Case 10 A 50-year-old man was admitted with a mild left-sided weakness which had developed 40 minutes before presentation. Initial neurologic examination revealed a left-sided pronator drift during the arm pronation test. No vascular risk factors were known. During emergency CT he clinically deteriorated with progression to a high-grade hemiparesis with dysarthria (National Institutes of Health Stroke Scale [NIHSS] score: 9). Cerebral CT showed no early signs of ischemia (Alberta Stroke Program Early CT Score [ASPECTS]: 10) but perfusion CT revealed severe hypoperfusion in the right middle cerebral artery (MCA) territory with a marked reduction in cerebral blood flow (CBF), a mild reduction in cerebral blood volume (CBV), and a markedly prolonged mean transit time (MTT). CT angiogram (CTA) showed a right MCA occlusion in the distal M1-MCA segment (Fig. B10.1, Fig. B10.2, Fig. B10.3). Acute MCA ischemia caused by right distal M1-MCA occlusion of unknown origin. After exclusion of contraindications, thrombolysis with 75 mg intravenous recombinant tissue plasminogen activator (IV rt-PA) was commenced 1.5 hours after the onset of symptoms. (Performed at the same time that thrombolysis was initiated.) Color-coded imaging of the extracranial vessels showed no atherosclerotic vascular changes. Doppler spectrum analysis revealed no relevant differences in bilateral flow velocities or pulsatility (not shown). Color-mode insonation permitted visualization of only the proximal part of the right M1-MCA segment while left-sided insonation was normal. Reduced flow velocity and an increased pulsatility were seen in the right proximal M1-MCA segment (right MCA, 20/5 cm/s; left MCA, 95/25 cm/s) indicating distal MCA occlusion (Thrombolysis In Brain Ischemia [TIBI] grade 2 or 3, Consensus on Grading Intracranial Flow obstruction [COGIF] grade 3). Normal flow signals and velocities were seen in both A1-ACA segments (flow velocity: right, 110/45 cm/s; left, 100/40 cm/s) and the PCA on both sides (Fig. B10.4, Fig. B10.5, Fig. B10.6, Fig. B10.7; see also Video Right distal M1-MCA occlusion of unknown etiology. Fig. B10.8 shows a schematic drawing of the patient’s extra- and intracranial brain-supplying arteries. During thrombolysis the neurologic status of the patient improved steadily. At 70 minutes after initiation of rt-PA infusion, color-mode insonation showed a normalized M1-MCA segment, now visible over its total length. Doppler spectrum analysis revealed an improved flow (flow velocity 55/20 cm/s). In comparison with the initial transcranial color-coded duplex sonography (TCCS) findings, the flow velocity in the right A1-ACA segment had decreased (flow velocity 75/35 cm/s) indicating an initial leptomeningeal collateralization (Fig. B10.9 and Fig. B10.10; see also Videos B10.2). Partial M1-MCA recanalization after IV rt-PA infusion with signs of residual peripheral flow obstruction. Clinical improvement continued further until there was a mild residual left-sided hemiparesis (NIHSS score 3). Transesophageal echocardiography (TEE) shortly after intravenous thrombolysis showed two small floating structures adjacent to the aortic valve. Heparinization, aiming for a twofold rise in partial thromboplastin time (PTT), was initiated after 24 hours. Follow-up CT 1 day later showed a small cortical/subcortical infarction mainly in the right posterior insular region. No hemorrhagic transformation was seen (Fig. B10.11). Follow-up TEE after 4 days showed complete normalization. Negative blood cultures and absence of systemic infection signs made endocarditis unlikely. A transient cardiac thrombus was suspected and continuous oral anticoagulation with phenprocoumon was initiated. Cardioembolic occlusion of the right distal M1-MCA segment with recanalization during intravenous thrombolysis with rt-PA. Fig. B10.2 Perfusion CT, rCBF, rCBV and MTT maps, axial planes. Severe hypoperfusion in the right MCA territory. CBF/CBV mismatch indicating tissue at risk within the right MCA territory. (A) Decreased cerebral blood flow (CBF) (arrows). (B) Mildly reduced cerebral blood volume (CBV). (C) Delayed mean transit time (MTT). Fig. B10.3 Intracranial 3D CTA, axial maximal intensity projection (MIP). Occlusion of the distal right M1-MCA segment (arrowhead). Note the prominent visualization of the insular MCA branches caused by vasodilation. Fig. B10.4 TCCS (transtemporal approach), left-sided insonation, midbrain plane. Normal flow signal in the left M1-MCA (flow velocity 95/25 cm/s). Fig. B10.5 TCCS (transtemporal approach), right-sided insonation, midbrain plane. Reduced flow velocities (20/5 cm/s) and increased pulsatility in the proximal right M1-MCA indicating distal MCA occlusion (TIBI grade 2 or 3, COGIF grade 3). Fig. B10.6 TCCS (transtemporal approach), left-sided insonation, midbrain plane. Normal flow signal in the left A1-ACA (flow velocity 100/40 cm/s). Fig. B10.7 TCCS (transtemporal approach), right-sided insonation, midbrain plane. Normal flow signal in the right A1-ACA (flow velocity 110/45 cm/s). Here, we discuss a 50-year-old stroke patient who initially presented with a mild left-sided hemisyndrome, which progressed after 70 minutes to high-grade hemiparesis. He received IV rt-PA thrombolysis 90 minutes after symptom onset. The underlying cause was a cardiac embolism. Our patient’s early clinical deterioration was rapidly assumed to be caused by clot disruption and distal fragmentation. Neurologic worsening in acute ischemic stroke is poorly defined. Analyzing 1964 consecutive patients admitted within 4 hours of onset, 256 patients (13%) had a worsening after 48–72 hours defined by an increase