer of the dentate, indicating a reduction in glial processes and reduced hippocampal volume. It was also found that there were decreases in the density of glial fibrillary acidic protein, suggesting less immunoreactive astrocytes that may account for alterations in glutamate/gamma-aminobutyric acid (GABA) neurotransmission (Rajkowska & Miguel-Hidalgo, 2007).
Patients with MDD also exhibited reductions in the density and ultrastructure of oligodendrocytes in the prefrontal cortex and amygdala; moreover, decreases in glial cell number and density were found in the deeper prefrontal cortical layers (Cotter et al., 2002). Lower density of oligodendrocytes and decreases in the expression of genes related to oligodendrocyte function have been reported to occur in the temporal cortices of patients with MDD (Beasley, Honavar, Everall, & Cotter, 2009). In the anterior frontal cortex of patients with MDD, there are also reduced levels of myelin basic protein, a marker for mature oligodendrocytes (Johnston-Wilson et al., 2000). Taken together, these pathological changes support the view that white matter pathology underlyies MDD.
White matter genes
Expression of the myelination gene was noted to be significantly lower in the temporal cortices of patients with MDD (Aston, Jiang, & Sokolov, 2004). These genes relate specifically to structural components of myelin (i.e. CNP, MAG, MAL, MOG, MOBP, PMP22), synthesis of myelin constituents (i.e. ASPA and UGT8), regulation of myelin formation (i.e. ENPP2, EDG2, TF, and KLK6), and axonal guidance, such as NogoB, and should be examined further to determine their functional roles and epistatic interactions with other related genes (Tham, Woon, Sum, Lee, & Sim, 2011). Recent findings from genome-wide association studies of cerebral white matter lesion burden in a large European community-based healthy cohort identified several novel risk-associated single-nucleotide polymorphisms (SNPs) in a locus on chromosome 17q25 covering known genes including WBP2, TRIM65, TRIM47, MRPL38, FBF1, and ACOX1 (Fornage et al., 2011). Earlier RNA microarray analysis to examine functional pathways and mechanisms underlying white matter lesions found eight major pathways within which different genes were noted with altered RNA transcription in white matter lesions and involving processes such as metabolism, proteolysis, immune regulation, cell cycle, apoptosis, ion transport, cell structure, and electron transport (Simpson et al., 2009). Using white matter lesion as a complex phenotype, further characterization of the genetic and genomic correlates can potentially provide new insights into the pathogenesis of the underlying white matter lesions frequently observed in disorders including, but not limited to, MDD.
Neuroimaging findings
Several neuroimaging studies have been conducted in individuals with late-onset MDD(i.e. ≥ 55 years of age). Magnetization transfer imaging found that patients with MDD had lower magnetization ratios, indicating lower integrity of the macromolecular protein pool, in the genu and splenium of the corpus callosum, right caudate nucleus, putamen, and occipital white matter (Kumar et al., 2004). Using magnetic resonance spectroscopy, it has been observed that patients with MDD had lower NAA/creatine ratio in left frontal white matter and higher cho/creatine and myoinositol/creatine ratios compared with controls (Chen et al., 2009). Moreover, white matter lesions tended to occur more commonly in the left superior longitudinal fasciculus and right frontal projections of the corpus callosum in patients with MDD (Tham et al., 2011).
In adults with an earlier onset (i.e. between 35 and 55 years old) MDD, subcortical WMH load predicts poor response to antidepressant treatment (Papakostas et al., 2005). In younger adults with MDD, decreases in fractional anisotropy (FA) were observed in the left brain hemisphere involving the anterior limb of the internal capsule and inferior parietal portion of the superior longitudinal fasciculus (Zou et al., 2008). Additionally, FA in the right anterior cingulate and left frontal white matter were negatively correlated with the total number of days spent in episodes of major depression (Abe et al., 2010).
In adults with MDD under 35 years of age, lower FA was observed in several prefrontal regions as well as in the right middle frontal gyrus, left lateral occipito-temporal gyrus, subgyral, and angular gyri of the right parietal lobe compared with controls (Ma et al., 2007). Overall, neuroimaging studies of patients with MDD have reported WMH load to be associated with different clinical features such as time spent in an episode of major depression, folate levels, and antidepressant response. Lower FA found in diverse brain regions implicates anomalies of white matter integrity and cortical–subcortical circuitry with associated neuronal injury related to white matter changes.
