Case 43 A 4-year-old boy with known homozygous sickle cell disease (SCD) presented for the first time at our neurosonology laboratory for a screening examination of his basal cerebral arteries. At that time, he was free of neurologic symptoms and his medical history was unremarkable. No vessel wall pathology was identified. High flow velocities were detected in both ICA (left: 224/82 cm/s, time-averaged maximum velocity = Vmean 128 cm/s; right: 201/79 cm/s, Vmean 117 cm/s). Flow parameters in the vertebral arteries (VAs) were normal (not shown). Intracranially, high flow velocities were found in both M1-MCA (left: 239/106 cm/s, Vmean 156 cm/s; right: 236/79 cm/s, Vmean 136 cm/s). Flow velocities were also increased in the left M2-MCA (206/84 cm/s, Vmean125 cm/s) and the right A1-ACA (211/80 cm/s, Vmean 124 cm/s) (Fig. B43.1, Fig. B43.2, Fig. B43.3, Fig. B43.4). All other intracranial arteries (left ACA, both ICA, PCA, VA, and basilar artery (BA) as well as both ophthalmic arteries (OAs) showed flow velocities within the normal range for children with SCD (see “Angiologic and Anatomic Aspects” below). Resistance and pulsatility indices were normal in all intracranial and intraorbital arteries. No poststenotic flow patterns and no signs of collateral flow were found in any vessel. Normal Lindegaard (MCA/ICA index) regarding peak systolic flow velocity ratio was measured (right 1.1, left 1.2). According to the ultrasound criteria for children with SCD, slightly increased flow velocities were observed in both MCAs and the right ACA, mainly caused by a bilateral cerebral hyperperfusion of the anterior circulation. Regular extracranial and transcranial duplex examinations during the following 4 years showed constant findings. The patient suffered from recurrent SCD crises with bouts of thoracic and multifocal osseous pain and pulmonary infections with respiratory insufficiency. Also, severe anemia with low hematocrit (0.19–0.25 L/L) and hemoglobin (6–8 g/dL) values and sickle cell hemoglobin (HbS) of ~90% was finally observed. Because hydroxyurea treatment proved ineffective, repeated transfusion therapy was performed and, later, a bone marrow transplant was conducted. No neurologic symptoms developed during the entire observational period. Cranial MRI performed 3 years after the initial presentation in our department and before receiving the first blood transfusion revealed no signs of cerebral ischemia. Time-off-light MR angiography (TOF-MRA) showed no clear intracranial stenosis of the basal cerebral arteries (Fig. B43.5). Normal flow velocities were observed in all extracranial and intracranial arteries and no signs of cerebral hyperperfusion were found (not shown). Fig. B43.1 TCCS (transtemporal approach), left-sided insonation, axial midbrain plane. Left M1-MCA segment with increased flow velocity (239/106 cm/s, Vmean 156 cm/s). Fig. B43.2 TCCS (transtemporal approach), right-sided insonation, axial midbrain plane. Right M1-MCA segment with increased flow velocity (236/79 cm/s, Vmean 136 cm/s). Fig. B43.3 TCCS (transtemporal approach), left-sided insonation, axial midbrain plane. Left M2-MCA segment with increased flow velocity (206/84 cm/s, Vmean 125 cm/s). Fig. B43.4 TCCS (transtemporal approach), right-sided insonation, axial midbrain plane. Right A1-MCA segment with increased flow velocity (211/80 cm/s, Vmean 124 cm/s). Unremarkable ultrasound findings in the brain-supplying arteries comparable to healthy children without anemia after bone marrow transplantation. SCD is a hereditary disorder in the spectrum of hemoglobinopathies of hemolytic anemias. The heredity is auto-somal recessive with a point mutation on chromosome 11 leading to an abnormal hemoglobin, sickle cell hemoglobin (HbS), in erythrocytes. HbS tends to form pathologic aggregates in the deoxygenated state with hemolysis. SCD is a common hematologic disorder in countries in which malaria is a relevant infectious disorder. The highest prevalence of SCD is found in up to 40% of the population in countries close to the equator, especially in Africa and Asia. These patients have a relative resistance against malaria, explaining the relevant selection advantage. Fig. B43.5 Top: MRI, T2-weighted (left) and FLAIR-weighted (right) images, axial plane revealing unremarkable brain parenchyma without signs of atrophy or cerebral ischemia. Bottom: 3D TOF-MRA, coronal maximal intensity projection (MIP). The vessel diameter of all visible basal cerebral arteries appeared normal. Patients, especially children, with SCD are at high risk of stroke. Stroke occurs in ~10% of patients with SCD under 20 years of age and is