of at least 1 point on the NIHSS. Neurologic worsening was considered a progressive stroke in 33.6% of cases, increased intracranial pressure in 27.3%, recurrent ischemia in 11.3%, and secondary parenchymal hemorrhage in 10.5%. ICA or M1-MCA occlusion, territorial infarction, brainstem infarction, and diabetes mellitus were identified as independent predictors (Weimar et al 2005). In the earliest phase of acute stroke increased intracranial pressure can almost be ruled out, but in the case of rt-PA treatment urgent CT is required. If bleeding is excluded, a clot fragmentation and secondary distal vessel occlusion, progressive in-situ atherothrombotic occlusion of a main-stem vessel or orifice of a perforator artery, or a hemodynamically caused hypoperfusion by arterial hypotension or cardiac decompensation can be assumed. Also, acute secondary anemia may lead to penumbral failure and extension of ischemic territory (Bösel et al 2005). Immediate vessel assessment is required to select the best therapeutic strategies. Fig. B10.8 Schematic of the patient’s extra-and intracranial brain-supplying arteries. Note the right distal M1-MCA occlusion (circle). Leptomeningeal collateralization of the right MCA territory via the right ACA (red arrow). Fig. B10.9 TCCS (transtemporal approach), right-sided insonation, midbrain plane. Color-mode delineation of the complete M1-MCA without discontinuation. Ameliorated flow signal with a flow velocity of 55/20 cm/s. Fig. B10.10 TCCS (transtemporal approach), right-sided insonation, midbrain plane. Right A1-ACA after thrombolysis with partial normalization of flow (flow velocity 75/35 cm/s) indicating regression of initial flow increase feeding leptomeningeal collaterals. Fig. B10.11 Unenhanced cranial CT, axial plane. Follow-up CT after 1 day: Small cortical/subcortical infarction mainly in the right posterior insular region (arrows). Since the results of the NINDS (National Institute of Neurological Disorders and Stroke) study were published in 1995 and U.S. Food and Drug Administration (FDA) approval was gained in 1996, IV rt-PA thrombolysis with a total dose of 0.9 mg/kg delivered 10% as bolus and the remainder as an infusion over 60 minutes has been the mainstay of therapy in acute stroke. It is used worldwide and has enormously promoted the founding of stroke units. The NINDS trial was a randomized placebo-controlled study including 624 patients treated by IV rt-PA commenced in the first 3 hours after onset of symptoms. Patients’ outcomes at 3 months compared with controls were clearly better despite a higher frequency of symptomatic intracranial bleedings under IV rt-PA treatment versus controls (6.4% versus 0.6%) (NINDS rt-PA Stroke Study Group 1995). Three other studies, the European Cooperative Acute Stroke Study ECASS-I and ECASS-II, with a time window extended to 6 hours, and the Alteplase Thrombolysis for Acute Stroke Noninterventional Therapy in Ischemic Stroke (ATLANTIS) with inclusion of patients between 3 and 5 hours failed to improve clinical outcome (Clark et al 1999, Hacke et al 1995, 1998). Pooling results from these studies using meta-analyses, however, confirmed the positive results of the NINDS study (Gonzales et al 2006, Hacke et al 1999, Hacke et al 2004, Wardlaw et al 2002). The Safe Implementation of Thrombolysis in Stroke Monitoring Study (SITS-MOST), a European observational study in 6,483 patients from 14 countries, showed that results comparable to the NINDS trial can be achieved even if centers with little experience in thrombolysis are included (Wahlgren et al 2007). ECASS III, published in 2008, included 821 patients and showed that the time window of systemic thrombolysis can be extended up to 4.5 hours. Despite a higher intracranial bleeding rate in the IV rt-PA treated cohort (27% versus 17.6%) and symptomatic intracranial hemorrhages (2.4% versus 0.2%) a modified Rankin scale (m-RS) of 0 or 1 was achieved in 52.4% of treated cases compared with 45.2% patients in the placebo group (Hacke et al 2008). Again, the positive effect within the first 4.5 hours was also confirmed in a meta-analysis including 6,756 patients of all published randomized controlled thrombolysis trials (Emberson et al 2014). Despite its proven effectiveness, the use of IV rt-PA thrombolysis remains limited. Initial surveys reported thrombolysis treatment rates for acute stroke patients of 2% in general hospitals and 5% in hospitals with an available stroke unit. Today, most hospitals reach treatment rates of up to 10% and single centers report treatment rates ranking up to 20% (Heuschmann et al 2004, Singer et al 2012) leaving a high proportion of patients without causal treatment options. The major reasons for this were delayed admission, unknown time of symptom onset, minor symptoms, clinical improvement, uncertainty regarding diagnosis, and fear of bleeding complications. Successful recanalization during systemic thrombolysis depends on the location, length, and composition of the clot (i.e., embolus or thrombus). More distally located clots had the highest recanalization rate in anterior circulation stroke when analyzed with TCD. Complete recanalization occurred in 44.2% of distal MCA occlusions (50 of 113), in 30% of proximal MCA occlusions (49 of 163), and only in 5.9% of terminal ICA occlusions (1 of 17) (Saqqur et al 2007b). Analyzing clot length in 138 patients with acute MCA occlusion undergoing systemic thrombolysis with CT (slice thickness of 2.5 mm) showed successful recanalization in 62 patients in whom the clot length was <8 mm. The median m-RS at hospital discharge was 2. In the remaining 76 patients without recanalization, the