Neural circuitry and cognitive dysfunction in depression
Brain function is subserved not only by the computations of individual brain regions, but also the circuits formed from networks of brain regions. The white matter tracts form the highway for information transfer within these circuitries. The components of circuit dysfunction in MDD comprise regions that display structural and functional deficits. Meta-analyses of structural magnetic resonance imaging (MRI) studies of patients with MDD have demonstrated gray matter reductions most consistently in the rostral anterior cingulate cortex (ACC) as well as in dorsolateral, dorsomedial, and right inferior prefrontal cortices; limbic areas, such as the amygdala, parahippocampus, and hippocampus; and subcortical areas such as the putamen, caudate, and thalamus (Sacher et al., 2012). In positron emission tomography (PET) studies, patients with MDD displayed increased glucose metabolism in the right subgenual and pregenual ACC. Antidepressants appear to increase the size of the orbitofrontal and subgenual ACC (Bora, Fornito, Pantelis, & Yücel, 2012a).
Closing in
Functional MRI studies of MDD have reported altered fronto-striatal activation in response to cognitive and emotional tasks. Patients with MDD who are less able to sustain positive emotion appear to exhibit diminished maintenance of prefrontal and striatal activation (Heller et al., 2009). During explicit learning, there is also decreased prefrontal activation in depressed elderly patients; this observation has been associated with increased striatal activation (Aizenstein et al., 2005).
Studies of functional connectivity in MDD document decreased frontal–amygdala connectivity, abnormal effective connectivity across visuo-attentional networks, and altered functional connectivity between anterior cingulate and insular regions (Dannlowski et al., 2009; Johnstone, Van Reekum, Urry, Kalin, & Davidson, 2007; Siegle, Thompson, Carter, Steinhauer, & Thase, 2007). Additionally, Sheline and colleagues demonstrated that a “dorsal nexus,” comprising the dorsomedial prefrontal cortex, comprised a hub with increased connectivity in individuals with MDD, which is related to the default, cognitive control, and affective networks (Sheline, Price, Yan, & Mintun, 2010).
In studies of resting state connectivity, elderly subjects with MDD had significantly lower functional connectivity in BA23 subgenual ACC and higher functional connectivity of the dorsomedial prefrontal cortex and orbitofrontal cortex (Greicius et al., 2007). Those who responded to pharmacotherapy showed increased resting state connectivity of the subgenual ACC and dorsomedial prefrontal cortex with lower activation of the subgenual ACC and higher activation of the rostral ACC and dorsal ACC (Lui et al., 2011; Veer et al., 2010).
Recently, Tao et al. (2011) described an uncoupling of the “hate circuit,” involving the superior frontal gyrus, insula, and putamen in patients with MDD. They also described circuits in risk-taking and action, reward, attention, and memory that were significantly affected in patients with MDD. Patients with MDD had reduced effective connectivity between the insula and putamen bilaterally, as well as between the left superior frontal gyrus and right insula. These brain regions were activated when an individual viewed someone they hated or who had rejected them recently, suggesting that the self-loathing as well as the inability to deal with feelings of hatred and accompanying social withdrawal often experienced by individuals with MDD might be related to disturbances in neural circuitry involving frontal and subcortical nodes. Additionally, the risk/action circuitry comprising the connections of the inferior frontal gyrus, precentral gyrus in the left hemisphere, and supramarginal gyrus in the right hemisphere were all reduced in strength in patients with MDD (Tao et al., 2011).
A feedback loop between the prefrontal cortex and amygdala is one of the core circuit dysfunctions in MDD, wherein the amygdala displays hyperactivity and prefrontal cortex function or efficiency is decreased (Etkin, Egner, Peraza, Kandel, & Hirsch, 2006; Greicius, 2008). Different treatments for MDD appeared to act on different components of the aforementioned circuit. Within this circuit, it was thought that antidepressants acted directly on amygdala hyperactivity, while cognitive therapies targeted prefrontal cortex function (Lui et al., 2011). Indeed, response to cognitive therapy is associated with decreased resting state activity in the prefrontal cortex and increased resting state in the hippocampus and dorsal cingulate cortex, possibly reflecting a resetting of tonic prefrontal activity to yield greater capacity for “top-down” emotion regulation. On the other hand, response to paroxetine treatment led to an increase in prefrontal activation and a decrease in hippocampal and subgenual cingulate activation, indicating tonic increases in subcortical cingulate metabolism leading to a “bottom-up” effect from the limbic system (DeRubeis, Siegle, & Hollon, 2008).
