div class=”ChapterContextInformation”>
13. Neurostimulation: Why, When, and Which One?
Neuromodulation has been proposed for more than a decade to treat primary headaches including cluster headache. Neuromodulation can be separated into invasive techniques, that is, with a surgical procedure to implant the stimulation device, and noninvasive techniques (transcutaneous or transcranial stimulation). For the treatment of cluster headache (CH), the only noninvasive neuromodulation technique studied up to now is vagus nerve stimulation (cervical portion), while invasive neuromodulation has been applied to target the posteroinferior hypothalamic area, the great occipital nerve, or the sphenopalatine ganglion. For each target, we will review key elements in terms of background, efficacy evidence, limits, and mechanisms of action.
13.1 Vagus Nerve Stimulation
13.1.1 Background
Vagus nerve stimulation has been considered as a promising treatment of primary headaches following migraine improvement in epileptic patients with a migraine comorbidity, while their epilepsy was treated by implanted vagus nerve stimulation [1]. Recent devices allowing a noninvasive stimulation of the vagus nerve (nVNS) have increased interest for this target, the gammaCore® device having been meanwhile specifically developed for the treatment of headache by noninvasive stimulation of the cervical branch of the vagus nerve.
13.1.2 Evidence
PREVA study is an open randomized controlled trial (RCT) in which nVNS was examined as adjunctive prophylactic treatment of chronic CH [2]. The PREVA study compared adjunctive prophylactic nVNS (n = 48) with standard of care (SoC), i.e., medications alone as a control (n = 49). A 2-week baseline phase was followed by a 4-week randomized phase (SoC plus nVNS vs. SoC alone) and a 4-week extension phase (SoC plus nVNS). The primary endpoint was the reduction in the mean number of CH attacks per week. During the randomized phase, individuals in intent-to-treat population treated with SoC plus nVNS (n = 45) had a significantly greater reduction in the number of attacks per week compared to those receiving SoC alone (n = 48) (−5.9 vs. −2.1, respectively) for a mean therapeutic gain of 3.9 fewer attacks per week (95% CI: 0.5–7.2; p = 0.02). This preventive effect was maintained during the 4-week extension phase during which all patients benefited from nVNS [3]. Using PREVA study data, a pharmacoeconomic model from the German statutory health insurance perspective showed cost-effectiveness of nVNS, suggesting that adjunctive nVNS provides economic benefits in the treatment of chronic CH [4].
The PREVA study did not show any evidence of nVNS efficacy for the acute treatment of CH in patients with chronic CH [5]. Conversely, nVNS showed its efficacy to abort or relieve attacks of episodic CH in two large sham-controlled trials (ACT1 and ACT2, ref.). ACT2 study is a RCT that compared nVNS with a sham (placebo) device for acute treatment in patients suffering from episodic or chronic CH [6]. After completing a 1-week run-in period, subjects were randomly assigned to receive nVNS or sham stimulation during a 2-week double-blind period. The primary efficacy endpoint was the proportion of all treated attacks that achieved pain-free status within 15 min after treatment initiation, without rescue medication. The Full Analysis Set comprised 48 nVNS-treated (14 episodic CH, 34 chronic CH) and 44 sham-treated patients (13 episodic CH, 31 chronic CH). From the primary endpoint, nVNS (14%) and sham (12%) treatments were not significantly different for the entire CH population. No significant differences were seen between nVNS (5%) and sham (13%) in the chronic CH subgroup. By contrast, nVNS (48%) was superior to sham (6%; p < 0.01) in the episodic CH subgroup. Efficacy of nVNS for the acute treatment of episodic CH was also supported by the ACT1 study [7]. ACT1 study is a RCT similar to ACT2, but the primary endpoint was the response rate, defined as the proportion of subjects who achieved pain relief at 15 min after treatment initiation for the first attack without any rescue medication use through 60 min. The intent-to-treat population comprised 133 subjects: 60 nVNS-treated (episodic CH, n = 38; chronic CH, n = 22) and 73 sham-treated (episodic CH, n = 47; chronic CH, n = 26). Again, response rates were overall not significantly different between nVNS-treated and sham-treated patients (26.7% vs. 15.1% p = 0.1), but were significantly higher with nVNS than with sham when the episodic CH subgroup was considered (34.2% vs. 10.6%; p = 0.008).
