30 The Role of Radiation Therapy in Skull Base Pathologies • Radiation induces double-strand breaks within DNA to kill tumor cells. • Normal tissues have a superior ability to repair radiation-induced DNA damage compared with tumor cells. This difference is exploited in therapeutic radiology. • Radiation has been traditionally given in small daily fractions 5 days/week over several weeks. • Radiation therapy is usually delivered using a linear accelerator (LINAC) to deliver mega-voltage photons, which are particles without charge or mass. • The unit of radiation is the gray (Gy) and 1 Gy is equal to 1 joule (J) of energy absorbed per kilogram of matter. • Daily doses of radiation are usually in the range of 1.8 to 2.0 Gy. • Total radiation doses depend on the tumor type and its histology, but the following are general principles: • Refers to radiation therapy that uses computed tomography (CT)-based planning. • The tumor and structures that are critical to avoid irradiating, such as the brainstem or optic nerves, are contoured on axial CT images. • Radiation therapy is planned, usually with two to six beams, with the goals of treating the tumor and avoiding critical structures. • The inability to shape the radiation beam to avoid critical normal structures (i.e., “modulate”) is the major limitation of 3D-CRT. • A major advance that built upon the foundation of 3D-CRT. • IMRT is the result of improvements in LINAC technology, treatment planning software, and the advent of multileaf collimators (beam-shaping devices that consist of leaves that move in and out of the field of irradiation to provide maximal exposure where tumor is visible and maximal sparing of eloquent normal structures where a critical structure is in the beam’s eye view). • Essentially it is now possible to shape the radiation around the critical structures to deliver higher doses at the tumor-critical structure interface, and minimize tumor underdosing (Fig. 30.1). • IMRT is the standard of care for treating complex tumors in the base of the skull (e.g., chordoma, meningioma) and the head and neck (e.g., nasopharynx and paranasal sinus), and it is now widely applied across tumor sites. • Incorporates three-dimensional imaging in the process of radiation delivery. • Prior to each treatment delivery, an imaging study is performed to confirm alignment of the target volume prior to treatment delivery. • Imaging can consist of either orthogonal diagnostic quality X-rays that can render three-dimensional information, a limited volume CT scan with an on-board cone beam (CBCT), in-room CT (CT on rails), and, most recently, an integrated magnetic resonance imaging (MRI) (Viewray Inc., Cleveland, OH). • IGRT increases the likelihood that treatment is delivered to the target volume, and allows for reduced margins applied for uncertainties beyond the target volume. • Stereotactic radiotherapy (SRT) is the precise delivery of focused irradiation to small targets using an advanced image-guidance delivery apparatus and patient immobilization systems. • SRT is a resource-intensive treatment that requires specific equipment and practitioners with expertise in treatment planning. • SRT requires prolonged treatment times on the unit, making it a specialized technique for selected applications. • Submillimeter precision in therapy is mandated: • Fundamentally, the delivery of stereotactic radiation requires the following: Stereotactic radiation apparatus can be categorized as follows: • LINAC-based stereotactic radiation • Stereotactic robotic radiosurgery • Gamma Knife • Charged particle therapy units • The management of meningiomas depends on several factors: location, symptomatology, size, grade, and patient age. • Observation may be reasonable for slow-growing lesions in asymptomatic patients. • Late recurrences are common, and thus evaluation of therapeutic efficacy requires more than 5 years of follow-up. • Reviews of nearly 1,200 patients have demonstrated > 90% rates of 5- to 10-year progression-free survival (PFS) for patients treated with SRS or external beam radiation therapy (EBRT) in recent years.5 • Both SRS and EBRT are reasonable options for treatment of meningioma. The ultimate choice should depend on available expertise, patient preferences, and tumor location in relationship to critical structures. • Of note, for patients who decline, or are not good candidates for resection, definitive radiation has been used with excellent results. In a series of 189 patients with skull base meningioma treated to a median dose of 56.8 Gy, control rates were similar in patients who