FIGURE 43.1 A. Ventral and (B) dorsal views of the human cerebellum. See Figure 43.4 for names of lobes and lobules.
FIGURE 43.2 Median longitudinal section through the human cerebellum, pons, and medulla.
FIGURE 43.3 Horizontal section through the human cerebellum showing the arrangement of the cortical gray matter and locations of the nuclei within the white matter.
Gross Anatomy
Anatomically, the cerebellum is divided into three lobes: anterior, posterior, and FN. Each has a vermis and hemisphere portion (Figure 43.3). The deep transverse primary fissure divides the cerebellum into anterior and posterior lobes. The posterolateral fissure separates the FN lobe from the posterior lobe. Anatomists further divide the cerebellum by fissures and sulci into 10 lobules that bear arcane names of no clinical relevance (Figure 43.4). In terms of afferent and efferent connections, the cerebellum can also be organized into three parallel, sagittal zones: vermian, paravermian, and lateral. As mentioned previously, clinicians divide the cerebellum functionally into three parts: (a) the hemispheres, responsible for appendicular coordination; (b) the anterior, superior vermis (or simply the vermis), responsible for gait and other axial functions; and (c) the FN lobe, or vestibulocer-ebellum. The FN lobe is phylogenetically the oldest and is referred to as the archicerebellum. The FN lobe has extensive connections with the vestibular nuclei and is concerned primarily with eye movements and gross balance. It also receives visual afferents from the superior colliculi and the visual cortex. The primary concerns of the archicerebellum are eye movement control and gross orientation in space, such as up and down. The next area of the cerebellum to evolve was the paleocerebellum or spinocerebellum. In humans, the paleocerebellum consists of the anterior, superior vermis and adjacent paravermal cortex; it corresponds approximately to the anatomical anterior lobe. The paleocerebellum developed during a period of evolution when extremity control was not a concern; it is concerned primarily with posture, muscle tone, axial muscle control, and locomotion. There are extensive connections between the vermis and spinal cord pathways. The most phylogenetically recent part of the cerebellum is the neocerebellum, or the cerebellar hemispheres, which make up the bulk of the cerebellum. The neocerebellum corresponds approximately to the posterior lobe. The hemispheres are concerned with coordinating movement and providing fine motor control for precise movements of the extremities. The primary afferents to the hemispheres are from the pontine nuclei, which receive the corticopontine fibers from the cerebral cortex. Another way to view the cerebellum is in terms of its primary afferent connections: the vestibulocerebellum (input from vestibular nuclei to the FN lobe), the spinocerebellum (input from the spinocerebellar tracts to the anterior vermis), and the pontocerebellum (input from the pontine nuclei to the hemispheres).
FIGURE 43.4 Diagram of the cerebellum showing the lobes and lobules.
The cerebellum is made up of a white matter core, covered with a thin layer of gray matter, the cerebellar cortex. Deep in the white matter are several gray masses, the cerebellar nuclei. The dentate nuclei, the largest of the cerebellar nuclei, are gray matter structures situated deep in the white matter of each hemisphere (Figure 43.3). In the hilus of each dentate nucleus lie the emboliform nuclei; medial to the emboliform are the globose nuclei. The globose and emboliform nuclei together are called the nucleus interpositus. In the white matter of the vermis, at the roof of the fourth ventricle, are the fastigial, or roof, nuclei. From medial to lateral, the deep nuclei are the fastigial, globose, emboliform, and dentate. The major cerebellar connections are to the vestibular system, the spinal cord, and the cerebral cortex (Figure 43.5). Microscopically, the cortex is made up of three layers: the outer, nuclear, or molecular layer; the layer of Purkinje cells; and the inner, or granular, layer (Figure 43.6).
FIGURE 43.5 Principal afferent and efferent connections of the cerebellum.
FIGURE 43.6 Transverse section of cerebellar folia showing the three layers of the cortex and the underlying white matter (cresyl violet], (Reprinted from Kiernan JA, Barr’s the Human Nervous System: An Anatomical Viewpoint. 9th ed, Philadelphia: Wolters Kluwer/Lippincott Williams & Wilkins, 2009, with permission.]
The FN lobe is a primitive part of the cerebellum primarily concerned with vestibular function. The connections of the FN lobe are primarily, if not entirely, vestibular. The FN lobe receives afferent impulses from the labyrinths and vestibular centers, spinal cord, and brainstem—including the reticular formation and olivary bodies—and projects to the vestibular nuclei, vestibulospinal tracts, and reticular formation. The cerebellum and vestibular centers function together to maintain equilibrium, the orientation of the body in space, and the regulation of muscle tone and posture. The clinical manifestations of disease of the FN lobe are difficult to separate from the invariably accompanying vestibular findings, primarily nystagmus. Isolated FN lobe dysfunction is usually caused by ependymomas and medulloblastomas in children.
The paleocerebellum communicates with the spinal cord, brainstem, and vestibular centers. The principal afferent connections of the anterior lobe come from the anterior spinocerebellar tract, although it receives trigeminocerebellar fibers, input from the vestibular nuclei, and some corticocerebellar fibers. The discharges are to the vestibular nuclei, brainstem, and spinal cord.
The neocerebellum (pontocerebellum) communicates with the cerebral cortex. It is enormously developed in mammals in association with growth of the cerebral hemispheres. In primates, the hemispheres overshadow the rest of the cerebellum. Its afferent connections are principally corticopontine, or corticopontocerebellar, although there are some spinocerebellar fibers; it discharges through the dentate nucleus to the red nucleus and thalamus, and thus to the cerebral cortex.
