11 Speech Mapping
Abstract
Cytoreduction surgery plays a fundamental role in the management of patients with low- and high-grade glioma. Maximal resection can be difficult to achieve due to tumor proximity to functional cortical and subcortical structures. Current evidence suggests that a more extensive surgical resection is associated with longer survival and improved quality of life, therefore, maximal safe resection is the goal whenever feasible. Intraoperative speech and language mapping using direct cortical and subcortical stimulation is a useful tool to improve extent of tumor resection while minimizing morbidity. This chapter summarizes the evidence supporting the role of surgery for patients with low- and high-grade gliomas within functional areas focused on cortical and subcortical neuroanatomy, preoperative planning, intraoperative language mapping technique, and complication avoidance.
11.1 Introduction
Surgical resection plays a central role in the management of intrinsic brain tumors, and there is a growing body of evidence concerning the value of extent of resection to improve patient outcome and quality of life (QOL). The challenge, however, remains in that many intrinsic brain tumors are within regions of presumed functional significance. This is particularly true when considering cortical and subcortical speech and language areas in proximity to the tumor. Intraoperative brain mapping via the awake craniotomy, functional neuronavigation, and a firm understanding of relevant neuroanatomy are useful tools to improve extent of tumor resection while minimizing morbidity. Current evidence suggests that a more extensive surgical resection is associated with longer survival and improved QOL for patients with either low- or high-grade gliomas. This chapter outlines the evidence supporting the role of surgery for intrinsic brain tumors, surgical neuroanatomy, and techniques for intraoperative speech and language mapping to maximize extent of resection while minimizing morbidity.
11.2 Surgical Neuroanatomy: Dorsal and Ventral Language Pathways
11.2.1 Cerebral Hemispheres and Craniocerebral Relationship
A knowledge of cortical landmarks, anatomy, and subcortical structures is critical when considering speech mapping for intrinsic brain tumors. The adult cerebrum is divided into two hemispheres containing frontal, parietal, temporal, occipital lobes, and the insula. The cerebrum is divided by six principal sulci which are continuous: the sylvian, callosal, parieto-occipital, collateral, central, and calcarine sulci. There are also two interrupted sulci: the precentral and inferior temporal sulci.
The perisylvian language network is critical for speech mapping and is based along the frontal, parietal, temporal lobes in addition to the insula. The frontal lobe is separated by two main sulci: the superior and inferior frontal sulci which divide the lateral surface of the frontal lobe into the superior, middle, and inferior frontal gyri. These extend from the precentral sulcus and are oriented in the anterior-posterior direction. Running parallel to the central sulcus is the precentral sulcus. The inferior frontal gyrus is further divided by the anterior ascending and the posterior rami of the Sylvian fissure into the pars orbitalis, triangularis, and opercularis. Broca motor speech area is classically defined by the pars opercularis and pars triangularis. Along the posterior border of the frontal lobe is a readily recognizable landmark, the Greek letter “Ω” (omega) seen at the intercepting point between the superior frontal and precentral sulci which marks the hand region of the motor cortex.
Boundaries of the parietal lobe include the interhemispheric fissure medially, the Sylvian fissure and temporal-occipital line inferiorly, the central sulcus anteriorly, and the lateral parietal-temporal line posteriorly. There are two main sulci in the parietal lobe: postcentral and intraparietal sulci. The intraparietal sulcus divides the parietal lobe into the superior and inferior parietal lobules. The superior parietal lobule is superomedial and smaller and continues as the precuneus medially, whereas the inferior parietal lobule comprises the supramarginal and angular gyri. The supramarginal gyrus is the posterior continuation of the superior temporal gyrus, and the angular gyrus is the posterior continuation of the middle temporal gyrus (MTG). The temporal lobe is marked superiorly by the Sylvian fissure. The temporal lobe has the superior and inferior temporal sulci which divide the temporal lobe into superior, middle, and inferior temporal gyri. These three temporal gyri converge anteriorly to form the temporal pole.
Separating the frontal, parietal, and temporal lobes is the Sylvian fissure, which is composed of both deep and superficial portions. Deep to the Sylvian fissure lays the insular cortex which is covered by the frontal and temporal operculum. The superficial portion of the Sylvian fissure contains a stem and three rami. The stem extends medially from the uncus to the lateral end of the sphenoid wing, where it divides into the anterior horizontal, anterior ascending, and posterior rami. The deep portion of the Sylvian fissure is divided into sphenoidal and operculoinsular compartments. 1 The sphenoidal compartment arises anteriorly in the region of the limen insulae and extends laterally toward the anterior perforated substance. 1 At the threshold between the carotid cistern medially and the Sylvian fissure laterally is the limen insula. The central sulcus of the insula divides the lateral surface of the insula into three anterior short gyri (anterior, middle, and posterior gyri) and two posterior gyri (anterior and posterior long gyri). 1 Encircling the insula is the circular sulcus (also known as the superior and inferior limiting sulci of the insula) which separates it from the overlying opercula.