underwent primary EBRT (59 patients) and in those who underwent surgery followed by EBRT (130 patients).6 • For SRS, doses between 13 and 15 Gy are commonly used for grade 1 tumors. Grade 2 and 3 tumors typically are not treated with SRS. • Gross total resection (Simpson grade 1) for grade 1 (benign) meningioma is considered definitive treatment (see Chapter 18). A review of this approach demonstrated relapse rates at 5 years of 7 to 12%, which increase to 20 to 25% by 10 years.5,7–9 • Results of subtotal resection, even for benign tumors, are suboptimal. A review of over 3,500 patients reported recurrence rates greater than 35% at 5 years and 50% at 10 to 15 years of follow-up. The addition of postoperative radiation can yield PFS rates of over 90% at 10 years of follow-up.10 • Grade 2 (atypical) meningioma has a seven- to eightfold increased risk of recurrence in the first 5 years of treatment following surgery alone, with recurrence rates of 38 to 62% and increasing to 81% at 8 years in one study.7,11–14 • Given the benefit of postoperative radiation for STR in benign tumors, the addition of radiation to atypical meningioma is relatively well accepted10; however, it is controversial as a routine practice following GTR of atypical meningiomas in non-eloquent observable areas of primary tumor. • Grade 3 (malignant) tumors are even more aggressive, with 5-year recurrence rates of 56 to 83% and a median survival of less than 2 years.11,13,14 • Given the poor outcomes with malignant meningioma, a reasonable approach is to offer all patients adjuvant irradiation. • A series of 168 patients with petroclival meningioma who underwent SRS to a median dose of 13 Gy has been reported.18 • A multi-institutional analysis, which included 763 patients treated to a median dose of 13 Gy, detailed the outcomes after SRS for sellar and parasellar meningiomas19: • Recurrent meningiomas should be managed aggressively. • Rates of recurrence appear to be improved with surgery and irradiation, with one study reporting an 8-year PFS of 78% with combined therapy versus 11% with surgery alone.20 • There results were corroborated by another study demonstrating a 5-year PFS of 89% with combined therapy versus 30% with surgery alone. This study also demonstrated a 5-year overall survival (OS) benefit with the addition of radiation over surgery alone in the recurrent setting, 89% versus 43%, respectively.21 • Results of stereotactic radiosurgery • Results of fractionated stereotactic radiation therapy Pearls • SRS is a reasonable option for cavernous sinus meningiomas up to 3 cm in size and at least 2 mm away from the optic nerves, chiasm, and optic tract. • Larger tumors or those close to critical structures, such as the optic chiasm, may be better served by FSRT. • Further study is needed to see if protons offer clinical advantages over IMRT and SRS. • Radiation therapy is generally used in four instances: incomplete resection, recurrent tumor, inoperable patient, and refractory secretory tumor. • A limitation of radiation is that normalization of hormonal hypersecretion can take months to years, depending on the tumor type and radiation technique. • Other considerations include tumor size and distance from the optic chiasm. • The optimal SRS candidate has a tumor < 3 cm in maximal dimension that is at least 2 to 3 mm away from the optic apparatus. • Recommended doses of radiation are as follows: 45–54Gy in 1.8–2.0 Gy daily fractions (regardless of secreting or nonsecreting status); 15 to 20 Gy for non-secreting tumors undergoing single-fraction SRS; and 20 to 25 Gy for secreting tumors undergoing SRS. • A review of results achieved with SRS demonstrated an overall 50% rate of control of secretion for hypersecreting tumors.26 • The rate of local control was 90% for nonsecreting tumors in a review of patients treated with SRS or FSRT.27 • Nonfunctioning tumors • Cushing’s disease • Prolactinoma • EBRT in nonfunctioning tumors • EBRT in Cushing’s disease • EBRT in acromegaly • EBRT in prolactinoma • Toxicity of SRS • Toxicity of EBRT Pearls • Radiation therapy has a critical role in the management of pituitary tumors. • The most common side effect is hypopituitarism. • Both EBRT and SRS show excellent rates of local control for nonfunctioning tumors. • SRS is preferable in secretory tumors due to faster rates of normalization of hormonal levels, potentially lower rates of hypopituitarism, and logistical ease.
Introduction to Radiation Therapy
Background
This facilitates maximal normal tissue healing, and time for tumor reoxygenation and greater radiation sensitivity.