The subgenual cingulate cortex appears to be a central node in MDD with convergent evidence across a range of interventions (Crowell, Riva-Posse, Garlow, & Mayberg, 2014). Changes in metabolic activity of the subgenual cingulate cortex have been observed with selective serotonin reuptake inhibitors as well as serotonin-norepinephrine reuptake inhibitors in combination with electroconvulsive therapy (ECT) or cognitive behavioral therapy (CBT) (McCormick et al., 2007). When the over-active subgenual cingulate cortex was suppressed by deep brain stimulation, the neural circuitry comprising the orbitofrontal cortex, dorsomedial prefrontal cortex, and hypothalamus exhibited decreased blood flow and the mid-cingulate cortex and substantia nigra exhibited increased flow when measured six months later (Crowell et al., 2014; Johansen-Berg et al., 2008; Mayberg, 2009; Mayberg et al., 2005).
A meta-analysis of neuroimaging studies (Bora, Harrison, Davey, Yücel, & Pantelis, 2012b) demonstrated deficits in the cortico–striatal–pallidal–thalamic circuits, with volume reductions in the prefrontal, anterior cingulate, caudate, and putamen. In medicated patients, volume deficits in the subgenual anterior cingulate and orbitofrontal cortices were significantly attenuated (Bora et al., 2012b). In elderly patients with MDD, volume deficits can be localized to frontal and subcortical areas, especially in the thalamus (Bora et al., 2012b). In short, neuroimaging changes in neural circuitry have been associated with cognitive dysfunction (including explicit learning, memory, and attention) in MDD and implicate frontal and non-frontal nodes, such as the subgenual anterior cingulate, as well as subcortical brain regions such as the amygdala, striatum, and thalamus.
Circuitry underlying cognitive domains in depression
Recent meta-analytic data (Lee, Hermens, Porter, & Redoblado-Hodge, 2012) highlighted that patients with MDD were found to have slower psychomotor speed, impairments in attention, executive function, verbal fluency, cognitive flexibility, and, to a lesser extent, visual memory. Performance on these complex cognitive tasks requires well-coordinated activity between distributed brain networks (Lee et al., 2012).
Processing speed
Reduced processing speed is one of the most consistent cognitive deficits observed among individuals with MDD (Lee et al., 2012). Processing speed can relate to underlying white matter deficits leading to slowed neurotransmission. In healthy elderly individuals, leukoaraiosis within the periventricular regions is associated with measures of cognitive processing speed. Overall slower processing speed is associated with increased diffusivity in the anterior corpus callosal genu, while slower nonverbal processing speed is associated with increased diffusivity in parietal white matter (Prins et al., 2005; Van den Heuvel et al., 2006).
Turken et al. (2008) investigated white matter deficits underlying cognitive processing speed and found that changes in FA in the left middle frontal gyrus, bilateral parietal lobes, and bilateral temporal lobes in healthy adults were associated with cognitive processing speed. Furthermore, impaired performance in digit symbol coding was associated with decreased FA in the superior (SLF) and inferior longitudinal fasciculi (ILF), occipito-frontal fasciculus, as well as the posterior corona radiata. Voxel-based mapping showed that lesions in left parietal white matter, as well as cortical lesions in the supramarginal and angular gyri, caudate nucleus, and putamen were associated with impaired processing speed. The SLF complex comprises reciprocal projections between association cortices, including posterior parietal and dorsolateral prefrontal cortices. These are regions that are implicated as regions relevant to processing speed and thereby associated with faster performance in lexical decision tasks and choice reaction time tasks (Turken et al., 2008). Furthermore, psychomotor retardation and general cognitive slowing are established features of MDD. Psychomotor features of MDD have been observed across a range of areas including speech, eye movements, gross motor movements, posture, and reaction time. It has been hypothesized that psychomotor retardation may, at least in part, be accounted for by slowed cognitive processing (Caligiuri & Ellwanger, 2000). This is supported by the fact that tasks with low or high cognitive demand are slowed in patients with MDD (Buyukdura, McClintock, & Croarkin, 2011). Clinically, psychomotor retardation is considered a biological symptom and has been associated with increased mean diffusivity in pathways linking bilateral left pre-supplementary motor areas (Bracht et al., 2012). In addition, decreased dopaminergic function, reflected by dopamine-like ligand uptake within the striatum, was associated with greater psychomotor retardation, suggesting affected fronto-striatal and fronto-parietal neural circuits underlying processing speed deficits in MDD (Walther et al., 2012).