clot length was mostly >8 mm. Here patients were discharged with a median m-RS score of 5 (Riedel et al 2011). At least in a rat model, platelet-rich thrombi seemed more resistant to thrombolysis than fibrin-rich clots (Tomkins et al 2015). The insufficient recanalization rates in proximal and extended occlusions subsequently resulted in intensified clinical use of intra-arterial therapeutic approaches, which had been tested (e.g., in basilar artery (BA) occlusion) even before the establishment of systemic thrombolysis (Zeumer et al 1983). A first approach was the intra-arterial (IA) administration of thrombolytic drug, resulting in higher local concentration and reduced systemic effects, assumed to be more effective and safe. However, the high technical requirements and the procedural delay of treatment onset restricted its use to specialized centers. In the PROACT II (Prolyse in Acute Cerebral Thromboembolism) study, IA urokinase was given within 6 hours of stroke onset. In comparison with heparin alone, substantially better recanalization rates of TICI (Thrombolysis in Cerebral Infarction) 2–3 of 66% versus 18% and a better 90-day clinical outcome were observed (Furlan et al 1999). These results were further confirmed by a meta-analysis of randomized trials published in 2010. There, IA thrombolysis with urokinase or recombinant prourokinase within 6 hours of stroke onset resulted in a better clinical outcome at 3 months compared with systemic thrombolysis. However, at the same time a significant increase of symptomatic intracranial hemorrhages within 24 hours after treatment onset was observed. Despite these results, prourokinase has not yet achieved FDA approval (O’Rourke et al 2010). Although not substantiated by positive clinical trials, several specialized centers practiced a combination of IV thrombolysis with IA drug-induced or mechanical thrombolysis (thrombectomy), the so-called “bridging” technique. In 2013, however, three randomized studies were published with negative results. The SYNTHESIS (Local Versus Systemic Thrombolysis for Acute Ischemic Stroke) expansion study included 362 patients with stroke onset within 4.5 hours. One-half of the patients received systemic thrombolysis, the other half received endovascular treatment with IA rt-PA administration, mechanical clot disruption or retrieval (SOLITAIRE, PENUMBRA, TREVO or MERCI device), or a combination of both. Neither intracranial hemorrhage, death rate, nor clinical outcome significantly differed across groups. Notably, endovascular therapy started, on average, 1 hour later than systemic thrombolysis (Ciccone et al 2013). A study with a similar design, the IMS-III (International Management of Stroke) trial, was the largest randomized trial and included 656 patients. The published results could not prove superiority of endovascular stroke therapy in general but subgroup analysis indicated a benefit for patients with carotid-T or tandem ICA-MCA occlusion despite the fact that the majority of patients in the endovascular arm were treated with IA thrombolysis alone and not with a retriever, suction device, or stent retriever (only used in 5 patients). Interestingly, documentation of large-vessel occlusion was not required for randomization (Broderick et al 2013). The MR RESCUE (Mechanical Retrieval and Recanalization of Stroke Clots Using Embolectomy) study tried to stratify if patients had a “favorable penumbra” pattern (small infarct core and relevant “tissue at risk”) assessed by CCT or MRI, to determine who would particularly benefit from endovascular therapy. Patients with a large-vessel occlusion of the anterior circulation received either endovascular therapy (MERCI retriever or PENUMBRA device for suction embolectomy) or standard therapy within 8 hours of symptom onset. The study could not prove superiority of embolectomy and also showed that the penumbra pattern was not helpful for identifying patient groups with specific therapeutic benefit (Kidwell et al 2013). All three studies (SYNTHESIS, IMS-III, and MR RESCUE) confirmed at least comparable safety profiles of systemic thrombolysis and endovascular therapy concerning the rates of intracranial hemorrhages, despite not having observed any outcome differences. Long time windows between symptom onset and therapy, the undifferentiated selection of patients, and the use of first- and second-generation devices were considered possible explanations for the unsatisfactory results (Qureshi et al 2014). Based upon experience from the aforementioned trials, new studies were conducted with improved stent retriever devices and improved patient selection based on non-contrast CT (ASPECTS criteria), CT angiography, and CT perfusion in documented large-vessel occlusion (for technical aspects, see also Chapter 6, “Technical Aspects of Mechanical Thrombectomy” under “Digital Subtraction Angiography”). A significant clinical benefit of mechanical thrombectomy compared with intravenous thrombolysis alone in acute proximal intracranial occlusion of the anterior circulation was proved for the first time in five randomized studies including 1,287 patients, all published in 2015. The MR CLEAN study (Multicenter Randomized Clinical trial of Endovascular Treatment in the Netherlands) conducted in 16 centers with a total of 500 patients was the first to demonstrate a benefit for patients with endovascular treatment within 6 hours of stroke onset in proximal vessel occlusions of the distal ICA, M1-MCA, M2-MCA, and A1-ACA. Treatment was performed in addition to a systemic thrombolysis within the 4.5-hour time window. In this study 81.1% in the intervention arm and 90.6% in