14 Cerebral Protection Measures
Abstract
Once ischemia begins in the brain time becomes critical to recognize and treat the condition. At times the systems of the body cannot be directed by nervous system communication to restore the delivery of vital nutrients or remove the cause preventing the delivery. The neurointensivist can come to the aid of the nervous system and provide augmentation to its structure and function through the judicious use of oxygen, blood pressure, and surgery. However, when those initial interventions are not adequate, when the intracranial pressure is refractory, heroic measures can be considered when adequate survival is possible. These measures are precisely delivered and not without considerable side effects.
Case Presentation
A 32-year-old man was an unrestrained driver involved in a head-on traffic collision at high speed. The patient was intubated in the field, and his Glasgow Coma Scale (GCS) score on arrival to the emergency room was 6 T. Head computed tomographic scan shows diffuse petechial hemorrhages and left frontal contusion, with no midline shift or surgical hematoma demonstrated. The patient is transferred to the intensive care unit where an intracranial pressure (ICP) monitor and cerebrospinal fluid drainage device is promptly placed. Initial ICP is 20 mm Hg; however, 4 hours later the on-call physician is notified that the ICP has slowly been moving upward and is now at 35 mm Hg.
See end of chapter for Case Management.
14.1 Introduction
The ultimate result of a severe head injury primarily depends on the extent of injury at the time of incident and the extent of secondary injury in the ensuing days after the initial injury. This chapter discusses the therapies for prevention of secondary injury, the only modifiable variable from the neurocritical care standpoint.
A primary brain injury from trauma includes contusions, epidural or subdural hematomas, intracerebral hemorrhage, and diffuse axonal injuries. Secondary brain injuries include edema, ischemia, and hypoxemia, which can result in infarction and/or herniation. Initially, surgical intervention for a space-occupying mass should always be considered before proceeding to neurocritical care management. A space-occupying lesion in itself will result in secondary injury via herniation and brain ischemia if untreated.
Secondary brain injury initiates numerous pathological and chemical changes that significantly impact the patient’s neurologic outcome. The mainstay of treating a secondary brain insult is to ensure adequate cerebral oxygenation. Metabolic requirements increase in the damaged brain tissue, and sufficient oxygenation is imperative to prevent cell apoptosis and secondary injury. Oxygen delivery to the brain is governed by (1) cerebral blood flow (CBF) via intracranial pressure (ICP) and cerebral perfusion pressures (CPPs), (2) oxygen carrying capacity of the blood, and (3) arterial partial pressure of oxygen. Many studies indicate that the overall outcome for patients with severe traumatic brain injury (TBI) is directly affected by hypoxia and elevated ICPs. 1 , 2
Because CBF is difficult to measure, physicians can easily follow the mean arterial pressure (MAP) and ICP to estimate CPP. CBF is related to MAP and ICP, as shown in the following equations:

where CVR is cerebrovascular resistance.
The equations show the complex relationship between brain perfusion and blood pressure. The Monro–Kellie hypothesis, which helps to explain the significance of ICP, states that the sum of the intracranial volumes of blood, brain tissue, and cerebrospinal fluid (CSF) are constant and that any increase in one component will be counterbalanced by a decrease in another. The volume of brain tissue is 90%; blood and CSF are 5% each. All of these are contained within the rigid skull. The introduction of additional volume, such as from hemorrhage or edema, must be compensated by changes in the blood or CSF volumes. Failure to counterbalance these changes will result in increased ICP and possibly intracranial shifts (herniation). Increased ICP can be compensated by shifting CSF from the ventricles and subarachnoid space to the spinal canal or by decreasing the intracranial blood volume via collapsing veins and constricting cerebral arteries. Changes in vessel diameter can result in a reduction of cerebral intravascular volume by as much as 70 mL, easily buffering a new volume mass, such as a hemorrhage. 3
After compensatory mechanisms for increased ICP are exhausted the patient begins suffering from secondary brain insults. Eighty to 90% of patients with TBI who die are found to have histopathological evidence of cerebral ischemia. 4 Studies show that approximately one-third of patients with TBI experience an “ultra-early” period of significantly decreased cerebral blood within 6 hours of injury. 5 The ischemia is characterized by focal reductions in CBF below the threshold of 18 mL/100 g/min, resulting in ischemic neuronal cell death. 6 The hypoxic conditions perpetuate a hypermetabolic rate, resulting in an aerobic to anaerobic metabolism and leading to an increased concentration of lactic acid. 7 Ionic homeostasis is then compromised, leading to a complex process of calcium influx and cellular injury and death. 8
Therefore strict ICP and CPP management is paramount in prevention of secondary injury. Options available for ICP management start with basic patient positioning—with the head of the bed elevated and the neck straight to improve venous outflow. Emphasis must be placed on normocarbia with a goal of PaCO2 35–40. Short-term hyperventilation may be beneficial in acute herniation; however, the resultant reduction in CBF has been shown to worsen outcomes in periods of prolonged therapy. 2 In turn, hypercarbia must be avoided as well because it results in vasodilation and increased ICP.