Radiation Dose
50 Gy/25 fractions (fx): used to sterilize microscopic malignant disease such as in the clinically negative neck at high risk for lymphatic nodal spread where few cells are likely harbored, or for the treatment of benign skull base tumors (e.g., glomus tumors, benign meningiomas, vestibular schwannomas)
60 Gy/30 fx: used in the postoperative setting to control low-volume malignant microscopic residual disease within the postoperative bed, or for the treatment of high-grade skull base tumors such as atypical meningioma
70 Gy/35 fx (or 78 Gy/39 fx): used to control gross tumor with curative intent and for some radioresistant histologies that are considered “non-malignant” (e.g., chordoma)
Three-Dimensional Conformal Radiation Therapy (3D-CRT)
If a critical structure is adjacent to the tumor, it may be impossible to deliver the desired dose to the tumor without overdosing the critical structure. In this situation, either tumor underdosing or acceptance of the risk of treating to full dose is required, and typically tumor underdosing can be considered acceptable in order to avoid iatrogenic harm.
Intensity-modulated Radiation Therapy (IMRT)
Image-Guided Radiation Therapy (IGRT)
Stereotactic Radiation
Fractionated stereotactic radiation therapy (FSRT): Conventionally fractionated radiation therapy is delivered with stereotactic precision to maximize dose at the tumor-critical structure interface and minimize normal tissue exposure.
Stereotactic body radiation therapy (SBRT): Stereotactic radiation therapy is delivered in a limited number of fractions, usually less than five, to an extracranial site.1
Stereotactic radiosurgery (SRS): Traditionally referred to as a treatment using stereotactic techniques, SRS entails delivering the entire dose of radiation in a single fraction.
Near-rigid or rigid immobilization devices
IGRT
IMRT
Advanced radiation oncology delivery apparatus
Rigorous quality assurance
Treatment Delivery Units
LINACs can be outfitted with subcentimeter width multileaf collimators leaves or stereotactic cones, image-guidance systems, and robotic couch tops.
Together these features enable submillimeter motions in all six degrees of freedom, ensure precise patient positioning, deliver millimetric precision, and provide conformal dose distributions with steep gradients beyond the tumor edge to minimize normal tissue exposure.
Given that these machines are capable of conventional 3D-CRT, IMRT, and stereotactic treatments, they are the most commonly used machines to deliver stereotactic radiation.
Stereotactic robotic radiosurgery refers to a dedicated LINAC system used to deliver SRS, FSRT, and SBRT.
It consists of a miniaturized LINAC mounted onto a robotic arm that is able to move in all six degrees of freedom; for example, Cyberknife® (Accuray Inc., Sunnyvale, CA).
The image-guidance system consists of a pair of orthogonal X-rays (i.e., stereoscopic X-ray) that can render three-dimensional information of the target location based on the imaging surrogate (a fiducial or bone in selected circumstances).
The stereoscopic X-ray system has the advantage of being able to image the patient during treatment delivery in near-real time.
The robotic arm also allows the LINAC to adjust for positioning inaccuracies and beam angles that are not possible using a conventional linear accelerator based system.
The Gamma Knife (GK; Elekta AB, Stockholm, Sweden) is the first dedicated brain SRS device and was invented by Lars Leksell.
The most recent version is the Gamma Knife Perfexion® (Elekta AB).
The system is based on cobalt sources, each emitting a beam of radiation that is collimated.
A total of 192 beams are concentrated on a spot localized in three dimensions to deliver a single dose or “shot” of radiation with submillimeter precision.
By applying several shots of radiation to cover the target, high doses in a single session are delivered with maximal sparing of the normal brain tissue as compared with other apparatuses.2
GK has traditionally required the head to be immobilized using a stereotactic head frame; however, noninvasive head frames are now available, and they can be equipped with cone-beam CT image-guidance to enable performing IGRT. Ultimately these innovations permit the GK to deliver multiple fraction radiation therapy (such as FSRT) and expand this technology’s scope beyond single-fraction SRS.
GK has been used for lesions in the brain, skull base, eye, and occasionally upper cervical spine.
Charged particle therapy most commonly refers to proton therapy, although there are also carbon ion centers (in Europe and Asia).
Protons and other charged particles, unlike photons, deposit the majority of their dose at a predictable depth, which is referred to as the Bragg peak.
Very little dose is deposited beyond the Bragg peak, and this is the advantage of protons as compared with photon-based therapy.
The Bragg peak has been exploited to treat tumors adjacent to critical structures such as the brainstem or optic structures; this is why charged particle therapy is often used for irradiation of skull base chordoma and chondrosarcoma.
Charged particle therapy is also helpful in reducing low-dose deposition within normal tissues in pediatric patients, and it is postulated that the benefit will be a reduced risk of radiation-induced secondary malignancies.