the control arm received IV rt-PA. Systemic thrombolysis was initiated by 85–87 minutes after symptom onset in both treatment groups. The time window from onset to groin puncture was 260 minutes. In the interventional group (initial median NIHSS score of 17) 32.6% of patients achieved functional independence (m-RS 0–2) after 90 days as compared with 19.1% (initial median NIHSS score of 18) in the IV thrombolysis group. Complete recanalization, defined as Thrombolysis In Cerebral Infarction (TICI) score 2b-3 (Higashida et al 2003), was seen in 58.7% of IA-treated patients. Recanalized vessel occlusion on follow-up CTA was seen in 75.4% in the intervention group and 32.9% in the medical arm. Accordingly, final infarct volume after 1 week was 49 mL versus 79 mL. Intracranial hemorrhage rates (7.7% versus 6.4%) and mortality rates at 30 days (18.9% versus 18.4%) were not different (Berkhemer et al 2015). Shortly thereafter, two studies (ESCAPE and EXTEND-IA) confirmed the results of the MR CLEAN trial. The ESCAPE trial (Endovascular treatment for Small Core and Anterior circulation Proximal occlusion with Emphasis on minimizing CT to recanalization times), conducted in 22 centers in Canada, the United States, and Europe, was stopped prematurely after a positive interim analysis of 315 included patients. In the trial, patients were included with a NIHSS score ≥6, occlusion of carotid-T or MCA (M1 or long segment of the M2), and good collaterals with symptom onset within 12 hours. Similar NIHSS and rate of IV rt-PA was gained in the intervention arm and control group (16 versus 17; 72.9% versus 78.6%). Median time from imaging to groin puncture was 51 minutes. A good clinical outcome (m-RS score 0–2) was achieved in 53% of patients in the interventional group as compared with 29.3% of patients in the control group (number needed to treat [NNT] to have one additional patient independent at 90 days = 4). Of note, subgroups of older patients and patients treated after more than 6 hours also benefited from the intervention (Goyal et al 2015). The Australian EXTEND-IA trial (EXtending the time for Thrombolysis in Emergency Neurological Deficits with Intra-Arterial therapy) was also halted after an interim analysis of 70 randomized patients; originally 1,044 patients had been screened. The study had included patients with an occlusion of the intracranial ICA or the M1-or M2-MCA who received systemic thrombolysis within the 4.5-hour time window. In addition, a significant mismatch in CT or MRI perfusion and a treatment initiation within the first 6 hours were required. The mean NIHSS was 17 in the intervention group and 13 in the control group. The time delay between stroke onset and groin puncture was 210 minutes and the Solitaire stent retriever was used for the procedure. Reperfusion of ischemic territory at 24 hours was greater in the endovascular arm than in the alteplase-only arm (median, 100% versus 37%), and an m-RS of 0–2 was achieved in 71% and 40%, respectively. No differences between treatment groups were found concerning death or intracranial hemorrhage (Campbell et al 2015). Other recently published studies (REVASCAT, SWIFT-PRIME) confirm the overwhelming data now available with NNTs of four and six, respectively (Jovin et al 2015, Saver et al 2015). Mechanical thrombectomy is expected to change therapeutic strategies in acute anterior stroke treatment, much as the introduction of IV rt-PA did in 1996. Further improvements can be expected by refinements in the techniques of embolectomy, stent-retrievers, and distal and proximal aspiration devices (Jauch et al 2013), patient selection, and minimization of time delays. The entire infrastructure of stroke care has to be reorganized to assure mechanical recanalization therapy as early as possible. Based on the current data, it seems that a treatment start within up to 347 minutes will result in superiority of the endovascular approach, resulting in a currently recommended time window for mechanical thrombectomy of 6 hours from symptom onset (Vagal et al 2014). Accordingly, the European Stroke Organization (ESO) has already changed its consensus guidelines: Mechanical thrombectomy, in addition to intravenous thrombolysis within 4.5 hours when eligible, is recommended to treat acute stroke patients with large artery occlusions in the anterior circulation up to 6 hours after symptom onset (Grade A, Level 1a). In addition, if intravenous thrombolysis is contraindicated (e.g., the patient is warfarin-treated with therapeutic INR) mechanical thrombectomy is recommended as first-line treatment in large-vessel occlusion (Grade A, Level 1a). A benefit may even be achieved in an 8-hour time window in appropriate patients (Jovin et al 2015). By analogy to the thrombolysis registers, as many patients with mechanical recanalization as possible should be included into a prospective register to gather more evidence from everyday practice and to prove the transferability of study results into clinical routine. The next step will also be to provide proof of concept for mechanical recanalization in vertebrobasilar vessel occlusion. Apart from its use for diagnostic purposes, ultrasound has been shown to have therapeutic potential. Ultrasound is able to cause changes within the fibrin structure of a thrombus by inducing plasma “microstreams.” This subsequently leads to better penetration and action of rt-PA. Both mechanisms accelerate thrombolysis. In 2004, the results of the CLOTBUST (Combined Lysis of Thrombus in Brain Ischemia with Transcranial Ultrasound and Systemic TPA) study were published, which included 126 patients with acute proximal or