most common between the ages of 4 and 15 years (Platt 2005). The annual stroke rate in unselected children is 0.5–1% (Ohene-Frempong et al 1998), which increases up to 3% if silent ischemia is detected on MRI (Miller et al 2001). About 12% of asymptomatic SCD children reveal pathologic flow velocities suggestive of significant arterial stenosis (Adams et al 1992a). Children with time-averaged maximum velocities = mean flow velocities (Vmean) >200 cm/s in the anterior circulation have an annual stroke risk of ~10% which decreases to <1% under regular transfusion therapy (Adams et al 1998). The pathophysiologic mechanisms of stroke in SCD are still not completely understood but two different lesion patterns affecting (1) small intracranial vessels and (2) large intracranial vessels can be distinguished. Considering the microcirculation, deoxygenation of the HbS leads to its polymerization and distortion of the erythrocytes. The disfiguration of the red blood cells is initially reversible but tends to become permanent (Bunn 1997). The adherence of sickle erythrocytes to the endothelium is enhanced by von Willebrand protein and procoagulant characteristics of their membranes (Kaul et al 1993). These mechanisms reduce the ability of erythrocytes to traverse the microcirculation and may result in intracerebral microvascular occlusions and ischemia. Large-vessel occlusive vasculopathy predominantly involves the anterior circulation, in particular the supraophthalmic ICA, proximal MCA, and ACA near the carotid-T (Stockman et al 1972). Again, the pathomechanism is not completely understood. One reasonable hypothesis is that sickle cells are “sticky” and particularly tend to attach to the vessel endothelial cells, leading to activation and damage of the endothelium. This damage and activation is thought to then induce a vicious cycle, whereby inflammatory mediators, adhesion molecules, procoagulants, oxidants, and growth factors are continuously produced which further activates endothelial and white cells, creating more damage and activation. Furthermore, a fibrous intima proliferation as well as migration and proliferation of smooth muscle cells occurs, causing hyperplastic lesions with increasing vessel narrowing (Brass et al 1988, Platt 2005, Rothman et al 1986, Seibert et al 1998). Finally, acute thrombotic vessel occlusion leading to ischemic stroke might occur. Postmortem analysis often reveals arteries occluded by a thrombus containing sickle red cells, platelets, white blood cells, thrombin, and fibrin (Balkaran et al 1992). The progression of large intracranial artery stenosis leads to an increasing stroke risk in children with SCD. Patients developing a high stroke risk can be identified in time by ultrasound with transcranial Doppler (TCD) or TCCS blood flow velocity analysis. Both methods allow diagnosis and monitoring of intracranial flow velocities to determine the individual stroke risk and select patients for prophylactic treatment like regular transfusion therapy (Adams et al 1998). Transfusion therapy suppresses the patient’s erythropoiesis and consecutive HbS fraction. This is thought to interrupt the cascade of intima proliferation and large arterial vessel narrowing. Remission of arterial stenosis with normalization of flow velocities has been reported. Conversely, discontinuation of periodic transfusions may cause a relapse with increased flow velocities and stroke risk (Adams and Brambilla 2005). It remains unclear whether the dynamic of flow velocity changes during transfusion therapy might predict the safety of switching to hydroxyurea therapy (Kwiatkowski et al 2011). Hydroxyurea stimulates HbF production, and HbF reduces the polymerization of deoxyhemoglobin S and inhibits the sickling of the red cells (Bunn 1997). This leads to a reduction of hemolysis and an increase of the hemoglobin concentration. A further effect of hydroxyurea is the reduction of HbS, reduction of the aforementioned SCD-related complications, and reduction of blood flow velocities in the intracranial arteries but not a decreased stroke recurrence (Kratovil et al 2006, Wang et al 2000, Zimmerman et al 2007). However, our own data failed to show differences across intracranial flow parameters between patients with and without hydroxyurea treatment (Doepp et al 2012). Stem-cell transplantation is an alternative treatment option in patients, to avoid long-term transfusion therapy and its associated problems (Adams and Brambilla 2005). In our patient, hemolytic anemia and intracranial arterial flow velocities normalized completely after stem-cell transplantation, indicating remission of