13.1.3 Limits
Evidence supports the use of nVNS as an acute treatment of episodic CH and as a prophylactic treatment of chronic CH. Nevertheless, based on clinical experience, therapeutic benefit from prophylactic treatment would be more convincing than from acute treatment especially in chronic CH [8]. Acute nVNS use requires the self-application of three stimulation sessions of 2 min each separated by 1 min from the beginning of the attack. For preventive use, the administration of a stimulation period of 2 min three times a day is necessary and must be evaluated over 3 months. The gammaCore® device has only one nVNS program. The subject can use the device on the right or left sides of the neck by putting it next to his/her carotid pulse (usually alternate sessions are recommended). Intensity is raised until the subject feels a tingling sensation deep in the neck, and the device is well positioned when the subject feels a tightness of its lower lip (due to platysma muscle contraction). Safety and tolerability of nVNS with gammaCore® was confirmed by the three RCTs (PREVA, ACT1, ACT2) performed in CH. In these trials, the side effects (voice change, skin irritation, muscle contraction, dysesthesia) were mild to moderate and all transient [2, 6, 7]. The manufacturer of gammaCore® advises not to use it in pregnancy and in the following situations: cervical atheroma, implanted stimulator, high blood pressure, hypotension, tachycardia, bradycardia, cervical vagotomy, and metallic device implanted in the cephalic segment. This device has a CE mark, but it is not reimbursed by all health insurance systems. It is available, on prescription, on the manufacturer’s website (https://gammacore.com) at a rate of 260 €. Although comparable to the triptan budget, this price might therefore represent a limit to nVNS use, especially as this device allows a limited number of stimulations (or “doses,” up to 300) but its battery cannot be recharged. Thus, a new device must be purchased at the end of the battery.
13.1.4 Mechanism of Action
The precise mechanism of action of nVNS in primary headaches is not known, but corpus of data is available and allows certain assumptions [9]. The reality of vagus nerve stimulation by gammaCore® has been confirmed using a neurophysiological approach in healthy volunteers, which showed that cervical nVNS induced evoked nerve potentials similar to those induced by invasive vagus nerve stimulation devices [10]. Similarly, a functional magnetic resonance imaging study, also performed in healthy controls stimulated by gammaCore®, highlighted an activation of the solitary tract nucleus, which is the main central relay of vagal afferences [11]. The therapeutic effect of nVNS is probably mediated by the stimulation of large myelinated fibers as argued by magnetic resonance-based model predicting the properties of the induced electric field in different anatomical planes [12]. The lack of C fibers recruitment suggested by this model accounts for the absence of pain and parasympathetic signs with nVNS using gammaCore®. Experimental works have also tried to specify the mechanism of the therapeutic effect of gammaCore® in primary headaches. Centered on migraine, a first experimental work has shown an inhibition of cortical spreading depression (CSD) support of the migraine aura and possible trigger of migraine headache [13]. Another study, focused on trigeminal pain and performed on a murine model of trigeminal allodynia induced by dural inflammation, has shown a significant reduction in periorbital skin sensitivity for more than 3.5 h after nVNS, this reduction being associated with a reduction of extracellular glutamate concentration in the trigemino-cervical complex [14]. A neuroimaging study showed an activation of the solitary tract nucleus that was associated to changes in the pain matrix (parabrachial nucleus, primary somatosensory cortex, and the insula) and the trigemino-cervical complex [11]. Finally, an experimental electrophysiological work demonstrated the ability of implanted vagus nerve stimulation to reduce dose-dependent nociceptive activation of neurons of the trigemino-cervical complex and the superior salivary nucleus which are the two essential relays of the trigemino-autonomic pathway supporting primary headaches like migraine and cluster headache [15].
13.2 Deep Brain Stimulation of Posteroinferior Hypothalamic Area
13.2.1 Background
Deep brain stimulation (DBS) of the posteroinferior hypothalamus has been the first neuromodulation technique to be proposed in drug-refractory chronic CH. The initial concept was to inhibit the presumed CH attack generator [16, 17] identified in this area shortly before, by neuroimaging studies. Indeed, positron emission tomography (PET) imaging during CH attacks showed a specific activation of an area located at the diencephalo-mesencephalic region, close to the floor of the third ventricle [18]. Based on its projection on the Talairach grid, this region has been called posteroinferior hypothalamus.