Hyperosmolar agents, such as mannitol and 23.4% hypertonic saline (HTS), can be used to mobilize extravascular water to intravascular space, in turn reducing cerebral edema. Recent studies point to improved results with side-effect profile with HTS versus mannitol 9 , 10 because mannitol tends to result in a drop in blood pressure and in turn decreased CBF. In addition, with prolonged administration, mannitol tends to have a rebound increase in ICP as it collects in brain tissue where the blood–brain barrier has been disrupted. Serum sodium and osmolarity should be monitored every 6 hours with either bolus hyperosmolar therapy or continuous infusions of 3% hypertonic saline. Serum osmolality > 320 mOsmol/L and serum sodium > 160 mmol/L are associated with an increased risk of nephrotoxicity and should be avoided. Recommended target serum sodium is within the range of 145–155 mmol/L. 11
Anemia in the setting of brain injury has been shown to result in poor neurologic outcome. Laboratory studies using animal models and healthy human subjects suggest that anemia below a hemoglobin (Hb) concentration of 7 g/dL results in impaired brain function and below 10 g/dL may be detrimental to recovery from TBI. Models indicate impairment in delivery of oxygen (DO2)with hemoglobin levels < 10 mg/dL. However, it remains controversial as to the minimal threshold for packed red blood cells (PRBC) transfusion in the brain injury patient. 12 , 13 Some studies point to worse outcomes in TBI patients who receive transfusions but fail to account for severity of disease. It is the standard practice at our institution to transfuse brain injury patients with Hb levels < 10 mg/dL.
New technologies, such as the Licox Brain Oxygen Monitoring System (Integra), which monitors brain tissue oxygenation, have given the neurocritical care physician another tool to guide therapy. Therapy directed specifically at brain tissue oxygenation has shown good results. A 2012 review of patients with TBI showed that 61% of patients with TBI had a favorable outcome with brain tissue oxygen-based therapy compared with 42% of patients who had a favorable outcome with ICP/CPP goal-directed therapy. 14 Brain tissue oxygen tension of < 15 mm Hg is associated with a significantly worse outcome and death compared with patients whose PBTO2 remained > 15 mm Hg. 2 No specific guidelines for brain tissue oxygen monitoring currently exist, but it is a promising and useful adjunctive parameter for management of brain injury patients.
14.2 Pharmacological Cerebral Metabolic Depressants
An estimated 15% of all head-injured patients suffer from refractory ICP. 15 When all other medical and surgical modalities have failed to adequately control elevated ICP, the physician may attempt the use of pharmacological agents to reduce the cerebral metabolic rate (CMR) and hence ICP in a patient who is deemed salvageable. Many pharmacological agents have been used to lower ICP, such as pentobarbital, thiopental, etomidate, propofol, isoflurane, and desflurane, but not enough data exist to recommend one drug over another. Most published information addresses the use of barbiturates, especially pentobarbital.
In 1974, Shapiro et al were the first to report the use of pentobarbital-augmented hypothermia in reducing CMR and ICP. 16 This therapy remains controversial, mainly because of the potential side effects of myocardial hypotension, hypothermia, immunosuppression, hypokalemia, and hepatic and renal dysfunction. 17 Accordingly, the Brain Trauma Foundation, in cooperation with the American Association of Neurological Surgeons, has recommended a guideline using high-dose barbiturate therapy in hemodynamically stable head-injured patients with elevated ICP resistant to maximal medical and surgical treatment modalities. 2
Because of marked hypotension seen in ~ 50% of patients treated with barbiturates, many will require cardiac inotropes and/or vasopressors to maintain an MAP resulting in a CPP ≥ 70 mm Hg, 18 unless increased secondary risk concerns favor an acceptable lower CPP. Dopamine (primarily a vasoconstrictor) is commonly used starting at 3μg/kg/min and titrating to a maximum 20μg/kg/min to maintain CPP ≥ 70 mm Hg. Phenylephrine starting between 100 and 180μg/min, with a maintenance dosage of 40 to 60μg/min, or norepinephrine at 8 to 12μg/min is frequently used for progressively decreasing CPP and/or hypotension. Hemodynamic monitoring via the FloTrac sensor (Edwards) or similar monitoring may be necessary to adjust cardiac medications and to ensure adequate volume status, systemic resistance, and cardiac output.