anemia-related hyperperfusion. Assessment of intracranial arterial blood flow velocities using TCD and TCCS enables physicians to detect and evaluate basal cerebral artery stenosis and to identify patients, including SCD patients, at increased risk for stroke (Adams et al 1997, Malouf et al 2001). However, a correct interpretation of velocity data needs to consider the presence of anemia. Low hemoglobin and hematocrit values lead to decreased blood viscosity, lowered oxygen-carrying capacity, and increased cardiac output which is the main reason for increased flow velocities in anemic patients and also in anemic SCD (Brass et al 1988, Doepp et al 2012). If stenoses are present, the anterior circulation with the distal ICA as well as the proximal MCA and ACA are mainly affected and should therefore be preferentially examined with TCD or TCCS. Velocity registration using TCD primarily considers Vmean for historical reasons. TCCS typically uses peak systolic flow velocities which is the recommend parameter for analyzing stenosis anywhere in the body including the brain-supplying arteries. In general, normal children have a higher CBF and therefore concomitantly higher intracranial blood flow velocities than adults (Schöning and Hartig 1996, Schöning et al 1996). Even higher velocities are seen in children with anemia and reduced hemoglobin or hematocrit. SCD patients with anemia were shown to have 40–50% higher flow velocities than healthy children (Adams 2007). Therefore, cut-off values for mild, moderate, and severe intracranial stenosis have been exclusively established for SCD. A normal Vmean in the MCA in SCD without stenosis ranges between 100 and 130 cm/s (Adams et al 1989). Compared with catheter angiography, a Vmean exceeding 190 cm/s was reported as a cut-off value for stenosis of the M1-MCA, A1-ACA and P1-PCA with a sensitivity and specificity of 90% and 100%. This cut-off also takes into consideration additional criteria, such as poststenotic or absent flow pattern in the MCA and signs of collateral flow. The same group established a slightly lower threshold (Vmean MCA ≥170 cm/s) using TCD as the best cut-off value for predicting stroke risk in children with SCD with a sensitivity and specificity of 86% and 91% (Adams et al 1992b). These findings were further supported by a large prospective randomized trial, the Primary Stroke Prevention in Sickle Cell Anemia Trial (STOP trial). Analyzing the effectiveness of transfusion therapy in SCD, the trial defined a Vmean MCA of 170–200 cm/s as conditional and >200 cm/s as abnormal (Adams et al 1998). Based on the STOP criteria, abnormal findings were seen using TCCS in 8% of cases and in 9.4% of cases analyzed by TCD in 125 asymptomatic patients (Malouf et al 2001). Two points regarding the comparison between TCD and TCCS deserve mention. First, velocity measurements without angle correction showed a better accuracy than measurements using angle correction. Second, when compared with TCCS, a slightly higher efficiency was seen for the use of TCD (92% versus 90%) (Arkuszewski et al 2012). Conversely, another study with 110 patients showed that TCCS peak systolic flow measurements were superior to TCD-determined Vmean values (Naffaa et al 2015). Potential differences of flow velocities between TCD and TCCS without and with angle correction are important for clinical practice (not only in SCD), and were systematically analyzed by several authors. Values of Vmean ~10–20% higher were found in all basal cerebral arteries using TCD compared with non-angle-corrected TCCS (Jones et al 2001, Krejza et al 2007a, Seibert et al 1998). The differences were negligible, however, when the angle-corrected TCCS was used (Krejza et al 2007a). Other authors described method-dependent differences of flow velocities in some but not in all intracranial arteries (Neish et al 2002). Low flow velocities (<70 cm Vmean in MCA, ACA, or ICA) were also reported as a risk factor for stroke, which needs further clarification as low flow may indicate severe compromise of the microcirculation or reflect severe stenoses in the distal MCA and/or ACA segments (Buchanan et al 2013). Flow velocity ratios between the intracranial ICA or MCA and extracranial ICA similar to the Lindegaard index (LI) in patients after subarachnoid hemorrhage (SAH) has been proposed as an alternative approach (for further reading on SAH, see Case 33). The interindividual range in asymptomatic children without intracranial stenosis is relatively high, however (Pawlak et al 2009), and in contrast to the LI no reliable cut-off values for relevant stenosis exist. In general, unilateral high