The cerebellum is connected to the brainstem by the three cerebellar peduncles. The inferior cerebellar peduncle (ICP) connects the cerebellum with the spinal cord and the medulla. The ICP lies medial to the middle cerebellar peduncle (MCP, brachium pontis). The ICP has two parts: the restiform body and the juxtarestiform body. Ascending fibers in the restiform body include the posterior spinocerebellar and cuneocerebellar (from the accessory cuneate nucleus) tracts, the dorsal and ventral external arcuate fibers from the nuclei gracilis and cuneatus, and the olivocerebellar, trigeminocerebellar, and reticulocerebellar pathways. Lying just medial to ICP is the juxtarestiform body, made up of fibers traveling directly between the vestibular nuclei and the FN lobe. The restiform body is an afferent system; the juxtarestiform body contains both vestibulocerebellar and cerebellovestibular fibers. The juxtarestiform body is mainly efferent. Although it carries primary afferent vestibulocerebellar fibers from the vestibular nerve and secondary vestibulocerebellar fibers from the vestibular nuclei, its primary component is cerebellovestibular fibers from the vermis and the FN lobe (fastigiobulbar tract). Other cerebellovestibular fibers run from the fastigial nucleus to the vestibular nuclei in the uncinate fasciculus, which enters the brainstem adjacent to the ICP. The MCP connects the cerebellum with the pons, and through it run the pontocerebellar tracts; these are the final neurons of the corticopontocerebellar pathway that comes mainly from the frontal, temporal, and other areas of the cortex to communicate with the contralateral cerebellar hemisphere. The superior cerebellar peduncle (SCP, brachium conjunctivum) contains the principal efferent fibers of the cerebellum and the dentatorubral and the dentatothalamic pathways. It also carries the afferent anterior spinocerebellar tract, as well as cerebellovestibular fibers in the uncinate fasciculus (hook bundle of Russell, fastigiovestibular or fastigiobulbar tract). The cerebellotegmental, cerebellotectal, and tectocerebellar tracts also travel in the SCP. The afferent fibers to the cerebellar cortex arrive primarily by tracts that enter through the middle and inferior peduncles, but the anterior spinocerebellar tract enters via the SCP.
Microscopic Anatomy
The molecular layer of the cerebellar cortex contains the dendritic arborizations of the Purkinje cells, radial fibers of the Bergmann glial cells, basket cells, stellate cells, climbing fibers, and parallel fibers (Figure 43.6). The flattened dendritic trees of the Purkinje cells spread out perpendicular to the long axis of the cerebellar folium. Climbing fibers are the terminal ramifications of fibers from the inferior olivary nucleus that ascend through the granular layer to contact Purkinje dendrites in the molecular layer. Each climbing fiber forms an excitatory synapse with a single Purkinje cell. Climbing fibers also synapse on the neurons of the deep cerebellar nuclei. Parallel fibers are granule cell axons that extend upward into the molecular layer where they bifurcate to send branches in opposite directions along the axis of a folium to terminate on the Purkinje cell dendrites. The parallel fibers intersect the Purkinje cell dendrites like telephone wires over the cross pieces of a telephone pole. The Purkinje cell layer contains the perikarya of the large Purkinje cells and the smaller Bergmann (epithelial) glial cells. The granule cell layer lies between the white matter and the Purkinje cell layer; it contains granule cells, Golgi cells, brush cells, and the cerebellar glomeruli (Figure 43.7). The granule cells send their axons to the molecular layer where they branch to form parallel fibers. Mossy fibers are the predominant afferent system to the cerebellum. They arise from the spinal cord, the trigeminal, reticular formation, vestibular and pontine nuclei of the brainstem. The mossy fibers terminate as mossy fiber rosettes that lie at the center of each cerebellar glomerulus. Like the climbing fibers, mossy fibers are excitatory. The mossy fibers primarily use glutamate; the climbing fibers release glutamate or aspartate. Cerebellar glomeruli are synaptic formations with mossy fiber rosettes at the center, surrounded by granule and Golgi cell dendrites.
FIGURE 43.7 Neurons in the cerebellar cortex showing excitatory and inhibitory synapses, The diagram represents a longitudinally sectioned folium, with an edge-on view of the dendritic tree of the Purkinje cell, Glutaminergic (excitatory] neurons are red; GABA-ergic (inhibitory] neurons are blue. (Reprinted from Kiernan JA, Barr’s the Human Nervous System: An Anatomical Viewpoint. 9th ed, Philadelphia: Wolters Kluwer/Lippincott Williams & Wilkins, 2009, with permission,)
The Purkinje cells are excited by the parallel and climbing fibers, and send inhibitory GABA-ergic projections to the deep cerebellar and the vestibular nuclei. Input from the basket and stellate cells inhibit the Purkinje cells. The granule cells are excitatory, glutaminergic neurons. The parallel fibers that arise from the granule cells excite the Purkinje, basket, stellate, and Golgi cells. The granule cell is excited by mossy fiber input at the cerebellar glomerulus and inhibited by Golgi cells. The mossy fiber input excites Purkinje cells indirectly through the granule cell-parallel fiber system and produces simple spikes from the Purkinje cell. The climbing fibers are entwined around the Purkinje cell, like a vine around a tree trunk, and excite it directly, producing complex spikes. The cerebellar cortex also receives noradrenergic fibers from the locus ceruleus, dopaminergic fibers from the substantia nigra, and serotonergic fibers from the raphe nuclei. All the aminergic afferents are probably inhibitory. Afferents to the cerebellar cortex and deep nuclei generally produce an increase in excitability. The Purkinje cells impose inhibitory control over the cells of the deep nuclei. Mossy fiber input causes strong direct excitation of the deep nuclei; the additional input via the granule cell-parallel fiber system provides inhibitory control and modulation of the direct excitatory pathway. The climbing fibers modulate the activity of the Purkinje cells by controlling the influence of the different systems that converge on it.