Attention
Everyone knows what attention is. It is the taking possession by the mind, in clear and vivid form, of one out of what seem several simultaneously possible objects or trains of thought. Focalization, concentration, of consciousness are of its essence. (William James)
Attention is also understood as the process underlying the allocation of cognitive resources (Posner & Petersen, 1989). It is a complex cognitive domain, intimately involved across cognitive processes, and can be difficult to disambiguate from the context of a specific task. One conceptualization of attention is in terms of alerting, orienting, and conflict processing in the attention task network (Posner & Rothbart, 2007). Each of these processes has been associated with the integrity of specific white matter tracts. Alerting, which is described as the system responsible for activating the necessary cognitive systems to respond to a task, is associated with FA of the posterior limb of the internal capsule. Orienting, involved in selecting specific information, is associated with the FA of the splenium of the corpus callosum, and conflict processing is associated with FA of the anterior corona radiata (Niogi, Mukherjee, Ghajar, & McCandliss, 2010).
Loss of concentration or impaired attention is an observed clinical feature of MDD, whereby patients are often found to be distractable or are not able to sustain attention for prolonged periods of time. Specifically, patients can show impairments on tests, such as the forward digit span and forward spatial span, impaired vigilance, and lack of effort, which may be accounted for by impairment of cognitive control and related to “affective interference” such as rumination and other task-irrelevant thoughts (Fales et al., 2008).
Imaging studies have demonstrated impairments in the prefrontal and anterior cingulate activation, as well as dysregulation of the fronto-striato-cerebellar network, in patients with MDD (Chantiluke et al., 2012; Halari et al., 2009). In children with a history of MDD, reduced resting state functional connectivity between the components of the ventral attention network, comprising the right VLPFC, right posterior superior temporal gyrus, and right ventral supramarginal gyrus, has been observed. Specifically, medication-naïve depressed adolescents have reduced activation in the right DLPFC, inferior prefrontal cortex, and anterior cingulate gyrus in addition to the putamen, insula, temporal and parietal lobes while performing specific tasks (Halari et al., 2009). Additionally, fronto-striato-cerebellar dysregulation was observed in adolescents during tasks of motivated attention, whereby rewarded sustained attention was associated with increased cerebellar activation and reduced fronto-striatal activation in individuals with MDD (Chantiluke et al., 2012).
Executive functioning
A child in his intellectual capacity and manifestations, he has the animal passions of a strong man. Previous to his injury, although untrained in the schools, he possessed a well-balanced mind, and was looked upon by those who knew him as a shrewd, smart businessman, very energetic and persistent in executing all his plans of operation. In this regard his mind was radically changed, so decidedly that his friends and acquaintances said he was “no longer Gage.” (O’Driscoll & Leach, 1998: 1673)
Phineas Gage is the index case from which the connection between the frontal lobes and executive function was first made. The executive system refers to the set of processes that facilitate the adaptation to novel situations and goal-directed action by modulating and controlling more fundamental or routine cognitive skills. In a sense, it directs controlled as opposed to automatic behavior. Executive ability has been traditionally considered synonymous with frontal lobe function. In the case of Phineas Gage, it was less well-known that 11 percent of total white matter volume was lost in his left frontal lobe (Ratiu, Talos, Haker, Lieberman, & Everett, 2004). More recently, a connectivity analysis of white matter involved in the case of Phineas Gage demonstrated that the damage to frontal white matter fasciculi would have caused disruption to several network hubs, suggesting that changes in frontal white matter integrity could account for deficits in executive function (Van Horn et al., 2012).