Many protocols have been used to administer barbiturate therapy. Eisenberg’s protocol starts with a loading dose of pentobarbital at 10 mg/kg intravenous (IV) over 60 minutes, followed by 5 mg/kg every hour for 3 hours, with a maintenance dosage of 1 to 3 mg/kg/h. 19 In a study by Cormio et al on 67 patients with severe head injury undergoing treatment for refractory intracranial hypertension, pentobarbital loading doses decreased ICP and MAPs on average by 12 and 9 mm Hg, respectively. 20 A more recent study by Marshall et al using Eisenberg’s protocol found response rates to pentobarbital therapy for refractory ICP of 38%, defined as ICP < 25 mm Hg for 24 hours after therapy initiation. 21 It can generally be considered that if the induced pentobarbital coma does not lower the ICP within 1 to 4 hours, it is unlikely to succeed without any other therapy.
Thiopental has been used with good reported outcomes; however, availability has become a limiting factor in the United States. It works by producing cerebral metabolic depression and cerebral vasoconstriction. The loading dose is 5 mg/kg IV over 10 minutes, with a continuous infusion of 5 mg/kg/h (range 3–5 mg) for 24 hours. After 24 hours, the infusion may be decreased to 2.5 mg/kg/h because fat stores have now become saturated.
Propofol is a well-established sedative-hypnotic agent that has been used as an alternative to control elevated ICP, but questions remain regarding the pharmacodynamics of the drug. A study by Oertel et al has suggested a key mechanism responsible for the metabolic suppressive effect is a decrease in CO2 production, resulting in a global “pharmacological hypocapnia.” 22 TBI guidelines 2 recommend propofol for control of ICP, but it has not shown any improvement in mortality or 6-month outcome, and high doses can produce significant morbidity, such as in the case in propofol infusion syndrome. 23 The starting dose is 5 to 10μg/kg/min and increases by 5 to 10μg/kg/min every 5 to 10 minutes until ICP is controlled. Like the barbiturates, propofol exhibits hypotensive effects that may require cardiac medications or eventual discontinuation. In addition to the cardiotoxic effects, propofol may cause electrocardiographic changes and discoloration of urine.
A continuous electroencephalogram is recommended to monitor electrocerebral activity. Burst suppression of 1 to 3 bursts/page may not be necessary if control of ICP ≤ 20 to 25 mm Hg is achieved without the use of larger doses of the cerebral metabolic depressant agents.
Pharmacologically induced burst suppression is generally maintained 3 to 7 days; if computed tomography (CT) does not show any new findings, and ICP has been controlled, then therapy can be withdrawn slowly by reducing the infusion rate while monitoring ICP. Because of prolonged high-dose barbiturate therapy and the long half-lives of these drugs, it is difficult to distinguish the residual pharmacological effects from the clinical condition. If a high index of suspicion for brain death is entertained, a nuclear cerebral metabolic test is warranted; if the test is negative, one should proceed with a cerebral angiogram before pronouncing brain death.
The prophylactic use of barbiturates for ICP treatment has shown no benefits in patients with intracranial mass lesions and was even found to be harmful in patients with diffuse injury (mortality of 77% vs. 41% in the mannitol group). 24 A 2000 Cochrane Database review evaluating barbiturate therapy in acute TBI concluded that there was no evidence that barbiturate therapy improves outcome for patients with acute severe brain injuries. The review also demonstrated that barbiturate therapy results in a decrease in blood pressure in one in four patients, and this hypotensive effect will offset any ICP-lowering effect on cerebral perfusion. 25
Alternative agents to pentobarbital have not been well studied, but they have been used because of easier accessibility. A study comparing etomidate, isoflurane, and thiopental in animals undergoing 3 hours of middle cerebral artery occlusion found injured brain volume largest in the etomidate and isoflurane groups and smallest in the thiopental group. 26
Surgical management for refractory ICP in the form of decompressive craniectomy should be considered prior to or in conjunction with metabolic depressants or hypothermic therapies. The study by Aarabi et al 27 comparing outcome following decompressive craniectomy for refractory ICP showed a 28% mortality rate postcraniectomy compared to studies by Eisenberg et al and Marshall et al 19 , 21 on pentobarbital therapy alone in refractory ICP of 62% and 64% mortality, respectively.
