10 Neurophysiology in the Neurosurgical Intensive Care Unit: Options, Indications, and Interpretations
Abstract
The neurointensivist should at all cost prevent nervous tissue ischemia and infarction. The greatest decrease in nervous system blood flow is understandably when the brain or spinal cord is the most injured. When injury is extensive, function is minimal and therefore difficult to assess for minor changes that could lead to major injury. The neurointensivist fortunately has available at many, but not all, times, technology to assess changing physiological conditions that may lead to a change in neurosurgical intensive care unit management of the patient.
Case Presentation
A 70-year-old man, who works as a musician in an orchestra, is undergoing resection of a right cerebellopontine angle mass. Monitoring for the operation includes brainstem auditory evoked response (BAER) and somatosensory evoked potentials (SSEPs). During the resection there is an increase in SSEP latency by 50% in the left upper extremity and left lower extremity. BAER detects a prolonged III–V interpeak latency on the right.
See end of chapter for Case Management.
10.1 Electroencephalogram
The electroencephalogram (EEG) records the sum of neuronal activity of approximately 10,000 neurons within the pyramidal layer of the cerebral cortex, which is 1 cm from the cortical surface. 1 It is not useful to detect the function of the midbrain or brainstem. The electrode system uses channels (two electrodes per channel) arranged in different combinations, termed montages. Each channel reflects the summation of both excitatory and inhibitory potentials produced by the cell membranes between the two electrodes. Neurosurgical intensive care unit (NICU) EEG monitoring can be acute or chronic using a full montage to localize specific activity or four electrodes to determine the type of activity. EEG monitoring can be complicated by patient movement, head wrapping, transportation, need for computed tomographic (CT) scan or magnetic resonance imaging (MRI). Its role must be considered before or after other tests. Furthermore, with long-term use the scalp should be inspected for skin breakdown. EEG monitoring can be effectively used in the following 2 :
-
Seizure monitoring and differentiation.
-
Alterations in mentation due to clinically silent status epilepticus or metabolic dysfunction.
-
Intracranial pressure (ICP) monitoring.
-
Hydrocephalus.
-
Postoperative monitoring and depth of anesthesia.
-
Cerebral perfusion alterations.
-
Vasospasms.
-
Monitoring effectiveness of ischemia therapy.
The four basic frequency patterns generated by the brain are referred to as beta, alpha, theta, and delta. Beta is associated with acts of mental concentration, alpha can be found in relaxed patients who have their eyes closed, theta waveforms are often seen during general anesthesia and rapid eye movement (REM) sleep and head injury, and delta is most often pathological or representative of deep sleep (► Fig. 10.1).

The EEG electrodes are attached in a standardized fashion, termed the International 10–20 system (► Fig. 10.2, ► Fig. 10.3, ► Fig. 10.4). Even-numbered electrodes are on the patient’s right, and odd-numbered electrodes are on the left, while electrodes with a z are midline. The letters used are P for parietal attachment, T for temporal, O for occipital, F for frontal, and A for auricular.






10.1.1 Burst Suppression
Burst suppression is an abnormal pattern characterized by bursts of higher-voltage sharp wave (8–12 Hz) and slow wave (1–4 Hz) activity appearing out of a background of relatively suppressed voltage. A flurry of activity is seen, followed by a period of electrical silence on the tracing. This can be seen during high-dose anesthesia administration. A common use of burst suppression monitoring is the titration of neuroprotective medications. The end point of titration is the induction of the burst suppression pattern on the EEG of one to two electrical bursts per page (► Fig. 10.5). Bursts occurring less often have not been shown to be more neuroprotective. Giving higher doses of medication to achieve fewer bursts can be associated with lower blood pressure and therefore decreased cerebral perfusion. Common burst suppression doses are listed here 3 :

-
Pentobarbital: 10 mg/kg load, then 1 to 3 mg/kg/h.
-
Midazolam: 200 µg/kg as a slow intravenous (IV) bolus, followed by 0.75 to 10.0 µg/kg/min.
-
Etomidate: 0.4 to 0.5 mg/kg.
-
Propofol: 1 mg/kg loading dose, titrate down rapidly starting at 20 mg/kg/h.
10.1.2 Somatosensory Evoked Potentials
Somatosensory evoked potentials (SSEPs) allow for the monitoring of the spinal cord and peripheral nerves, as well as both cortical and subcortical structures. The evoked potential is generated when repetitive stimuli are applied to a peripheral nerve. Responses are then recorded centrally. Common nerves used are the median, ulnar, common peroneal, and posterior tibial. SSEPs are less affected by anesthesia than EEG and are more sensitive indicators of ischemia than EEG monitoring. SSEP waveforms move in parallel to regional blood flow and reflect changes when blood flow falls to near critical levels. It is notable to point out that SSEPs can be affected by medical equipment generating strong electrical fields (e.g., CT scanner is next door to the NICU). Any SSEP with amplitude reduction by more than 50% or latency increase by 1 millisecond should be further investigated. 4 , 5
SSEPs have the following NICU applications 4 :
-
Differentiating preganglionic versus postganglionic injury.
-
Evaluating peripheral nerve trauma and postoperative progress.
-
Evaluating postsurgical premotor and motor strip operations.
-
Investigating postoperative paraplegia after posterior spinal fusion.
-
Evaluating the comatose patient.
10.1.3 Limitations of SSEPs
SSEPs can be poor in predicting function in areas of the brain not involved with the somatosensory pathways.
10.1.4 Predictive Value of SSEPs
SSEPs have been used in evaluating the predicted prognosis of patients with spinal cord injury (SCI) in many studies. The tibial or common peroneal nerves are used, and the latency times are compared to those of normal subjects (► Fig. 10.6a). Further delineation between patients with ischemic and traumatic SCI allows for a more specifically tailored outcome. The outcome after rehabilitation can also be predicted using SSEPs (► Fig. 10.6b). 6