13.2.2 Evidence
Open series and RCT related to DBS in chronic CH
Study | Patients (n) | Country | Mono/multi centric | Mean follow-up (years) | At least 50% improvement (n) |
---|---|---|---|---|---|
17 | Italy | Mono | 8.7 | 12 | |
Schoenen et al. [25] | 6 | Belgium | Mono | 4 | 3 |
Starr et al. [27] | 4 | USA | Mono | 1 | 2 |
Owen et al. [24] | 1 | GB | Mono | 0.7 | 1 |
Bartsch et al. [20] | 6 | Germany | Mono | 1.4 | 3 |
Fontaine et al. [22] (RCT) | 11 | France | Multi | 1 | 6 |
Seijo et al. [26] | 5 | Spain | Mono | 2.8 | 5 |
Akram et al. [19] | 21 | GB | Mono | 1.5 | 11 |
Chabardès et al. [21] | 7 | France | Mono | 1 | 6 |
Total | 78 | 49 (62.8%) |
13.2.3 Limits
DBS is the last-line preventive treatment of the most severe chronic CH patients and should only be practiced by medico-surgical teams combining headache expertise and functional neurosurgery expertise with a strict respect of patient selection criteria (at least 2 years of disease duration, at least one attack per day, resistance to pharmacotherapy including verapamil and lithium, headache “locked” to the same side, normal neurological examination, and absence of psychiatric comorbidity) [28, 29]. This position as a last-line treatment is justified by the invasiveness and the risks of this therapeutic approach. If few side effects are related to the stimulation itself (essentially gaze disturbances), the implantation of the electrode can be associated to brain hemorrhages which can be fatal [25]. This risk can be reduced by endoventricular stimulation of the hypothalamus using a floating DBS electrode laid on the floor of the third ventricle [21].
13.2.4 Mechanisms of Action
The common target used for posteroinferior hypothalamic DBS is located 5 mm below the mid-commissural point (MCP), 2 mm lateral to the midline, and 3 mm posterior to the MCP [16], although stimulation delivered from an electrode located on the floor of the third ventricle is also effective [21]. The neural structure corresponding to these coordinates and whose stimulation induces the therapeutic effect is still debated. Fontaine and colleagues studied the anatomical locations of the DBS electrodes and identified several candidates [30], including the mesencephalic gray substance, the ventral tegmental area, and several tracts connecting the hypothalamus with autonomic nuclei of the brain stem. Recently, a more precise modeling of volume of cerebral tissue activated by DBS in responders and non-responders was used to identify the region associated with the highest improvement [19]. The spot that correlated with better outcome was located 6 mm lateral, 2 mm posterior, and 1 mm inferior to MCP, in an area between the red nucleus and the mammillothalamic tract, encompassing the ventral tegmental area and mesencephalic gray and the lateral wall of the floor of the third ventricle (explaining the efficacy of DBS lead implanted in the V3). An additional tractography study showed that this area was crossed by a so-called trigemino-hypothalamic tract, connecting the trigeminal system (and other brain stem nuclei associated with nociception and pain modulation) with the hypothalamus, the prefrontal, and the mesio-temporal area. However, as the electrodes’ coordinates are usually similar in DBS responders and non-responders, failure of DBS in CH may be caused by factors other than electrode misplacement, likely related to the disease itself.
Very few neuroimaging studies have explored brain activity changes following retro-hypothalamic DBS. May et al. studied the acute (60 s) effects of DBS by positron emission tomography. They reported cerebral blood flow changes induced by stimulation in the ipsilateral posterior hypothalamic gray (site of electrode implantation), the ipsilateral thalamus, the somatosensory cortex and precuneus, the anterior cingulate cortex, and the ipsilateral trigeminal nucleus and ganglion [31]. A magnetoencephalography study in a single patient reported short-term (10 min) retro-hypothalamic DBS-induced activity changes in the orbitofrontal cortices and in the periaqueductal gray [32]. No study explored long-term effect of DBS in chronic CH patients. Together, these data suggest two alleged mechanisms of action for DBS in CCH. First is the inhibition of a CH generator located in the hypothalamus via stimulation of afferent fibers located in the retro-hypothalamic area. This mechanism might be specific to CH. Second is the modulation of non-specific antinociceptive systems, including the mesencephalic gray substance, and the orexinergic system [33] leading to modulation of regions belonging to the “pain matrix.”
13.3 Occipital Nerve Stimulation
13.3.1 Background
Occipital nerve stimulation (ONS) is characterized by the application of a continuous electrical stimulation over the great and/or lesser occipital nerves (respectively, GON and LON), using a subcutaneous chronically implanted electrode that is placed close to the nerve and connected to a battery. This procedure was originally described by Weiner and Reed [34] and has been first proposed to treat occipital neuralgia and then primary headaches, including CH.