flow velocity is suggestive of stenosis, whereas bilateral increased velocity might alternatively be anemia-related cerebral hyperperfusion. However, intraindividual side-to-side differences are often very high in SCD children without intracranial stenosis (Krejza et al 2011). Therefore, side-to-side indices should be interpreted with caution and are probably not reliable to differentiate hyperemia from arterial stenosis. TCCS extended criteria were proposed to be indicative of stroke risk: (1) Peak systolic flow velocity in the MCA >200 cm/s; (2) mean flow velocity in the MCA >170 cm/s; (3) systolic flow in the OA >35 cm/s; (4) low resistance indices in the OA (<0.6) and (5) intracranial arteries (<0.3); (6) OA flow velocity higher than the ipsilateral MCA flow; (7) flow velocity in the PCA, VA, or BA higher than in the MCA; (8) turbulent intracranial flow patterns; and (9) PCA reading without MCA signal detection (Bulas 2005, Seibert et al 1998). The best cut-off to predict stroke over an 8-year follow-up period was found if two or more of these criteria were present (sensitivity 69% and specificity 80%), although the positive predictive value was only 50%, indicating a high number of patients at lower stroke risk beside pathologic TCCS findings (Seibert et al 1998). One reason for this discrepancy might be that many of the extended TCCS criteria reflect cerebral hyperperfusion rather than relevant intracranial stenosis. The somewhat similar effects that both hyperperfusion and vessel narrowing have on cerebral hemodynamics might be the crucial factor explaining the differences reported between angiographic and ultrasound methods (Abboud et al 2004, Adams et al 1992b, Seibert et al 1998). The role of hyperperfusion on intracranial flow velocities can be estimated by evaluating the global cerebral blood volume flow (BVF) which is equivalent to CBF using extracranial duplex sonography (Doepp et al 2012). BVF is defined as the product of angle-corrected time-averaged flow velocity and the cross-sectional area of the vessel. It can be calculated as the sum of flow volumes in both ICAs and VAs (for further reading, see Chapter 3, “Cerebral Blood Flow Volume” under “Parameters of Cerebral Hemodynamics,” and Case 27, Case 34, Case 35, and Case 41). A strong correlation exists between intracranial flow velocities and the BVF and CBF, and a parallel increase of BVF and intracranial flow velocity was found in SCD patients compared with age-matched controls (Doepp et al 2012). None of the eight patients in the above study who repeatedly fulfilled the extended TCCS criteria of critical flow velocities developed stroke or showed intracranial stenosis on MRA. A comparable constellation is seen in the patient presented here. Similar to the differentiation of vasospasm and hyperperfusion after SAH, the concomitant examination of intracranial venous flow velocities may further allow the distinction between both different vascular conditions (for further reading, see Case 33). Despite these limitations, monitoring SCD children with TCD and TCCS is of enormous clinical relevance. About 10% of asymptomatic SCD children reveal pathologic flow velocities suggestive of significant arterial stenosis (Adams et al 1992a) and the spontaneous risk of stroke in these children is ~40% (Platt 2005). Regular blood transfusion therapy in children with abnormal TCD findings, however, results in a 92% reduction in stroke risk (Adams et al 1998), while the intracranial flow velocities tend to normalize (Adams and Brambilla 2005, Kwiatkowski et al 2011, Minniti et al 2004). Based on these studies, TCD and TCCS are highly recommended as screening methods in SCD children (Class I evidence; Sloan et al 2004). Peak systolic flow velocity measurement, assessed by TCCS or TCD, is advised instead of Vmean assessment as it avoids measurement artifacts caused by insufficient envelope curve placement. Transcranial ultrasound should be performed annually in all children with SCD. Children with abnormally high flow velocities in the intracranial ICA, MCA, and/or ACA should undergo reassessment within 6 months according to internationally accepted guidelines (Goldstein et al 2001). If the reassessment within 6 months is also pathologic, MRI and MRA are recommended to search for intracranial vessel narrowing and exclude silent ischemic stroke. If ischemic lesions are diagnosed or flow velocities remain increased, MRI and MRA should be routinely repeated every year and initiation of transfusion therapy should be considered. Of note, extracranial flow assessment should also be done to exclude concomitant hyperperfusion.