Data suggest that executive function is sensitive to white matter changes. Tullberg et al. (2004) demonstrated that WMH load whether in the frontal, parietal, or to a lesser extent, occipito-temporal regions is associated with reduced frontal lobe glucose metabolism. WMH load was also associated with worse executive function in non-demented subjects with no relationship to glucose metabolism in any other brain region (Tullberg et al., 2004).
Parallel loops with segregated functions following a rostro-caudal gradient exist within cortical–subcortical circuitry involving the connections between areas of the cortex, striatum, pallidum, and thalamus. This includes the limbic circuitry involving more ventral and medial prefrontal circuits as well as executive functioning circuits involving rostral and dorsal areas. Dorsal striatum co-activates in areas of the associative cortex such as the rostral anterior cingulate, insula, and DLPFC (Alexander, DeLong, & Strick, 1986; Draganski et al., 2008). In a diffusion-tensor imaging (DTI) study of patients with cerebral autosomal dominant arteriopathy, subcortical infarcts, and leukoencephalopathy (CADASIL), it was found that executive function, as measured by performance on various tests such as trail making, digit symbol, and digit span, was significantly correlated with both mean diffusivity and FA in the cingulum bundle, left inferior frontal gyrus, and area adjacent to the left precuneus (O’Sullivan, Barrick, Morris, Clark, & Markus, 2005). In the domain of inhibition, Aron and colleagues were able to define a circuit between the right inferior frontal cortex, pre-supplementary motor area, and the subthalamic nucleus with both diffusion-weighted tractography and functional MRI of a conditional stop-signal paradigm (Aron, Behrens, Smith, Frank, & Poldrack, 2007).
Alexopoulos (2003) proposed that fronto-striato-limbic abnormalities underlie MDD and patients with both MDD and executive dysfunction commonly have WMH within this circuit. In younger patients with MDD, the basal ganglia, prefrontal, and limbic areas demonstrate reduced metabolism when compared with healthy controls (Mayberg et al., 1999). In a study of middle-aged patients with MDD, the Stroop interference time correlated with the number of white matter lesions in frontal, striatal, and insular regions, which correlated strikingly with the “hate circuit” described earlier (Alexopoulos, 2003).
Memory
Psychoanalytic theory contextualizes forgetting and memory impairment in terms of repression, a defense mechanism where the unconscious mind buries painful feelings or thoughts. Subjective memory complaints are common in MDD. Indeed, memory impairment can sometimes be a predominant symptom in MDD where the patient presents with pseudodementia which resembles true dementia in terms of apparent cognitive domains affected, including memory. Memory deficits can be divided into failures of encoding, storage, and retrieval, or in terms of the specific forms of memory that are impaired such as declarative or non-declarative memory. Encoding, for example, could be affected by rumination, while retrieval could be deficient when executive function is also impaired.
WMH in the subcortical area are negatively associated with both memory and executive functioning in the healthy elderly (Van Petten et al., 2004). In particular, visual paired associated, long-delay cued recall in the California Verbal Learning Test and logical memory were negatively correlated with total number of WMH lesions and overall memory was negatively associated with a number of patchy and punctate lesions. In addition, memory was also related to inferior temporal and middle prefrontal gray matter volumes (Van Petten et al., 2004). A voxel-based lesion mapping analysis of declarative memory in multiple sclerosis found that white matter lesions in the temporal lobe lateral to the hippocampus and the anterior temporal stem as well as the thalamic region and anterior limb of the internal capsule were associated with impairments in memory storage and retrieval. Lesions of the temporo-parieto-frontal paramedian bundles and fronto-occipital fasciculi were specifically associated with impairments of retrieval (Sepulcre et al., 2008).
Minett and colleagues investigated the presence of memory complaints in elderly individuals with MDD. They found that greater subjective memory impairment was present in those with more severe symptoms of MDD and an increased proportion of brain white matter lesions (Minett, Dean, Firbank, English, & O’Brien, 2005). This was consistent with a study conducted by Murata et al. (2001) in which patients with moderate deep white matter lesions had more pronounced cognitive impairment and clinician-rated depressive symptoms than those with mild lesions. Subjective memory complaints can be attributed to a number of causes, including objective memory deficits as well as a failure in meta-cognition (Ponds & Jolles, 1996). Meta-memory refers to the ability to accurately detect or predict memory processes and performance. Given that MDD is associated with negative biases, a plausible hypothesis would be that these complaints partly reflect a distortion in meta-memory. Meta-memory appears to be subserved by medial networks of the anterior prefrontal cortex involving the medial prefrontal cortex, the central precuneus, and the intraparietal sulcus/inferior parietal lobule (Baird, Smallwood, Gorgolewski, & Margulies, 2013; Modirrousta & Fellows, 2008). The involved brain regions suggested a fronto-parietal network that is consistently dysregulated in MDD which provides a potential mechanism for subjective memory complaints seen in MDD.