13.3.2 Evidence
Open series related to ONS in chronic CH
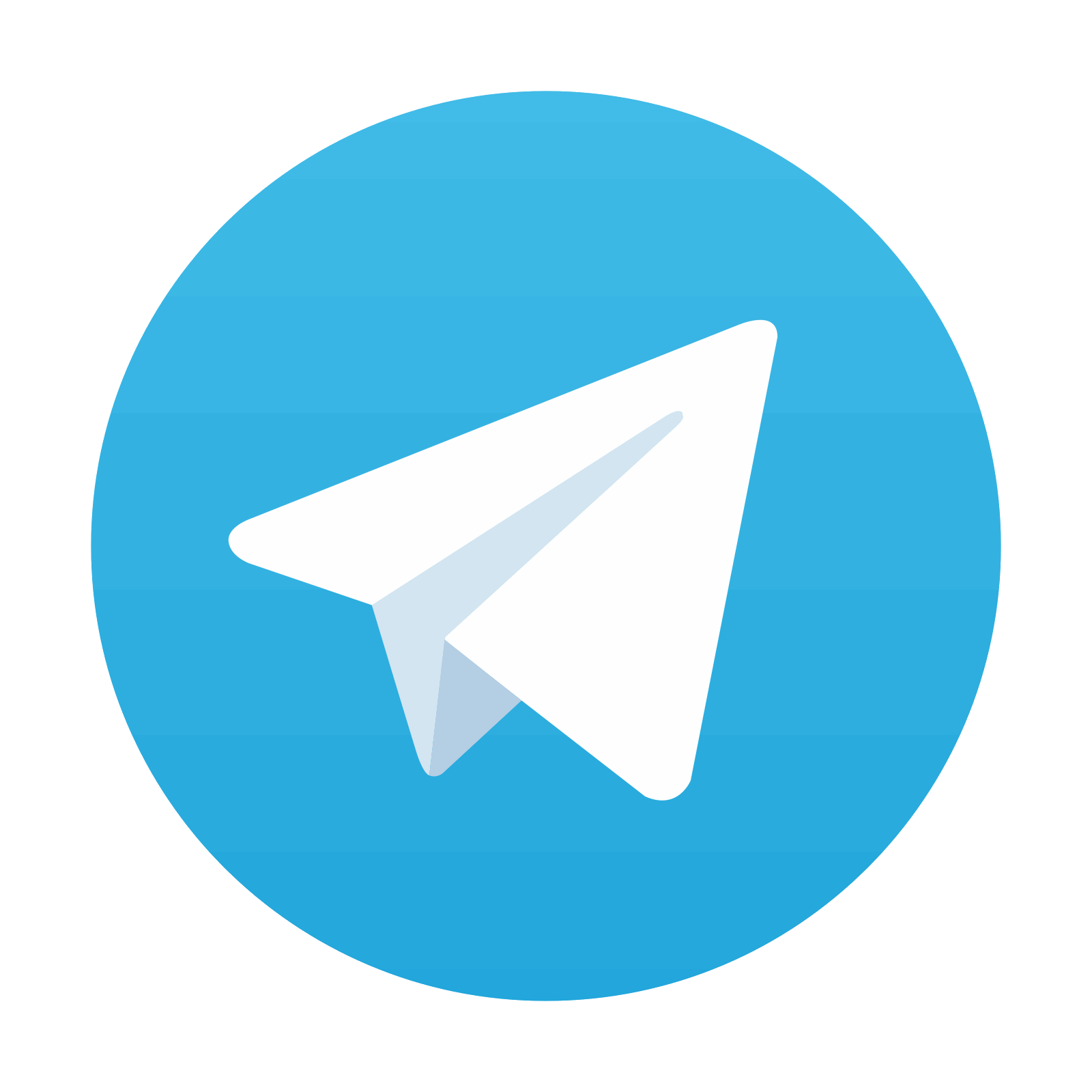
Stay updated, free articles. Join our Telegram channel

Full access? Get Clinical Tree
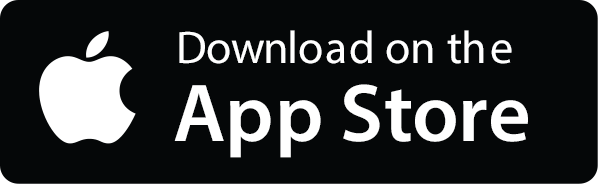
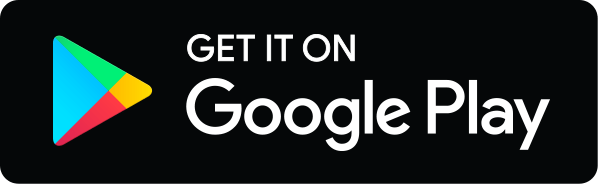