distal MCA occlusion. The patients were randomized to either rt-PA treatment alone or a combination of IV rt-PA and continuous TCD with a diagnostic 2-MHz transducer over 2 hours. Patients treated with the combined approach had a much higher rate of early recanalization within 2 hours (46% versus 18%) and a trend toward a distinct clinical improvement (29% versus 21%). The trend persisted after 3 months. The study was under-powered, however, to identify a statistically significant difference (Alexandrov et al 2004). A single-center TCCS study in 37 patients with MCA main-stem occlusion also found a higher recanalization rate if continuous ultrasound was applied over 1 hour (46% versus 21%, respectively). However, the number of patients was again too small for a sensitive statistical analysis (Eggers et al 2003). To improve ultrasound penetration and therefore the thrombolytic potency of ultrasound, the effects of low-frequency ultrasound (300 kHz) were analyzed in acute stroke patients undergoing intravenous thrombolysis. Unfortunately, this approach resulted in a substantially higher number of intracranial hemorrhages and the study had to be terminated early (Daffertshofer et al 2005). A meta-analysis of six randomized and three nonrandomized clinical sonothrombolysis studies showed that insonation with diagnostic frequencies increased the probability of recanalization threefold and the rate of a better clinical outcome twofold. Rates of symptomatic intracranial hemorrhages did not significantly differ between standard and sonothrombolysis therapies (Tsivgoulis et al 2010). Two other recent meta-analyses concluded that sonothrombolysis reduces 3-month deaths and dependency rates and increases recanalization rates, but does not increase the rate of symptomatic intracranial hemorrhages (Ricci et al 2012, Saqqur et al 2014). Another potential approach that could enhance ultrasound-induced thrombolysis is the use of air- or gasfilled microbubbles—i.e., ultrasound contrast agents. A small study showed a positive effect using combined TCD ultrasound, IV rt-PA, and microbubbles (Molina et al 2006). A continuative controlled phase IIb TUCSON trial (Transcranial Ultrasound in Clinical SONothrombolysis) reported that a 1.4-mL dose of perflutren-lipid microbubbles did not increase the risk of symptomatic intracranial hemorrhage and could be safely applied during IV rt-PA administration. Using this approach, recanalization rates reached 50–67% (Barreto et al 2009, Molina et al 2009). Finally, sonothrombolysis has been proposed as a monotherapy, i.e., without additional thrombolytic medications. This is of particular interest specifically as a large proportion of patients are denied IV rt-PA due to contraindications. A pilot study that included patients with MCA main-stem occlusion within a 6-hour time window reported recanalization in 62.5% of patients who underwent 1 hour of continuous TCCS and no recanalization in the noninterventional group (Eggers et al 2005). One limitation for the widespread use of sonothrombolysis may be the lack of a sufficient temporal bone window for MCA insonation as the main target vessel. In a study of 179 patients (42% female), 39% had an M1- or M2-MCA occlusion. Overall, 23% had inadequate insonation conditions. Remarkably, a comparable number (18%) were ineligible for CTA or MRA diagnostic evaluation because of contraindications or severe motion artifacts (Nolte et al 2013). A second important limitation is the operator dependency in ultrasound examinations. As the availability of experienced sonographers in the emergency department is currently limited to a few centers (Tsivgoulis and Alexandrov 2008), the development of an investigator-independent “hands-free” 2-MHz trans cranial ultrasound device seems an attractive option. Following promising results from smaller phase IIa/IIb studies, a hands-free device was studied in the randomized controlled multi-center CLOTBUT-ER trial (Barlinn et al 2013, Barreto et al 2013, Schellinger et al 2015). Currently, the selection of patients for systemic thrombolysis is based solely on the exclusion of intracerebral hemorrhage using CCT. Knowledge of the vascular status or the tissue at risk is not required to make a therapeutic decision. In our patient CTA revealed a distal M1-MCA occlusion without a hyperdense MCA sign on plain CT. Interestingly, a hyperdense MCA sign was observed in one-half of 20 cases with M1-MCA occlusion. Histopatho-logically, a hyperdense vessel segment reflects clots composed of mainly red blood cells (red thrombi) whereas fibrin-dominant clots (white thrombi) are less detectable (or not at all) on CT. Large overlaps exist, however, in pathologic findings which make simple interpretation of data difficult (Liebeskind et al 2011b). A further study distinguished between the origins of emboli. No differences in the age of thrombi were observed. Emboli that originated in arteries, i.e., artery-to-artery clots, had the highest percentages of red blood cells, whereas the cardioembolism and unknown subtypes had the lowest percentage of red blood cells. Importantly, the proportions of both platelets and fibrin proportion were similar across origin subtypes (Niesten et al 2014). Not all patients with clinical stroke symptoms have a major brain-supplying artery occlusion, or even an occlusion that could potentially be reopened by thrombolysis. This patient group, however, is exposed to the 5–10% risk of an intracranial bleed. A major argument against an extended vascular diagnosis has been the time factor. But with the increasing availability of multimodal CT, MRI, and ultrasound techniques, which