Circuitry underlying cognitive biases in depression
Beck first proposed a cognitive model for depression over four decades ago that has formed the basis for cognitive therapy. His model is based on schemas, which are internal representations of stimuli, ideas, or experiences that influence the acquisition and processing of information. These schemas can lead to biases in attention, interpretation, and memory, which in turn may result in negative thoughts about self, the world around, and the future. The neural correlates of this model are reviewed in a recent paper by Disner et al. (Disner, Beevers, Haigh, & Beck, 2011).
Attentional bias
Individuals with clinical MDD have an attentional bias for sad stimuli (MacLeod, Mathews, & Tata, 1986). Neuroimaging studies have demonstrated involvement of the ACC, DLPFC, VLPFC, and superior parietal cortex (SPC) (Surguladze et al., 2005). Specific roles have been suggested for different brain regions in that VLPFC is important in selecting gaze targets, the DLPFC and ACC inhibit the VLPFC to allow disengagement of the target, and the SPC coordinates the shift in gaze. It was proposed that reduced activity involving aforementioned brain regions leads to difficulty in disengaging from negative stimuli (Disner et al., 2011). This may be partly mediated by deficient inhibition by the rostral ACC as healthy individuals showed greater rostral ACC activity when successfully inhibiting attention, regarding positive stimuli, while those with MDD displayed greater activation when diverting attention from negative stimuli (Eugène, Joormann, Cooney, Atlas, & Gotlib, 2010).
Biased processing of emotional stimuli
In addition to biases in the allocation of attention to negative stimuli, individuals with MDD also appear to focus on the negative aspects of any given stimuli. This extends beyond the saliency of negative stimuli and involves changes in higher-level processing and concerns emotional tone or meaning in the interpretation of perceptions.
Emotional stimuli, as with other stimuli, are relayed to the thalamus, which projects to the amygdala. The amygdala is activated during processing and detection of emotional stimuli and has an inverse relationship with the left DLPFC activation. Individuals with MDD have increased amygdala reactivity that persists after emotional stimuli are removed. Increased amygdala reactivity is also associated with faster processing of negative stimuli which is attenuated by antidepressants (Elliott, Rubinsztein, Sahakian, & Dolan, 2002).
The thalamus is responsible for the distribution of afferent signals downstream to the ventral and dorsal cingulate cortex. This may determine the balance between hot and cold cognitive processes. Individuals with MDD show increased thalamic activity and decreased functional connectivity between the dorsal ACC and medial thalamus and compensatory increased activity of the thalamus during processing of emotional stimuli (Greicius et al., 2007). This may then lead to less inhibition of the limbic circuitry and increased emotional processing of stimuli in MDD.
In MDD, there is also impaired processing of positive stimuli and patients have impaired brain responses to reward. Subjects with MDD displayed hyperactivation in the presence of negative stimuli and hypoactivation for positive stimuli in the amygdala, striatum, parahippocampal region, fusiform, and ACC (Russo & Nestler, 2013). These regions constitute the limbic and reward networks involved in bottom-up emotional processing and may reflect overall bias for negative valence. The amygdala is thought to process both positive and negative emotions in the brain and is ethologically considered to respond to features of relevance for the organism. The striatum receives input from and interacts with the amygdala, and is part of the reward circuitry involved in emotion regulation.