The efferent fibers from the Purkinje cells in the cerebellar cortex are nearly all relayed to the deep nuclei, where the cerebellar outflow originates (Figure 43.5). The output from the deep cerebellar nuclei is excitatory and glutaminergic except for the projection to the inferior olive, which uses gamma-aminobutyric acid. The fastigial nucleus, the oldest of the cerebellar nuclei, receives afferent fibers from the paleocerebellum and also from the vestibular nuclei and the eighth cranial nerve. Its efferent impulses, many of them crossing in the roof, pass into the brainstem to the vestibular nuclei—especially the lateral vestibular nucleus—and to the reticular formation. Some of these go through the ICP; others travel in the SCP in the uncinate fasciculus. The fastigial nucleus also projects to the ventral lateral (VL) nucleus of the thalamus, which in turn projects to the trunk area of the motor strip. The interposed nuclei receive afferents primarily from the paravermal cortex and project to VL and to the magnocellular part of the contralateral red nucleus, which gives rise to the rubrospinal tract. The dentate nuclei, the most important of the nuclear masses in terms of clinical function, receive afferents principally from the Purkinje cells of the neocerebellum. The dentate projects to the ipsilateral VL and intralaminar thalamic nuclei and to the contralateral red nucleus and inferior olivary nucleus.
The cerebellum is part of complex feedback loops that are involved in the coordination of motor activity (Figures 43.8 to 43.10). Large myelinated muscle spindle and Golgi tendon organ afferents travel to the cerebellum via the spinocerebellar tracts and enter primarily through the ICP. This information is processed in the hemispheres and influences the activity of Purkinje cells in the deep, midline (primarily dentate) nucleus. The Purkinje cells send axons via the SCP to the contralateral VL nucleus of the thalamus, which in turn projects to the motor cortex. Descending corticopontine fibers then synapse on pontine nuclei in the basis pontis, which in turn send pontocerebellar axons via the MCP to the cerebellar hemispheres. Other descending corticomotor fibers actually execute the task at hand. The cerebellum can thereby communicate the need for fine adjustment of movement to the cortex, and the cortex can take corrective action while simultaneously informing the cerebellum of the extent of the correction so further adjustments can be made (see Chapter 22). The motor thalamus serves to integrate cerebellar, basal ganglia, and cortical activity. Another loop consists of fibers that arise from the inferior olive and travel via the ICP to the dentate nucleus, with projections from the dentate going to the red nucleus, which then projects to the inferior olive (Guillain-Mollaret triangle, see Chapter 30). The direct vestibulocerebellar and returning cerebellovestibular fibers form yet another circuit. The cerebellum also receives input from the hypothalamus.
FIGURE 43.8 Ultrastructure of a synaptic glomerulus in the granule cell layer, The astrocytic processes (yellow) prevent diffusion of neurotransmitters to adjacent synapses, (Reprinted from Kiernan JA, Barr’s the Human Nervous System: An Anatomical Viewpoint. 9th ed, Philadelphia: Wolters Kluwer/Lippincott Williams & Wilkins, 2009, with permission,]
FIGURE 43.9 Connections of the vestibulocerebellum and vestibular nuclei, Afferents to the cerebellum are blue, cerebellar efferents are red, and other neurons are black. (Reprinted from Kiernan JA, Barr’s the Human Nervous System: An Anatomical Viewpoint. 9th ed, Philadelphia: Wolters Kluwer/Lippincott Williams & Wilkins, 2009, with permission.)
FIGURE 43.10 Connections of the spinocerebellum, Afferents to the cerebellum are blue, cerebellar efferents are red, and other neurons are black, (Reprinted from Kiernan JA, Barr’s the Human Nervous System: An Anatomical Viewpoint. 9th ed. Philadelphia: Wolters Kluwer/Lippincott Williams & Wilkins, 2009, with permission.)
CLINICAL MANIFESTATIONS OF CEREBELLAR DYSFUNCTION
Patients with cerebellar dysfunction suffer from various combinations of tremor, incoordination, difficulty walking, dysarthria, and nystagmus, depending on the parts ofthe cerebellum involved (see Table 22.1). Ataxia is the cardinal sign of cerebellar disease; it consists of varying degrees of dyssynergia, dysmetria, lack of agonist-antagonist coordination, and tremor. Ataxia may affect the limbs, the trunk, or the gait. Cerebellar disease may also cause hypotonia, asthenia or slowness of movement, and deviation or drift of the outstretched limbs. Disease involving the cerebellar connections in the brainstem causes abnormalities indistinguishable from disease of the cerebellum itself. When cerebellar ataxia results from dysfunction of the cerebellar connections in the brainstem, there are usually other brainstem signs.