permit a fast and reliable diagnosis of vessel occlusions, the vascular status can now be obtained without significant delay. This in turn will help to stratify patients early, and subsequently allow for the administration of individualized treatment strategies, likely leading to better clinical outcomes. Perfusion and diffusion-weighted MRI allows brain tissue that is functionally but not yet structurally impaired (penumbra) to be visualized, defining the penumbra as the difference between the perfusion MRI and diffusion MRI. From this technique the so-called “mismatch concept” was developed and has been successfully used to select patients for thrombolysis and to prolong the time window of IV rt-PA treatment to up to 6 hours and longer in selected cases (Hacke et al 2009). A similar approach is followed in current CT perfusion studies. The CT technique has a particular logistic advantage as CT access for emergency patients is usually easy to obtain. Using CT, the ischemic penumbra or “tissue at risk” can be defined as the difference between the CBF (analogous to perfusion MRI) and the CBV (analogous to diffusion MRI). A comparative study in 42 stroke patients showed that tissue at risk, as determined by perfusion CT and CTA, was equivalent to the MRI results in all but one case (Wintermark et al 2007). Moreover, recent studies support the suitability of perfusion CT for stroke detection (Campbell et al 2015, Hana et al 2014) including a meta-analysis that confirmed perfusion CT as having a high sensitivity and a very high specificity for detecting infarcts (Biesbroek et al 2013). Finally, ultrasound perfusion tests have also been performed in acute stroke patients. Ultrasound follows the same basic approach as MRI and CT, i.e., it analyzes the perfusion kinetics of a contrast bolus within the micro-circulation. However, for several reasons, this technique is currently still experimental and rather limited (Eyding et al 2006, Kern et al 2011, Meairs and Kern 2015, Seidel et al 2013). Digital subtraction angiography (DSA) remains the reference method for the evaluation of intracranial occlusions. In clinical practice, multislice CTA, yielding similar results, is being increasingly used. In BA pathology, CTA sensitivity is even higher as it has been shown to detect distal BA near-occlusions that were considered complete occlusion using DSA. After correction for false-positive BA occlusions, sensitivity, specificity, and positive and negative predictive values for the detection of intracranial occlusions of the major arteries by CTA were all 100%. Respective values for time-of-flight (TOF)-MRA in the same patient group were 87% sensitivity, 98% specificity, 59% positive predictive value, and 99.5% negative predictive value (Bash et al 2005). When analyzing more distal artery occlusions the limitation of TOF-MRA becomes more evident. Using contrast-enhanced MRA 20% of main-stem arteries or their branches were patent arteries, which had been interpreted as occluded using TOF-MRA (Yang et al 2002). The spatial resolution of TOF-MRA can be improved if 3-T and sensitivity-encoding techniques are combined, which has been shown to improve diagnostic results. Compared with DSA, sensitivity, specificity, and positive and negative predictive values of 100%, 99%, 87%, and 100% were reported but the authors included only MCA and ICA occlusions (Choi et al 2007). CT and MRI can mainly be used as “single shot” methods. They are too laborious, too expensive, or too stressful for the patient to be used for continuous monitoring. In comparison, ultrasound has the advantage of permitting serial as well as continuous measurements without these restrictions. In the hands of an experienced sonographer, TCD may allow diagnosis of main-stem occlusion, at least for the main stem of the MCA. In acute stroke patients, however, TCCS is the superior method provided that the transcranial bone window is sufficient or signals are enhanced by contrast agents. A proximal MCA occlusion can be diagnosed simply if the color signal and Doppler spectrum in projection of the artery in question are absent. In a small study (10 stroke patients with a main-stem MCA occlusion diagnosed using TOF-MRA), TCCS confirmed the diagnosis of a main-stem MCA occlusion in all patients (Kenton et al 1997). In a second study 20 of the 23 patients who had vascular pathology and underwent contrast-enhanced TCCS were correctly diagnosed, whereas TCD and unenhanced TCCS were used successfully only in 14 and 7 patients (Goertler et al 1998). In hyperacute stroke, the diagnostic accuracy of TCCS in comparison to CTA and MRA was analyzed in 58 stroke patients within the first 6 hours of stroke onset by unenhanced and, if necessary, subsequent contrast-enhanced extra- and intracranial duplex ultrasound. Examinations were performed before the initiation of other diagnostics while patients were still in the emergency department. Echo contrast agent use was considered necessary in 51 patients. The mean duration of the complete examination was 21.3 minutes in the combined native and contrast-enhanced examination and 13.6 minutes if native examination was performed alone. Ultrasound findings were confirmed using DSA in 31 of 32 patients (Gerriets et al 2002). Shorter examination times could probably be achieved if contrast agents were administered at the beginning of the ultra-sound examination. In an own clinical ultrasound study we analyzed the time needed for the duplexsonographic assessment of all extra- and intracranial arteries (extra-cranial ICA and VA, intracranial MCA, ACA, PCA, V4-VA, and BA) if reduced items were assessed (vessel status: open, occluded, stenosis >50%, distal stenosis/occlusion) and if intravenous echo contrast (SonoVue) was initially used. Analysis of 126 patients revealed a mean insonation time of ~6 minutes with a range of 4–12 minutes (Schreiber et al 2010). Detection of a single M2-MCA can be considered if only one M2-MCA branch is visualized despite good insonation conditions and the patient has a corresponding ischemia. M3-MCA branch occlusions are usually not detectable because of the unknown number of branches occluded in the individual patient. A relevant distal occlusion, however, can be considered if a substantial difference in blood flow velocity is present between the right and left sides (see also the asymmetry index of Zanette, Case 13). Proximal occlusions may also result in detectable indirect signs: for example, an M1-MCA occlusion usually leads to raised flow velocities in the ipsi-lateral ACA and PCA, indicating their function as feeders of leptomeningeal collateral vessels, a phenomenon also called flow diversion. In M2-MCA occlusion the remaining M2-MCA branch might also show high velocities because of leptomeningeal collateral flow within the MCA itself (for further reading see also Fig. A5.134). It can, however, be difficult to differentiate collateral flow from additional stenosis, or to confirm the presence of both. Comparable to the hyperdense MCA sign on plain CT, a hyperechoic vessel sign was described using TCCS in four patients with occluded MCA main stem within 6 hours of symptom onset (Kadimi et al 2000). In good insonation conditions, the deep middle cerebral vein is seen in low flow velocity settings in the lateral fissure without concomitant MCA, which also indicates proximal MCA occlusion. Visualization of a venous vessel without the paralleling artery may affirm PCA occlusion as well if only the accompanying basal vein of Rosenthal is detected. Extracranially, the presence of the vertebral vein can be used as an indication of VA occlusion. TCCS also allows assessment of vessel recanalization. In patients receiving IV rt-PA, recanalization of MCA main-stem occlusion was seen in 50% after 2 hours and in 75% after 24 hours. In conservatively treated patients the same study found no recanalization within 2 hours and in only 8% after 24 hours (Gerriets et al 2000). Acute MCA occlusions differ from chronic occlusions in that acute changes result in rapid changes of findings over a short period. Occlusions might be incomplete, resulting in subsequent flow normalization, and reocclusion might be seen within a few minutes of continuous observation. To evaluate the different types of observable flow profiles, the TIBI grading system which is based on TCD and ranges from 0 to 5 has been introduced to characterize flow in MCA and BA strokes (Demchuk et al 2001). Later, the COGIF classification was introduced by groups preferentially using TCCS, which follows similar principles to the TIBI graduation (Nedelmann et al 2009b). For further reading see also Chapter 5, “Occlusions” under “Intracranial Pathology”). Both grading systems allow a first step in the description of the dynamic processes that occur during recanalization. Flow velocity differences between the right and left sides can furthermore be analyzed and described by several asymmetry indices (see also Case 13). Initial application of the TIBI criteria in acute stroke patients revealed valuable information. It was shown that recanalization of embolic occlusions follows different temporal patterns. According to Alexandrov and coworkers (2001), embolic occlusions can be divided into three main recanalization types: those that reopen within 1 minute of rt-PA treatment initiation; those that need 1–29 minutes, demonstrating stepwise recanalization; and those that need more than 30 minutes. Correlation with stroke etiology showed that fast recanalization occurs in 59% of cardioembolic MCA occlusions but in only 8% of atherothrombotic artery-to-artery occlusions. Fast recanalization in patients with stroke of undetermined origin occurred in 50% of cases, suggesting that most of these might actually be of cardioembolic origin (Molina et al 2004). Furthermore, the TIBI grades can be used for early assessment of stroke prognosis. A low initial TIBI grade correlates significantly with a bad clinical outcome and a higher mortality (Demchuk et al 2001, Tsivgoulis et al 2013). Sudden recanalization has a better clinical prognosis. A residual flow, often not distinguished from occlusion using TOF-MRA, predicts early recanalization (Alexandrov et al 2001). TCCS assessment of recanalization in the multicenter Neurosonology in Acute Ischemic Stroke (NAIS) study (361 patients) confirmed that a persisting MCA main-stem occlusion 6 hours after onset of symptoms was an independent predictor for poor clinical outcome. After 3 months, 88% of these patients died or were dependent, whereas patients with distal branch occlusions or normal ultrasound findings had good clinical outcomes in 50% and 63%, respectively (Allendoerfer et al 2006). The assessment of collateral flow, i.e., the ACA and PCA flow in MCA occlusion, may further help to evaluate prognosis. As expected, patients with good collateral flow in persisting MCA occlusion have a better clinical outcome (Y.S. Kim et al 2006). Finally, continuous monitoring helps us understand secondary clinical worsening that may be observed in acute stroke patients after primary recanalization. A systematic analysis of 374 patients undergoing systemic thrombolysis reported an early reocclusion in 14% of cases, which was mostly strongly associated with clinical worsening (Saqqur et al 2007a).