Treatments using protons or carbon ions are usually done with simple 3D-CRT plans, and require significant margins for uncertainty. As a result, the superiority of the technology as compared with modern linear accelerator systems able to deliver radiation with millimetric precision has been questioned.3 As a result, some centers are now treating tumors that were traditionally treated with proton therapy (such as chondrosarcoma and chordoma) with stereotactic LINAC-based techniques.
With new developments in proton delivery, such as intensity modulated proton therapy (IMPT) and the application of CBCT, the superiority of this technology may become evident once again.
Carbon ions have a greater relative biological effectiveness than photons or protons.4
With research and development, carbon ion therapy may be a highly useful technology for radioresistant tumor subtypes, but further study is needed to understand its application and implications.
Role of Radiation in Specific Pathologies of the Skull Base
Skull Base Meningioma
Toxicity in this series was low, with a 2.2% rate of grade 3 toxicity, consisting of reduced vision, a new visual field deficit, and trigeminal neuropathy.6
For large grade 1 meningiomas that are not amenable to gross total resection (GTR), it is still unknown whether subtotal resection followed by EBRT to residual disease or EBRT alone is preferred. It is unclear if the baseline pre-radiation tumor volume predicts local control.
Benign Meningioma
Atypical and Malignant Meningioma
A 108-patient study of atypical meningiomas that underwent GTR demonstrated a recurrence rate of 48% at 10 years. Eight of the patients received irradiation; none suffered a recurrence.15
A study of 45 patients who underwent GTR of atypical meningioma demonstrated local control in 92% of patients who underwent immediate irradiation versus 59% who underwent surgery alone.16
A systematic review, however, found that although adjuvant irradiation improved local control for atypical meningiomas, especially after subtotal resection, a statistically significant benefit of adjuvant irradiation after GTR could not be shown.17
Given the high recurrence rates for atypical tumors following resection alone, a suggested approach is to consider adjuvant irradiation in all patients, even those with GTR.
Petroclival Meningioma
SRS was used as the primary therapeutic modality in 97 patients, after STR in 32 patients, or for recurrent disease after GTR in 39 patients.
With a median follow-up of 72 months, 10-year PFS was 86%.
Twenty-six percent of patients had improvement in neurologic symptoms, 58% had stabilization of symptoms, 8% had clinical or neurologic deterioration, and 4% developed hydrocephalus requiring cerebrospinal fluid (CSF) diversion. SRS can provide durable control with acceptable rates of toxicity.
Sellar and Parasellar Meningioma
With a median follow-up of 67 months, the 10-year PFS rate was 82%.
Functional improvements were seen in 34% of patients with preexisting deficits, in contrast to 9.6% of patients who had new or worsening cranial nerve deficits.
Overall, 79.6% of patients had a favorable outcome, defined as tumor control with no new or worsened cranial nerve deficits. SRS provides excellent rates of local control with minimal morbidity.
Recurrent Meningioma
Cavernous Sinus Meningioma
SRS has been used in tumors near the cavernous sinus with good results (Table 30.1).
Results from a 15-patient series of cavernous sinus meningioma treated with single-fraction SRS to a median marginal dose of 16 Gy were reported.22
With a median follow-up of 89 months, the tumor control rate was 93% at 10 years.
Permanent complications occurred in 12% of patients at a median of 23 months post-SRS and included trigeminal nerve dysfunction, ischemic stroke, diplopia, and hypopituitarism.
The rate of complications was dependent on the size of the primary tumor: 3% for those ≤ 9.3 cc versus 21% for tumors ≥ 9.4 cc.
These results were similar to those from the University of Pittsburgh, in which 16 patients were treated with a dose of 13 Gy.
The control rate was 93% at 10 years, and the crude rate of toxicity was 6.7%.
The authors recommended SRS for cavernous sinus lesions < 3 cm in maximum diameter or < 15 cc in volume.23
Outcomes of fractionated stereotactic radiation in 222 meningioma patients, 78 of whom had cavernous sinus lesions were reported.24
The 10-year rate of local control was 86% overall, and 100% for cavernous sinus lesions.
Doses ranged from 50 to 55 Gy in 30 to 33 fractions.
The median tumor size was 12.0 cc in the 209 patients for whom data were available.