Sickle Cell Disease
Clinical Presentation
Question to Answer by Ultrasound
Initial Neurosonologic Findings
Extracranial Duplex Sonography
Transcranial Duplex Sonography
Conclusion
Follow-up Neurosonologic Findings over 4 Years
Clinical Course
Neuroradiologic Findings
Neurosonologic Findings after Bone Marrow Transplantation
Extracranial and Transcranial Duplex Sonography
Conclusion
Discussion
Clinical Aspects
Angiologic and Anatomic Aspects
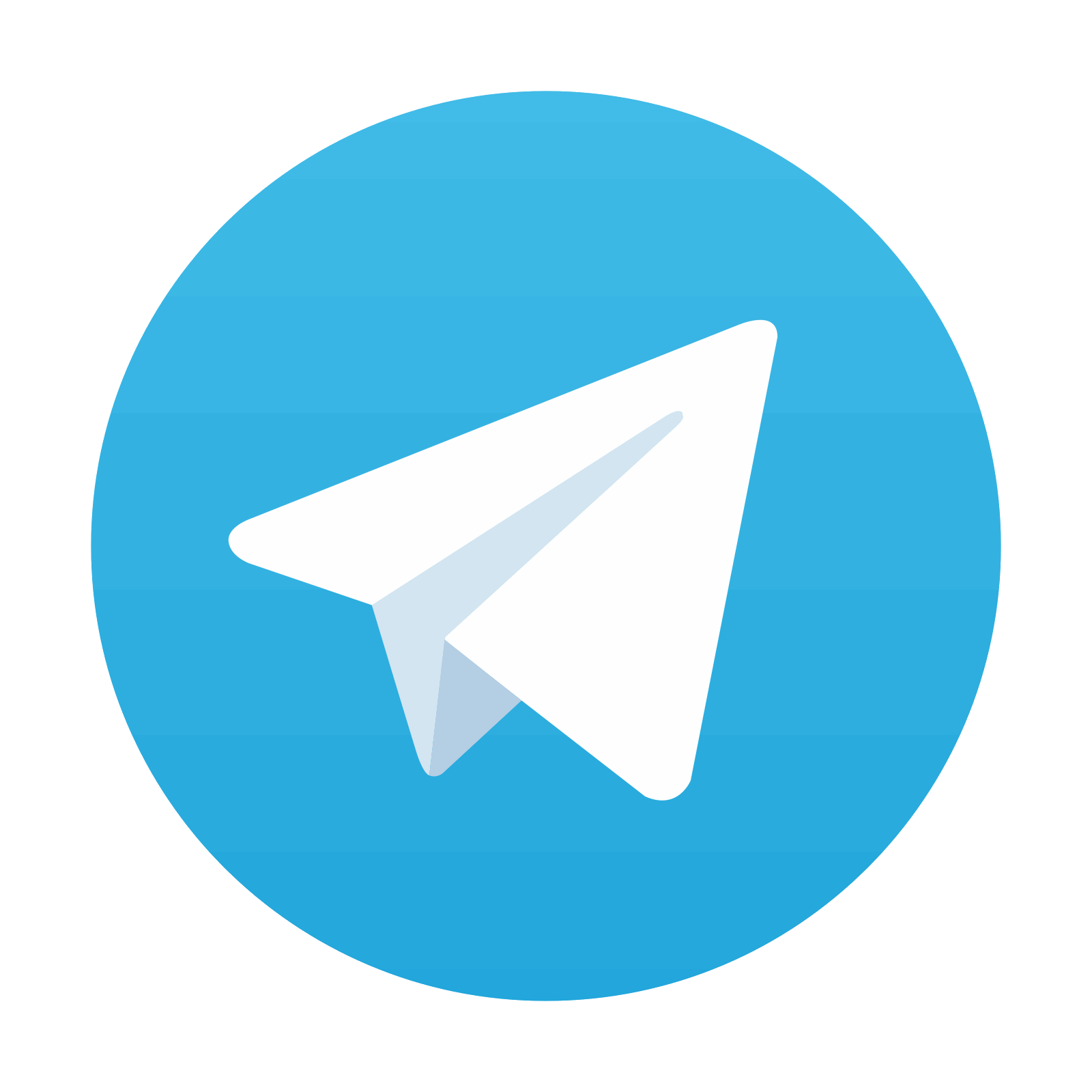
Stay updated, free articles. Join our Telegram channel

Full access? Get Clinical Tree
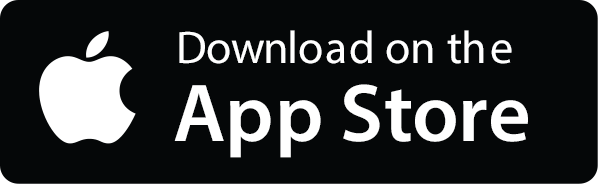
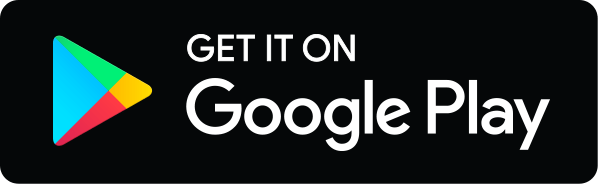