Dyssynergia
The essential disturbance in cerebellar disease is dyssynergia. Normally, there is harmonious, coordinated action between the various muscles involved in a movement so that they contract with the proper force, timing, and sequence of activation to carry out the movement smoothly and accurately. Cerebellar disease impairs the normal control mechanisms that organize and regulate the contractions of the different participating muscles and muscle groups to insure smooth, properly coordinated movement. There is a lack of speed and skill in performing movements that require the coordinated activity of several groups of muscles or of several movements. The cerebellum is instrumental in timing the activation of the different muscles involved in a movement. Lack of integration of the components of the act results in decomposition of movement—the act is broken down into its component parts and carried out in a jerky, erratic, awkward, disorganized manner. The cerebellum is particularly important in coordinating multijoint movements.
Dysmetria
Dysmetria refers to errors in judging distance and gauging the distance, speed, power, and direction of movement. Cerebellar dysfunction leads to loss of the normal collaboration between agonist and antagonist. When reaching for an object 50 cm away, the hand shoots out 55 cm, overshooting the target (hypermetria), or fails to reach the target (hypometria). Hypermetria is more common. The movement may be carried out too slowly or too rapidly with too much or too little force. The patient with dysmetria does not make a movement along a straight line between two points, but erratically deviates from the intended track. Electromyographic studies have shown that dysmetria is associated with abnormalities of the timing and force of antagonist contraction necessary to decelerate the movement. Hypermetria is associated with a more gradual buildup and prolongation of agonist activity with a delayed onset of antagonist activity, or with a slower rate of rise of activity in the antagonists. Evidence suggests that different mechanisms may underlie dysmetria, depending on the anatomical location of the cerebellar lesion.
Agonist-Antagonist Coordination
A disturbance in reciprocal innervation results in a loss of the ability to stop the contraction of the agonists and rapidly contract the antagonists to control and regulate movement. In patients with cerebellar deficits attempting to make rapid, voluntary movements, the first agonist burst is frequently prolonged, the acceleration time is longer than normal, and the acceleration time may exceed the deceleration time. The normal triphasic agonist-antagonist-agonist sequence of activity is disturbed by a too long or too short agonist burst, or an agonist burst that continues into the antagonist activity. Impairment of the ability to carry out successive movements and to stop one act and follow it immediately by its diametric opposite causes dysdiadochokinesia, loss of checking movements, and the rebound phenomenon. Dysdiadochokinesia (or adiadochokinesia) is a clumsy term (coined by Babinski) that means inability to make rapid repetitive movements or RAMs. The patient with impaired RAMs has difficulty with such tests as patting the palm of one hand alternately with the palm and dorsum of the other hand, rapid tapping of the fingers, tapping out a complex rhythm, or tapping the foot in steady beat. Inability to rapidly reverse an action also causes impairment of the check response, producing the Holmes rebound phenomenon (see section on “Impaired Check and the Rebound Phenomenon”).
Tremor
The most common type of cerebellar tremor is an intention (active, kinetic, or terminal) tremor that is not present at rest but becomes evident on purposeful movement. In the upper extremity, when the patient reaches to touch an object, there are irregular, to-and-fro, jerky movements perpendicular to the path of movement that increase in amplitude as the hand approaches the target. A postural tremor of the outstretched limbs may also occur, without the patient reaching for a target. Cerebellar tremor often involves the proximal muscles. When severe, cerebellar tremor may involve not only the extremities but also the head or even the entire body. Severe cerebellar tremor may at times take on an almost myoclonic character; some conditions cause both cerebellar ataxia and myoclonus. The tremors and other movements probably result from disease involving the cerebellar efferent pathways or their connections with the red nucleus and thalamus (dentatorubral and dentatothalamic pathways, or SCP), and are sometimes referred to as a cerebellar outflow tremor. A rubral tremor is present at rest but worsens with action and probably results from a lesion involving the cerebellar outflow tracts (see Chapter 30).
Hypotonia
Hypotonia, or muscle flaccidity, with a decrease in resistance to passive movement, is often seen in cerebellar disease. Cerebellar dysfunction results in a decrease in the tonic output of the cerebellar nuclei, causing loss of cerebellar facilitation to the motor cortex. The muscles are flabby and assume unnatural attitudes; the parts of the body can be moved passively into positions of extreme flexion or extension. The stretch reflexes are normal or diminished in disease limited to the cerebellum. Occasionally, the tendon reflexes are “pendular.” Tapping the patellar tendon with the foot hanging free results in a series of to-and-fro movements of the foot and leg before the limb finally comes to rest. Pendular reflexes are caused by muscle hypotonicity and the lack of normal checking of the reflex response. The superficial reflexes are unaffected by cerebellar disease. Cerebellar disease may also cause a characteristic position of the extended hand, probably because of hypotonia. The wrist is flexed and arched dorsally, with the fingers hyperextended, and a tendency toward overpronation. The hand is similar to that seen in Sydenham’s chorea. A cerebellar lesion may cause a decrease in the normal pendular movement of the affected arm when walking. A decreased arm swing may also occur with extrapyramidal disorders and with mild hemiparesis. In the shoulder-shaking test, a cerebellar lesion causes an increase in the range and duration of swinging of the involved arm, although the movements may be irregular and nonrhythmic (see Chapter 28).
Dysarthria
Cerebellar disease often affects speech. Articulation may be slow, ataxic, slurred, drawling, jerky, or explosive in type because of dyssynergy of the muscles of phonation. A scanning type of dysarthria is particularly characteristic ofcerebellar disease (see Chapter 9). The scanning speech of multiple sclerosis and the staccato speech of Friedreich’s ataxia (FA) are probably the result of cerebellar dysfunction. Dysarthria may be an isolated manifestation of paravermal cerebellar infarction.