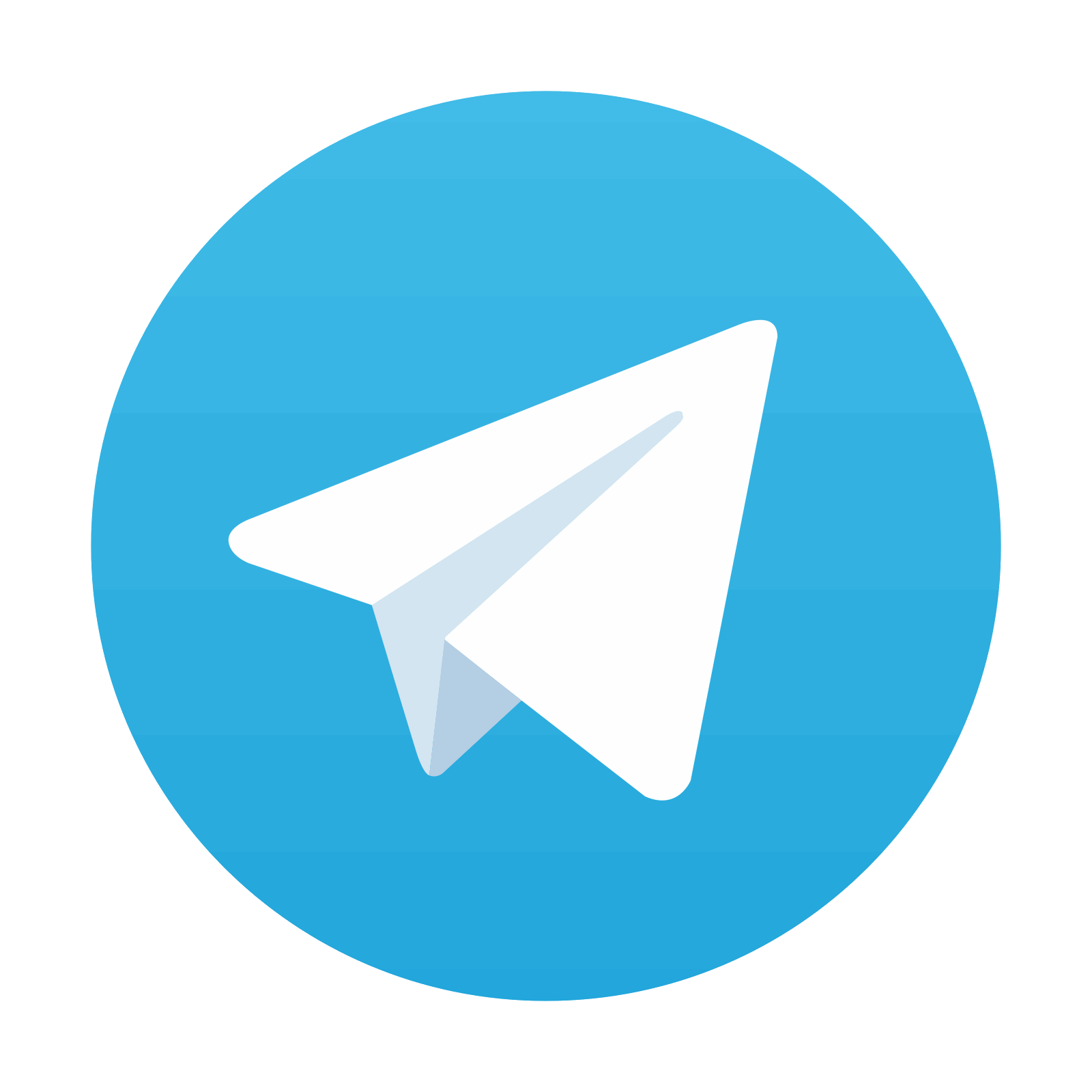
Stay updated, free articles. Join our Telegram channel

Full access? Get Clinical Tree
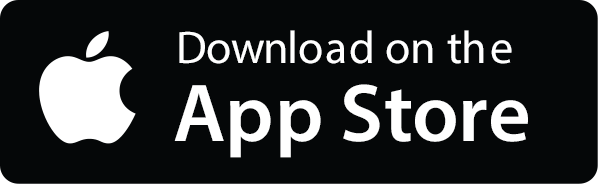
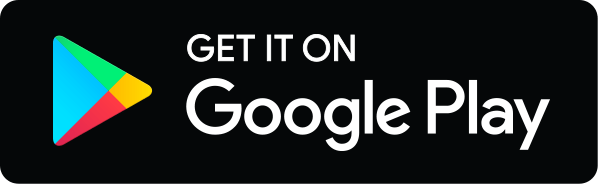