► Fig. 10.7 shows a normal median nerve SSEP. The labels used stand for Erb’s point (EP), a negative dorsal cervical spine potential (N13), a negative potential recorded from the contralateral scalp (N20), and a positive scalp potential (P22). The overlapping of tracings occurs as the previous tracing is retained while a new evoked potential is written over it for comparison. Each nerve has its own physiological limits on the SSEP. The criteria for an abnormal study are prolonged central conduction time, 1 abnormal internerve (right-left) central conduction time difference, 2 and absent EP, N13, N18, or N20 waves. 3 A typical value for the upper limit of normal for an EP-N20 is ~ 11 milliseconds.

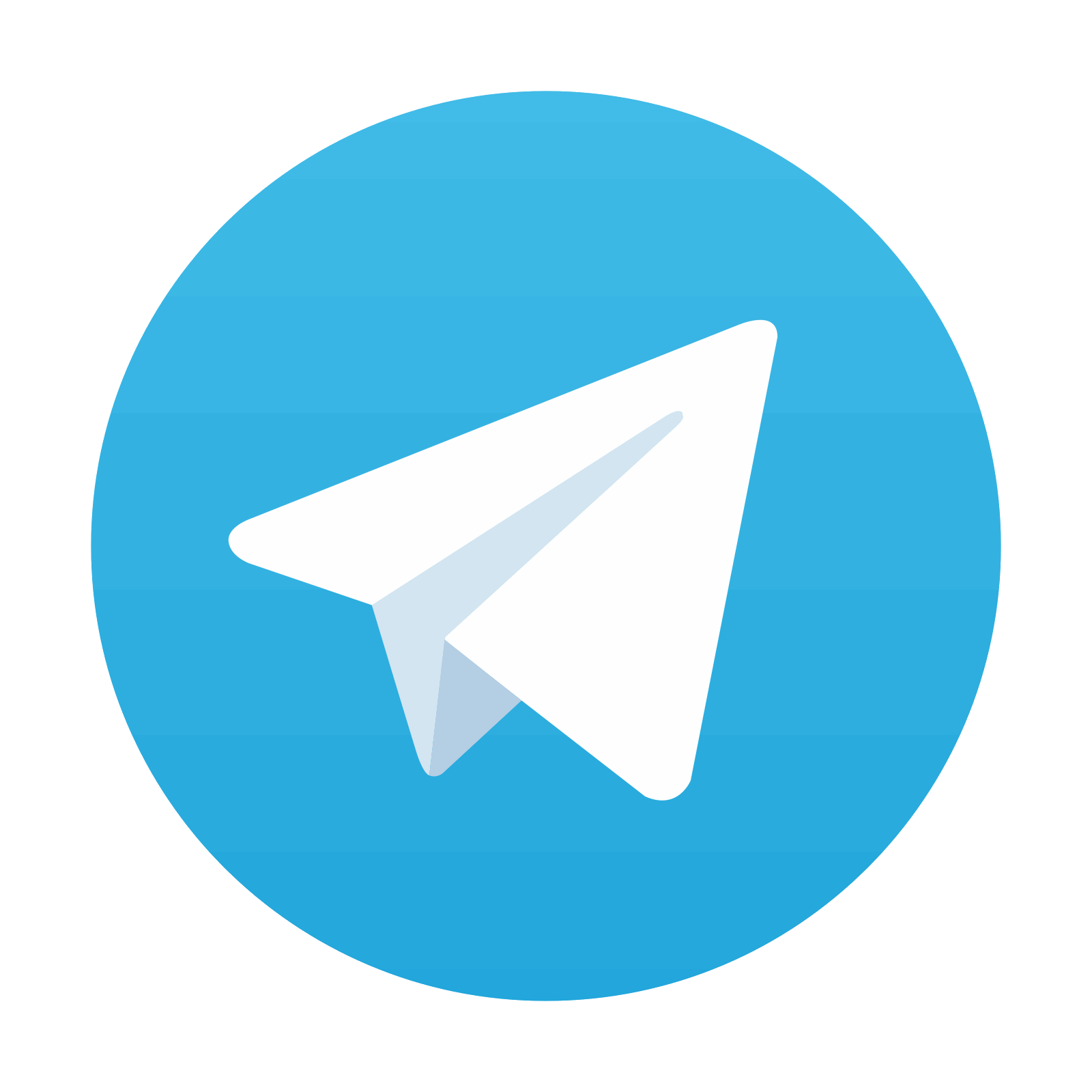
Stay updated, free articles. Join our Telegram channel

Full access? Get Clinical Tree
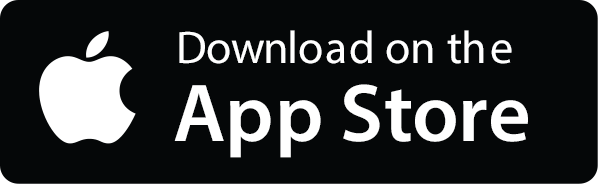
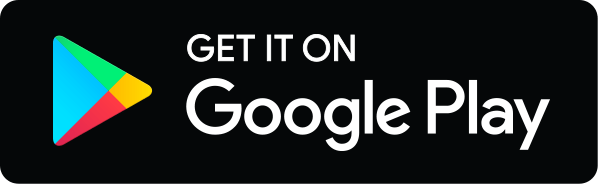