Nystagmus
Nystagmus and other disturbances of ocular motility may occur with lesions of the cerebellum. Nystagmus often indicates involvement of vestibulocerebellar pathways. The ocular abnormalities often result from involvement of the connections of the cerebellum with other centers rather than actual cerebellar dysfunction. Cerebellar disease may cause gaze paretic nystagmus. The patient is unable to sustain eccentric gaze and requires repeated saccades to gaze laterally. With a lesion of one hemisphere, the eyes at rest may be deviated 10 to 30 degrees toward the unaffected side. When the patient attempts to gaze elsewhere, the eyes saccade toward the point of fixation with slow return movements to the resting point. The movements are more marked and of greater amplitude when the patient looks toward the affected side. When a tumor of the cerebellopontine angle is present, the nystagmus is coarse on looking toward the side of the lesion and fine and rapid on gaze to the opposite side (Bruns’ nystagmus). Other ocular motility disturbances seen with cerebellar disease include skew deviation, ocular dysmetria, ocular flutter, opsoclonus, ocular tilt reaction, and saccadic intrusions. Rebound nystagmus is a type of nystagmus that may be unique to cerebellar disease; the fast component is in the direction of lateral gaze, but transiently reverses direction when the eyes come back to primary position (see Chapter 14).
Other Abnormalities
Abnormalities of posture and gait with abnormal attitudes and spontaneous deviation of the head and parts of the body may be seen in cerebellar disease. In unilateral cerebellar disease, there may be deviation of the head and body toward the affected side, with past pointing of the extremities toward the affected side. When standing, there is an inclination to fall, and when walking a tendency to deviate, toward the side of the lesion. The outstretched extremities deviate laterally, toward the affected side. There may be a decrease or absence of the normal pendular movement of the arm in walking. In midline, or vermis, lesions, the patient may not be able to stand erect and may fall either backward or forward. The gait is staggering, reeling, or lurching in character, without laterality. “Cerebellar fits” is an antiquated term, referring to episodes of decerebrate rigidity because of brainstem dysfunction due to mass effect from lesions in the cerebellum.
EXAMINATION OF COORDINATION AND CEREBELLAR FUNCTION
Clinical tests for cerebellar dysfunction are basically designed to detect dyssynergia, decomposition of movement, and dysmetria. The combination of incoordination, awkwardness, errors in the speed, range and force of movement, along with dysdiadochokinesia, and intention tremor is referred to as cerebellar ataxia. Simple observation can be as informative as a detailed clinical examination. Watching as the patient is standing, walking, dressing and undressing, buttoning and unbuttoning clothing, and tying shoelaces may reveal tremor, incoordination, clumsiness, and disturbed postural fixation. The patient may be asked to write, use simple tools, drink from a glass, and trace lines with a lightweight pen while no support is given at the elbow. The examination of infants and children may be limited to simple observation, noting the child’s ability to reach for and use toys and objects. Tests for coordination may be divided into those concerned with equilibratory and nonequilibratory functions.
Equilibratory Coordination
Equilibratory coordination refers to the maintenance of balance and the coordination of the body as a whole. The examination of station and gait assesses equilibratory coordination; these are discussed further in Chapter 44.
Nonequilibratory Coordination
Tests of nonequilibratory coordination assess the patient’s ability to carry out discrete, oftentimes relatively fine, intentional movements with the extremities. Although these are primarily tests of coordination, other neural systems must be intact for normal performance. The other components affecting fine motor control are discussed in Chapter 27. It is important to consider handedness in judging coordination, and to allow for the normal slight clumsiness of the nondominant side. Patients who are fatigued or sedated may have incoordination that is not normal for the individual. Fine motor skills may also be assessed functionally by asking the patient to do such things as thread a needle, pick up a pin, string beads, pour water, or draw circles.
The Finger-to-Nose (Finger-Nose-Finger) Test
There are several variations on the theme of having the patient touch his index finger to his nose, all of which will be included as the finger-to-nose (FTN) test. All may be carried out with the patient lying, seated, or standing. The patient extends the arm completely and then touches the tip of the index finger to the tip of the nose, slowly at first, then rapidly, with the eyes open and then closed. The examiner may place the outstretched extremity in various positions and have the test carried out in different planes and from various angles. The patient may be asked to touch the tip of his index finger to his nose, then touch the tip of the examiner’s finger, and then back to the tip of his nose. It is helpful to demonstrate the requested movement, lest the patient make some odd interpretation of the verbal request; an occasional patient will attempt to put his index finger on the examiner’s finger without removing it from his own nose. The examiner’s finger may be moved about during the test, and the patient asked to try to touch the moving target as the finger is placed in different locations at different distances and to move both slowly and quickly. The examiner may pull his finger away and make the patient chase it; fully extending the arm in this way can bring out mild intention tremor.
During these movements, note the smoothness and accuracy with which the act is executed and look for oscillations, jerkiness, and tremor. An intention tremor becomes more marked, coarse, and irregular as the finger approaches the target. There may be little tremor during the midrange of the movement, but near the end the tremor erupts; when the finger contacts the target, the tremor stops. In cerebellar ataxia, the difficulty may vary from slight incoordination, with a blundering type of movement, to wild oscillations causing complete inability to execute the act. A patient with severe appendicular ataxia may not be able to touch hand to head, much less finger to nose. For a video of appendicular ataxia with intention tremor, see http://www.youtube.com/watch?v=5eBwn22Bnio.