A review of 72 patients who were treated with 57 to 59 Gy using protons demonstrated a 5-year rate of local control of 96 to 99% in presumed grade 1 or biopsied lesions, and 50% in atypical tumors.25
Toxicities were considered acceptable at 12.5%, with adverse events consisting of hypopituitarism, optic nerve damage, and diplopia.
Pituitary Adenoma
Results Specific to SRS
Local control rates for nonfunctioning adenomas following single-fraction SRS range from 83 to 100%, with an average of 95.7%.27
Neurologic deficits are rare, with a range of 0 to 7.1%, with an average of 2.1%.27
Hypopituitarism ranges from 0 to 39%, with an average of 12%.27
The recommended SRS margin dose ranges from 12 to 18 Gy, and rates of < 12 Gy were shown to be inferior in at least one experience.28
Many adrenocorticotropic hormone (ACTH)-secreting pituitary adenomas demonstrate invasion into the dura or cavernous sinus, making surgical resection difficult.
SRS is useful in patients with unresectable or persistent Cushing’s disease following resection.
Rates of remission after SRS range from 16.7% to 87%, with the majority of results demonstrating control rates of > 50%.27
The average time to remission is 13 months following SRS (range 2–67 months).29
Biochemical control rates range from 0 to 92%, with an average of 43.6%.27
The mean time to endocrine remission appears longer than with Cushing’s disease, and was found to be 27.6 months in a single institution experience of 136 patients.30
Patients on octreotide in the perioperative period, were found to have a lower rate of biochemical control following SRS, at 11% versus 60% in patients not on such medications.31
Pharmacological therapy is the mainstay of treatment.
Patients selected for radiation therapy are thus the subset of patients with the most recalcitrant disease. This may help explain why biochemical control rates are lower than with acromegaly and Cushing’s disease.
SRS has demonstrated biochemical remission rates ranging from 0 to 83%, with an average of 29.4%.27
Patients on dopamine agonists at the time of SRS were found to have lower rates of biochemical control.31,32
Results of Fractionated Radiation Therapy
Doses of 45 to 54 Gy in 1.8- to 2-Gy daily fractions are typically used.
The rate of local tumor control following conventional radiation is greater than 90% in most series.27
A 24-patient series examining the results of proton therapy to 54 cobalt gray equivalents (CGEs) demonstrated 100% local control at a median follow-up of 47 months.33
In a series of 63 patients who underwent FSRT, tumor control was 100% at a median follow-up of 82 months.34
Remission rates of 0 to 84% have been reported, although the majority are within the 50 to 80% range.35
In a review of multiple series, SRS was associated with normalization of ACTH levels in a median of 7.5 to 33 months, whereas the median time with EBRT tend to be longer and ranged from 18 to 42 months.35
EBRT results in remission rates ranging from 16 to 100% at 5 to 10 years, with 50 to 60% expected with modern techniques.35
Overall time to remission appears longer as compared with SRS, with a mean time to remission of 6 to 10 years.35
A series using proton therapy reported a 45% endocrine control rate in 11 patients with a median follow-up of 47 months.33
EBRT has demonstrated complete endocrine normalization with radiation alone in 25 to 50% of patients, and in conjunction with medical therapy to 80 to 100%.35
The median time to response is quite variable, ranging from 1 to 10 years.35
Rates of hypopituitarism range from 20 to 40% following SRS, and the reported onset time ranges from 16 to 40 months in most reports; however, hypopituitarism has been reported at 100 months.26
The second most common toxicity is related to cranial nerve toxicity, and is estimated to occur in 2% of patients or fewer.27
Other rare side effects include ophthalmoplegia, transient ischemic attacks, temporal lobe epilepsy, transient hemiparesis, and memory loss.26
The rate of second malignancy following Gamma Knife SRS is low, as one study examining 5,000 patients followed for more than 10 years demonstrated no increased risk of secondary malignancies.36
Hypopituitarism is the most common side effect of pituitary irradiation.
In patients undergoing SRS for secretory tumors, it is recommended to withhold hormonal therapy for 6 to 8 weeks prior to treatment.