11.2.2 White Matter Tracts
A contemporary view of human language has shifted from a rigid organization to a more dynamic view of brain processing. 2 Large-scale subcortical white matter networks facilitate complex functions such as language, movement, and cognition. The primary fasciculi of clinical significance for speech and language are (1) the superior longitudinal fasciculus (SLF), (2) uncinate fasciculus (UF), (3) inferior occipitofrontal fasciculus (IFOF), (4) arcuate fasciculus (AF), (5) middle longitudinal fasciculus (MdLF), and (6) intermediate longitudinal fasciculus (ILF; Fig. 11‑1). The SLF is separated into SLF II, SLF III, and SLF-tp. In general, the SLF curves around the insula and connects the frontal, parietal, and temporal lobes. Specifically, SLF II connects frontal and parietal lobes allowing communication between the dorsal premotor and prefrontal cortices to angular gyrus. SLF II also contains frontal to parietal connections terminating within the supramarginal gyrus. SLF-tp joins temporal and parietal lobes running in the posterior direction from inferior parietal to posterior temporal lobe. The UF lies within the anterior insula along the limen insulae and connects the basal frontal lobe with the temporal lobe by extending under the Sylvian fissure within the insula. Connecting the frontal and occipital lobes, as well as the posterior portion of the temporal and parietal lobes, is the IFOF. The IFOF runs anterior to posterior passing through the anterior floor of the external capsule medial to the temporal lobe, sending radiations to the MTG, ITG, and occipital lobe. The AF connects frontal-opercular cortical sites with posterior temporal cortex and is thought of classically as connecting Broca with Wernicke regions. MdLF connects anterior and posterior temporal regions while ILF connects the temporal pole to the occipital lobe.

11.3 Evidence-based Clinical Decisions
11.3.1 Preoperative Planning
Preoperative clinical evaluation includes a baseline language assessment performed within 24 hours of surgery. High-quality contrast magnetic resonance imaging (MRI) scans with and without enhancement are vital for developing the optimal operative plan. Diffusion tensor imaging (DTI) for white matter tracts or task-based functional brain MRI (fMRI) are helpful studies to assist with preoperative planning. 3 , 4 Preoperative MRI provides insight into tumor size, location, focality, vascularity, mass effect, peritumoral edema, and proximity to areas of potential functional significance. The optimal transcortical approach for approaching intrinsic tumors during speech and language mapping is along the equator of the mass. 5 In addition, MRIs can be reconstructed to create three-dimensional models combined with tractography that can be used during surgery (neuronavigation; Fig. 11‑2). DTI tractography, fMRI, and perfusion MRI enable clinicians to make more accurate preoperative diagnoses and provide information regarding the interface of tumor tissue with adjacent functional cortical and subcortical pathways. The fMRI uses the blood-oxygenation-level-dependent (BOLD) signal to identify cortical and subcortical regions of activation to create an operative corridor or plan for resection that minimizes risk to eloquent surrounding structures. 6 , 7 Perfusion MRI is useful for evaluating tumor angiogenesis, endothelial permeability, and potentially distinguishing between recurrent glioma and treatment-related changes. DTI tractography is used to mark the subcortical dorsal and ventral language tracts surrounding a tumor and is commonly used in surgical planning. 8 , 9 , 10 For the brain tumor surgeon, functional and anatomic image guidance is an essential part of determining the risks associated with surgery and for consulting patients about potential postoperative neurological outcomes. Image guidance also allows for a generalized preoperative impression of where a functional pathway might be displaced in relationship to a space-occupying lesion. Neuronavigation is widely used in brain tumor surgery that can be integrated with DTI tractography, magnetoencephalography (MEG), or fMRI to identify cortical and subcortical areas of potential sensory, motor, language, and visual significance (Fig. 11‑1 and Fig. 11‑2). 11 Neuronavigation can, therefore, generate individualized maps of functional areas and their relationship with mass lesions within the brain. These studies, however, lack sensitivity, given the variability in individual patient’s neuroanatomy, distortion due to mass lesions, and functional reorganization caused by plasticity make classic anatomic identification of functional areas insufficient. 12 , 13 Compared with direct cortical stimulation, fMRI has a sensitivity and specificity of 91 and 64% for identification of Broca area, 93 and 18% for identification of Wernicke area, and 100 and 100% in motor areas, respectively. 14 Resting state coherence measured with MEG is capable of mapping functional connectivity of the brain. Intrinsic brain tumors with decreased resting state connectivity have a relatively low risk of postoperative language deficits, while those with increased resting state connectivity are associated with higher risk of postoperative aphasia. 15 Identification of subcortical pathways is critical to prevent injury to white matter pathways and preserve function. 16 The gold standard approach for resection of intrinsic brain tumors within speech areas is cortical and subcortical intraoperative brain mapping.