With dysmetria, the patient may stop before he reaches his nose, pause and then complete the act slowly and unsteadily, or overshoot the mark and bring the finger to the nose with too much speed and force. With dyssynergy, the act is not carried out smoothly and harmoniously; there may be irregular stops, accelerations, and deflections, or the movement may disintegrate into its component parts. Performing the FTN test against slight resistance may cause mild ataxia to become more obvious, or latent ataxia evident. The examiner may apply resistance by placing his fingers against the patient’s forearm and exerting slight pressure as the patient moves his arm toward the nose, or by placing a long rubber band around the patient’s wrist and pulling gently on it during the test. Another test is to have the patient draw a line, starting and then stopping at fixed points. He may have difficulty in starting at the correct point and may either stop short of the second point or overshoot the mark. This may also demonstrate tremor, with side-to-side oscillations along the intended tract. The patient with cerebellar disease may have macrographia, using large characters that become larger across the page, the opposite of the writing disturbance seen in Parkinson’s disease.
In the finger-to-finger (fingertips in the midline) test, the patient abducts the arms widely to the horizontal and then brings in the tips of the index or middle fingers through a wide arc to touch them exactly in the midline. This is done slowly and rapidly, with the eyes first open and then closed. With unilateral cerebellar disease, the finger on the involved side may fail to reach the midline, and the finger on the normal side may cross the midline to reach it. Also, the arm on the affected side may sag or rise, causing the finger on that side to be below or above the one on the normal side.
In hysteria or malingering, there may be bizarre responses of various types. The patient may act as if unable to touch the finger to the nose, or circle around it with widespread, wandering movements but eventually touch the very tip. Or the patient may repeatedly but precisely touch some other part of the face, implying there is no loss of sensation or coordination.
Similar tests may be used to evaluate the lower extremities. In the heel-to-shin (heel-knee-shin/toe) test, the patient is asked to place the heel of one foot on the opposite knee, tap it up and down on the knee several times, push the point of the heel (not the instep) along the shin in a straight line to the great toe, and then bring it back to the knee. The patient with cerebellar disease is likely to raise the foot too high, flex the knee too much, and place the heel down above the knee. The excursions along the shin are jerky and unsteady. With sensory ataxia, the patient may have difficulty locating the knee with the heel, groping around for it; there is difficulty keeping the heel on the shin, and it may slip off to either side while sliding down the shin. In the toe-to-finger test, the patient tries to touch his great toe, knee bent, to the examiner’s finger. If there is dysmetria, he will undershoot or overshoot the mark; intention tremor and oscillations may also be evident. The patient may be asked to draw a circle or a figure eight with his foot, either in the air or on the floor; in ataxia, the movement will be unsteady and the figure irregular.
Rapid Alternating Movements
With dysdiadochokinesia, one act cannot be immediately followed by its diametric opposite; the contraction of one set of agonists and relaxation of the antagonists cannot be followed immediately by relaxation of the agonists and contraction of the antagonists. Patients with cerebellar ataxia may have great difficulty making these kinds of movements. A common test for dysdiadochokinesia is to have the patient alternately pronate and supinate his hands, as in patting alternately with the palm and dorsum of the hand on the thigh or on the palm or dorsum of the other hand, or imitating screwing in a light bulb or turning a doorknob. The movements are performed repetitively and as rapidly as possible. Any movement involving reciprocal innervation and alternate action of agonists and antagonists can be used, such as alternate opening and closing of the fists, quickly flexing and extending individual fingers, touching the tip of the index finger to the tip or extended interphalangeal joint of the thumb, or patting rapidly against a table top with hand or fingertips. A good test is to have the patient touch the tip of his thumb with the tip of each finger rapidly and in sequence—starting with the index finger and proceeding to the little finger, repeating with the little finger and going to the index finger, and so forth. Another good test is to have the patient tap out a simple rhythm with each hand (e.g., 1-2-3/pause in steady beat), and then a more complex but familiar rhythm (e.g., Happy Birthday song). Testing RAMs in the lower extremity is much more limited. The patient may be asked to pat the foot steadily, against the floor if standing, and against the examiner’s palm if recumbent or to repetitively touch the heel up and down to the knee if supine. RAMs of the tongue may be tested by having the patient move the tongue in and out or from side to side as rapidly as possible.
In all of these tests, note the rate, rhythm, accuracy, and smoothness of the movements. In patients with ataxia, the RAMs are either carried out slowly and hesitantly, with pauses during transition between the opposing motions, or unsteadily and irregularly, with loss of rhythm. There may be a rapid fatigability: The movements may be executed satisfactorily in the beginning, but after a few attempts they become awkward and clumsy. The two extremities are usually compared, but patients with bilateral abnormalities are common, and the examiner must rely on experience or use another control. Demonstrating the movements to the patient provides an opportunity for the examiner to be the control. For some maneuvers, such as rapid, repetitive finger movements, the two extremities can be examined simultaneously and one side compared with the other. Simultaneous testing may also cause accentuation of the abnormality on the affected side.