Thrombolysis of M1 Middle Cerebral Artery Occlusion
Clinical Presentation
Initial Neuroradiologic Findings
Suspected Diagnosis
Clinical Course (1)
Questions to Answer by Ultrasound Techniques
Initial Neurosonologic Findings
Extracranial Duplex Sonography
Transcranial Duplex Sonography
B10.1).
Conclusion
Clinical Course (2)
Follow-up Neurosonologic Findings (1 Hour)
Transcranial Duplex Sonography
Conclusion
Clinical Course (3)
Final Diagnosis
Discussion
Clinical Aspects
Sonothrombolysis
Angiologic and Anatomic Aspects
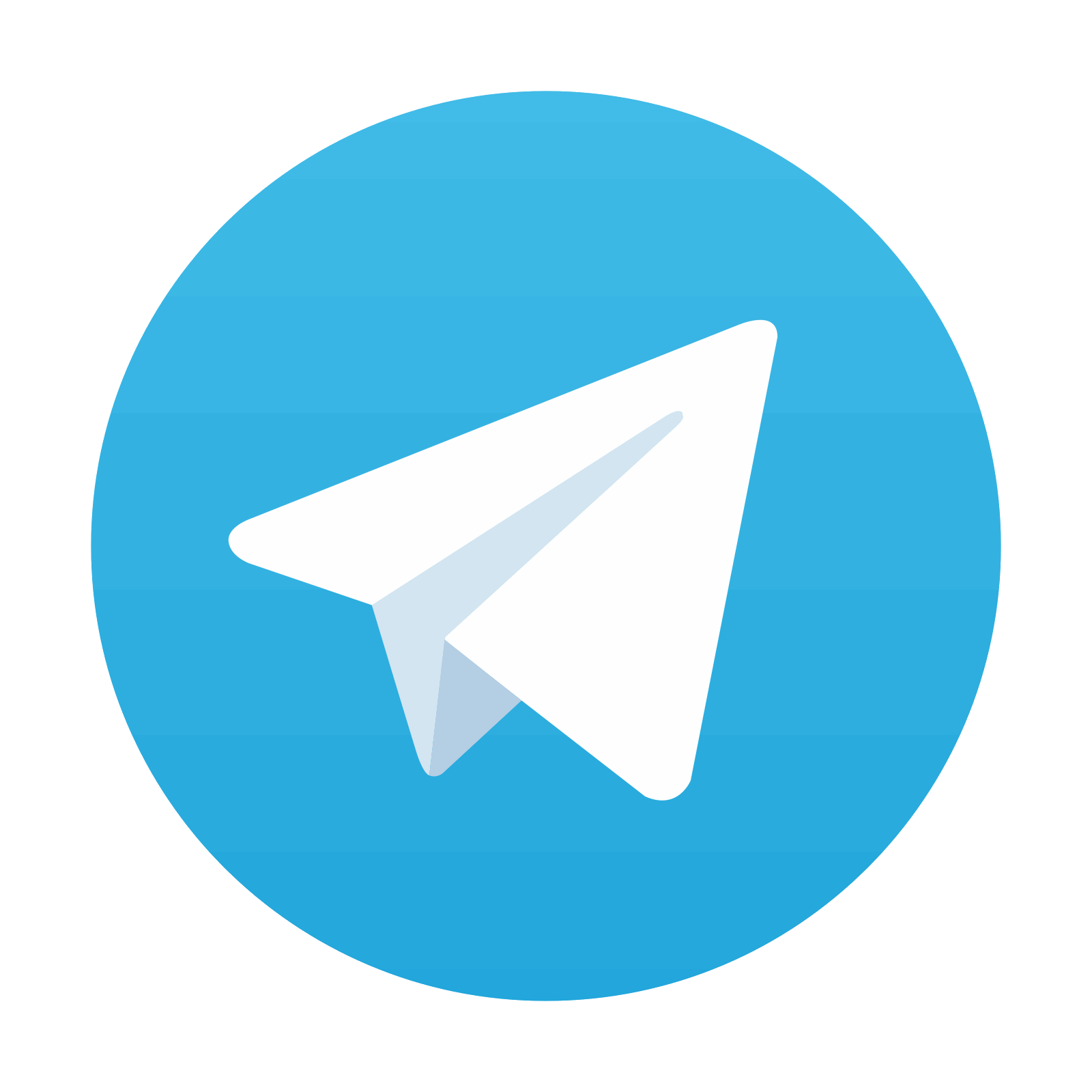
Stay updated, free articles. Join our Telegram channel

Full access? Get Clinical Tree
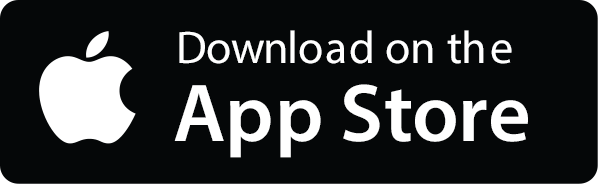
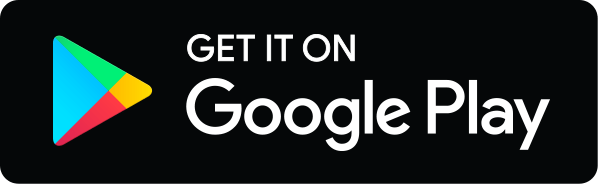