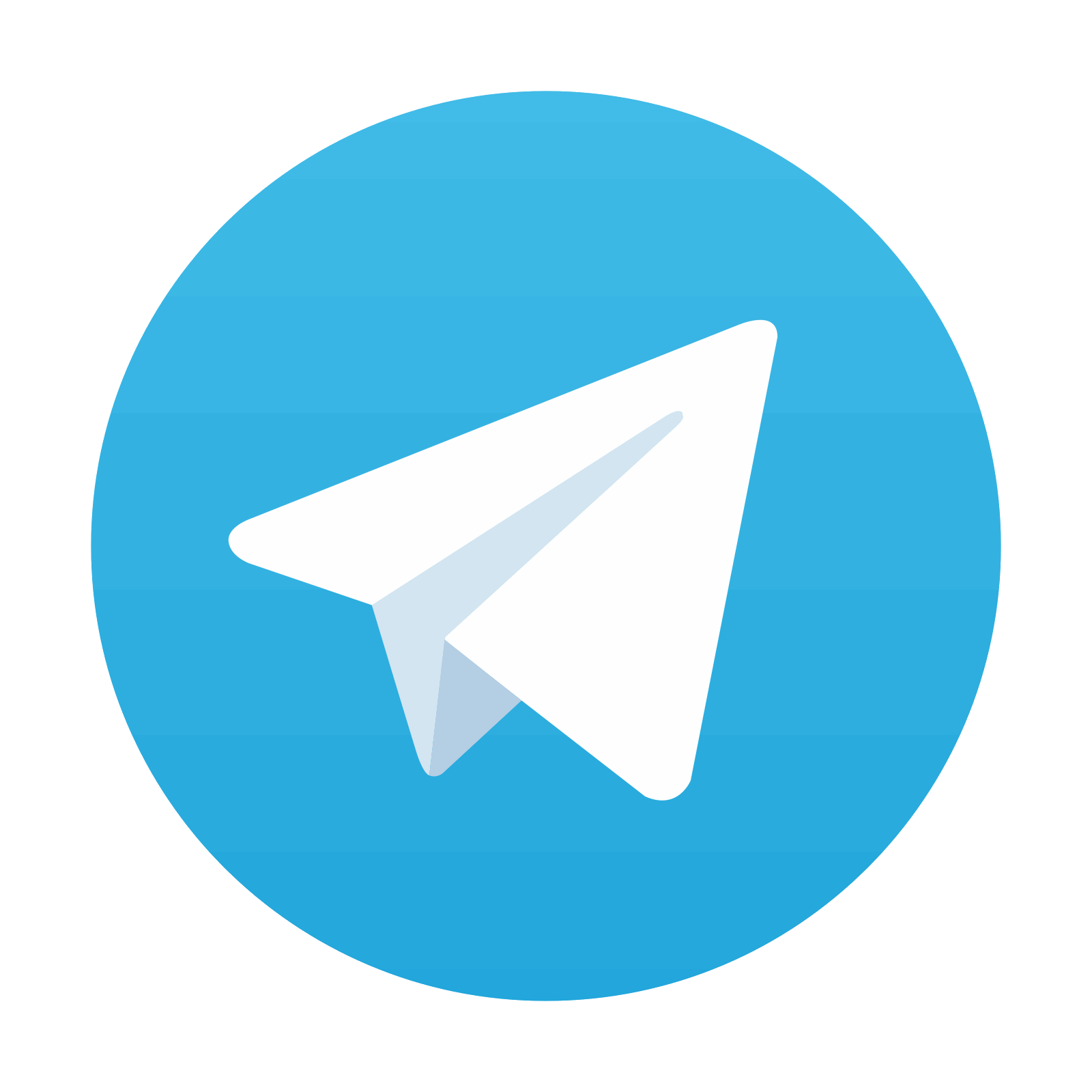
Stay updated, free articles. Join our Telegram channel

Full access? Get Clinical Tree
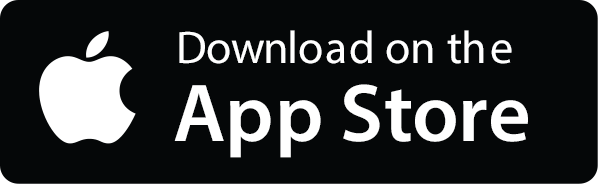
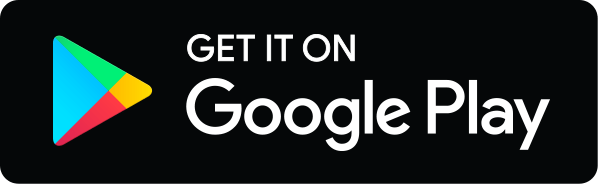