Impaired Check and the Rebound Phenomenon
Checking movements involve contraction of the antagonists after a load is unexpectedly removed during strong contraction of the agonist. The agonists must immediately relax and the antagonists must contract to provide braking after the sudden release of resistance. Since cerebellar dysfunction causes impairment of the reciprocal relationship between agonist and antagonist, patients may have impairment of the checking response.
In the Holmes (Stewart-Holmes) rebound test, the patient holds the arm adducted at the shoulder and flexed at the elbow, with the forearm supinated and the fist firmly clenched. The elbow may rest on a table or be held unsupported close to the body. The examiner pulls on the wrist, and the patient strongly resists the examiner’s attempt to extend the elbow. The examiner then suddenly releases his grip on the wrist. Normally, with the sudden unloading, the contraction of the elbow flexors immediately ceases and is rapidly followed by contraction of the elbow extensors to arrest the sudden flexion movement and stop the patient from hitting himself. The normal patient is able to control the unexpected flexion movement of the elbow. In cerebellar disease, when the strongly flexed extremity is suddenly released, the patient cannot stop the flexor contraction and engage the extensors to stop the elbow movement. Because of loss of the checking response, the fist flies up to the shoulder or mouth, often with considerable force. The examiner’s free arm should be placed between the patient’s fist and face to block the blow. The prevalent description of this as the Holmes rebound phenomenon is not precisely correct. Stewart and Holmes used rebound to refer to the jerk back in the opposite direction, the recoil, on release of the restraint. The rebound phenomenon is present normally and exaggerated in spastic limbs. It is the absence of rebound (usually accompanied by impaired checking) in limbs affected by cerebellar disease that is abnormal. The rebound test may be carried out in other ways. Elbow extension against resistance may be tested instead of flexion. With both arms outstretched in front of the patient, the examiner may press either down or up on them as the patient resists and then suddenly lets go. This allows comparison of the rebound phenomenon and loss of checking movements on the two sides. In the lower extremities, rebound can be tested by sudden release after the patient has been resisting either flexion or extension at the knee, hip, or ankle. Impaired checking and the rebound phenomenon are not invariably present in cerebellar disease and may sometimes be present in normal limbs or even exaggerated in spastic limbs. An abnormal rebound test unilaterally is more significant than when present bilaterally. In the arm-stopping test, the patient holds both arms overhead or by his sides, the examiner holds his arms outstretched horizontally, and then the patient tries to quickly bring his arms up or down so that his fingertips are at the exact same level as the examiner’s. With a unilateral hemispheric lesion, the good arm will stop on target, the affected arm often overshoots and then corrects in the opposite direction, oscillating around the target before eventually coming to rest.
Deviation and Past Pointing
Patients with cerebellar disease often have difficulty maintaining normal alignment of the limbs or body when performing a task such as holding the arms outstretched or walking, especially with eyes closed. The patient may miss when trying to reach out to touch a target (past pointing), drift to one side when walking eyes closed, or have drift of the outstretched arm. Similar findings may occur with vestibular lesions.
To perform the traditional test for past pointing, the patient and examiner should be facing, either seated or standing, the outstretched upper extremity of each held horizontally with the index fingers in contact. The patient raises his arm to a vertical position, finger pointed directly upward, and then returns to horizontal to again touch the examiner’s finger. The maneuver should be tried a few times with the eyes open and then executed with the eyes closed. The arms may be tested sequentially or simultaneously. The test is less commonly done with the patient raising the arm from below up to the horizontal. Normally, the patient will return to the starting position fairly accurately, without any drift or deviation. In labyrinthine disease or with a cerebellar hemispheric lesion, the arm will deviate to the involved side on the return track, more so with the eyes closed. This deviation is called past pointing. A simpler way to test for past pointing is to have the patient close his eyes while doing the finger-nose-finger test. With eyes open, the pointing is accurate, but with eyes closed, the patient points off to the side of the target. Repeating the test several times may produce greater deviation. With severe lesions, past pointing may occur even with eyes open. The pattern is different in vestibular as opposed to cerebellar past pointing. In vestibular disease, past pointing occurs with both upper extremities toward the involved side; in unilateral cerebellar disease, past pointing occurs toward the side of the lesion, but only in the ipsilateral arm. Past pointing is discussed further in Chapter 17.
A cerebellar lesion may also cause drift of the outstretched upper extremities. Three types of drift may occur when the patient attempts to hold the arms outstretched with eyes closed: pyramidal drift, parietal drift, and cerebellar drift. In pronator drift (Barré’s sign) due to a pyramidal lesion, the arm sinks downward and there is accompanying pronation of the forearm (see Chapter 27). In parietal drift, the arm usually rises and strays outward (updrift). With cerebellar drift, the arm drifts mainly outward, either at the same level, rising, or less often sinking. Testing is done with arms outstretched and eyes closed. With disease involving one cerebellar hemisphere, the arm drifts toward the side of the lesion. The deviation may be accentuated by having the patient raise and lower the arms several times, or by tapping the patient’s outstretched wrists. Tapping on the wrists may also create an up-and-down oscillation because of impaired checking, so that the arm swings up and down a few times and gradually drifts laterally and often upward.
Position holding can also be tested in the lower extremities. The patient, lying supine, raises the legs one at a time. When there is ataxia, the leg cannot be lifted steadily or in a straight line. There may be adduction, abduction, rotation, oscillations, or jerky movements from one position to another. When the limb is lowered, the patient may throw it down heavily, and it may not return to its original position beside its mate but may be deviated across it or away from it. When the seated patient extends the legs without support and attempts to hold them steady, a unilateral cerebellar lesion may cause oscillations and lateral deviation of the ipsilateral extremity. The extended supported leg may show an increased range and duration of pendulousness when released or given a brisk push. If the prone patient bends the knees and tries to maintain the shins vertically, there may be marked oscillations and lateral deviation of the leg on the side of the lesion.