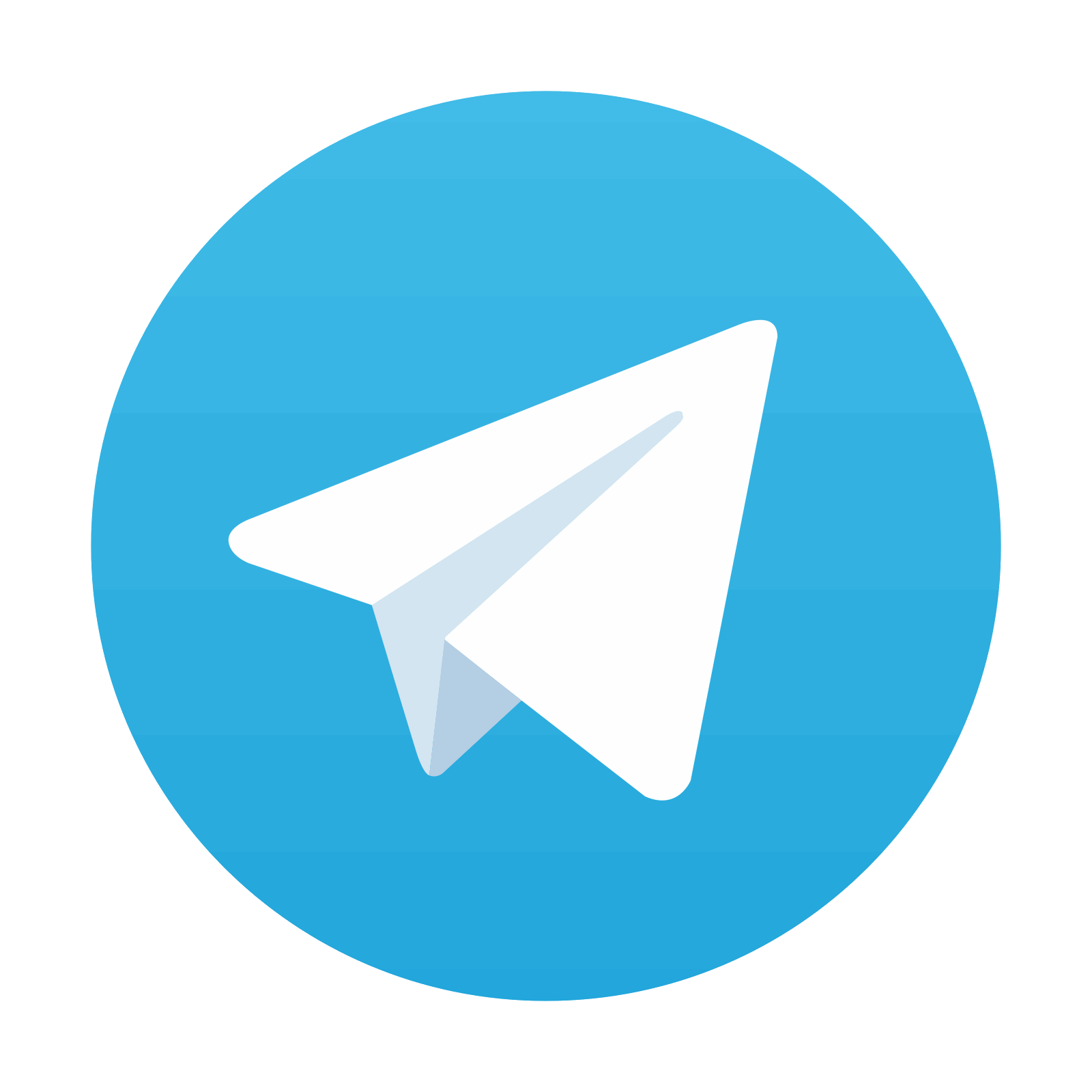
Stay updated, free articles. Join our Telegram channel

Full access? Get Clinical Tree
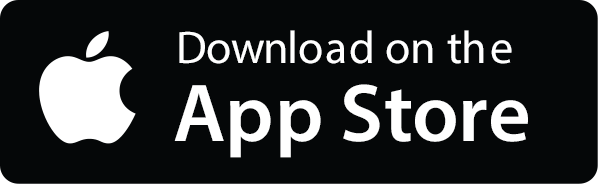
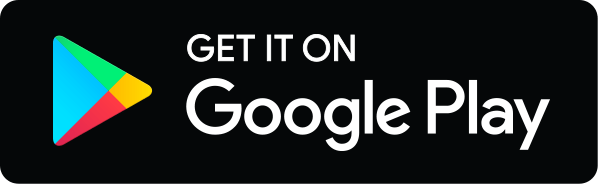