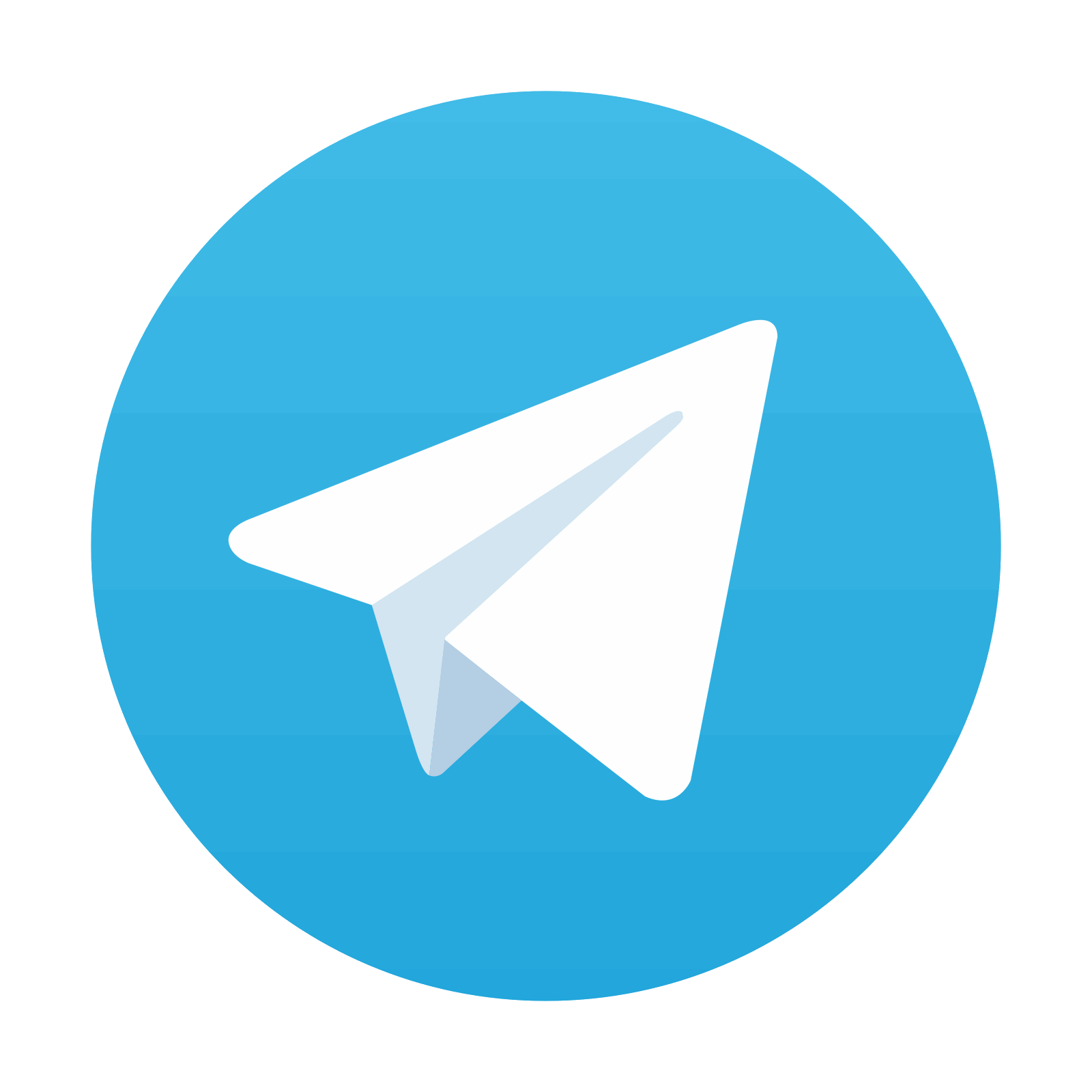
Stay updated, free articles. Join our Telegram channel

Full access? Get Clinical Tree
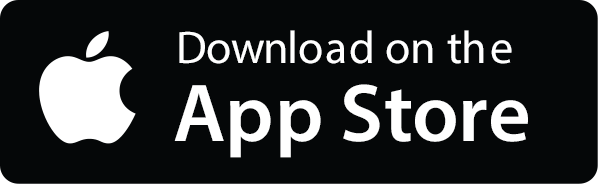
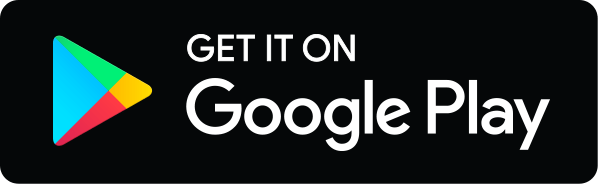