Deviation and drift may also occur when the patient tries to walk with eyes closed. As in vestibulopathy, the patient drifts to the side of the lesion (see Chapter 17). Walking back and forth with eyes closed may reveal a “compass” or “star” gait due to deviation toward the involved side. When walking around a chair, the patient shows a tendency to fall toward the affected side.
CEREBELLAR SYNDROMES
Cerebellar disease may affect all or only a specific part of the cerebellum. There are two clearly defined cerebellar syndromes: a midline or vermis syndrome and a lateral or hemispheric syndrome. With the vermis, or midline, syndrome, the outstanding symptoms are abnormalities of station and gait, with abnormalities ranging from slight widening of the base on walking in mild disease (gait ataxia) to total inability to sit or walk in severe disease. Disease of the cerebellar hemispheres produces appendicular ataxia, disturbance in coordination of the ipsilateral extremities, the arm more than the leg. The primary clinical manifestations of dysfunction of the FN lobe or its connections are disturbances of equilibrium; nystagmus, often positional; and other abnormalities of extraocular movement. There is no limb ataxia. Table 43.1 summarizes the clinical manifestations of disease of these parts of the cerebellum.
TABLE 43.1 Clinical Manifestations of Disorders of the Cerebellum (Related to the Different Zones of the Cerebellum)
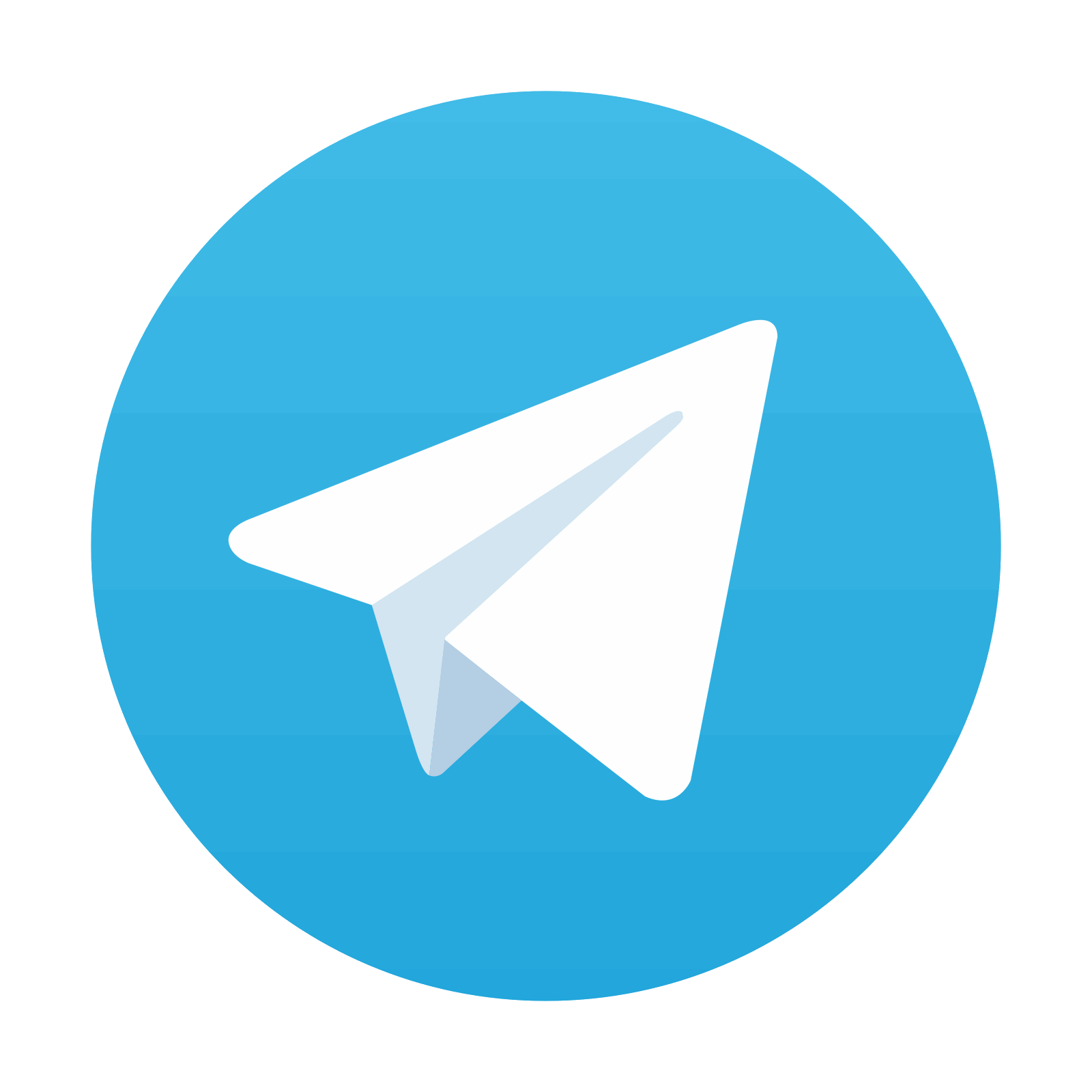