15 Mapping and Surgery of Insular Tumors
Abstract
Resection of insular tumors remains one of the most challenging areas of neuro-oncological surgery. This chapter examines the incidence and unique characteristics of insular tumors, and the evidence behind attempts to maximize extent of resection. Subsequently, key elements of the complex surgical anatomy of the insula are described, including surface and relational topographic anatomy, arterial networks, and eloquent cortical parcels and subcortical segments along with proposed mapping paradigms based on our current understanding of function. The focus is then placed on classification schemes, surgical principles, and step-by-step paradigms, along with nuances and caveats in achieving maximum safe resection.
15.1 Introduction
The surgical management of insular gliomas remains a formidable challenge as the insula is covered by eloquent cortical parcels; is encased by complex arterial and venous networks; has disputed function; is surrounded by white matter tracts; and superimposes the basal ganglia nuclei. Therefore, the surgeon embarking upon maximum safe resection of insular tumors should have a clear understanding of its surgical and physiological anatomy; be familiar with cortical and subcortical direct electrical stimulation (DES) techniques predicated on thorough working knowledge of functional significance of cortical parcels and subcortical segments; and work with the support of wider teams, including specialists from the fields of advanced functional neuroimaging, neuroanesthesia, neuropsychology, and cognitive neuroscience.
15.2 Epidemiology
Gliomas are the commonest primary intraparenchymal tumors of the brain in adults, with an incidence rate of 4.7 to 5.7 per 100,000 population. The insula is a common location for gliomas, with studies reporting approximately 25 and 10% incidence of low- and high-grade gliomas, respectively. It remains unclear as to why the insular location is relatively overrepresented in glioma epidemiology, although several explanations have been proposed. 1
Insular gliomas are considered to have worse outcomes than gliomas in other locations, with a higher frequency of the astrocytic phenotype and, consequently, reduced incidence of the better prognostic profile of oligodendrogliomas. In one study of World Health Organization (WHO) grade II gliomas involving the insula, no patients had complete 1p and 19q deletions and only 25% had partial deletions. 2 Indeed, it has been shown in a large Chinese sample of 1,210 high- and low-grade glioma (LGG) samples that lesions in the insular lobe were more likely to be IDH-mutant astrocytomas (29.6%). 3 However, other authors have suggested insular gliomas follow a more indolent course than gliomas elsewhere. 4 Although other types of tumors, including dysembryonic neuroepithelial tumors and gangliogliomas, have been described in the insular region, the focus of this chapter is on mapping of gliomas which are by far the commonest tumors encountered in the insula.
15.3 Clinical Presentation
Insular gliomas tend to present differently than gliomas located elsewhere. Although some have argued that they present with a protracted and slowly progressive clinical course, 5 others have proposed that populations of patients with insular gliomas have a high proportion of patients with intractable seizures. 6 Indeed, epilepsy is commonly the only presenting symptom of insular gliomas. 5 , 7 , 8 Other presentation symptoms and signs may include expressive dysphasia with or without paresis of the facial muscles. 9 Cognitive dysfunction is also common in patients with insular glioma, potentially due to infiltration of white matter tracts and in particular the inferior fronto-occipital fasciculus (IFOF) which is involved in supramodal semantic processing. 10 This necessitates a comprehensive preoperative neuropsychological and cognitive assessment prior to surgical intervention.
15.4 Evidence on Extent of Resection
15.4.1 Low-Grade Gliomas
Maximum safe resection of LGGs as initial therapeutic option, regardless of topographical location, is strongly supported by current literature. 11 , 12 , 13 , 14 , 15 Progression-free survival (PFS), overall survival (OS), and rates of malignant transformation are statistically improved with greater extent of resections (EOR), although discrepancy exists in the current literature with regard to the percentage of residual tumor volume and absolute residual tumor volume (cm3) required to produce a statistically significant survival benefit. More specifically, Smith et al reported that a minimum EOR of > 90% of original volume is required to produce survival benefit and, more recently, absolute residual tumor volumes stratified between 0 , 0.1 to 5.0, and > 5.0 cm3 were associated with consecutively decreasing survival benefit for each group. 11 Also, in a “near-randomized” trial, Roelz et al reported that survival benefit was recorded in patients with absolute residual tumor volume of < 15 cm3 and patients, in their series, where this threshold was not reached fared similar to the biopsy-only group. 12 Finally, also accounting for molecular subtyping, a recent large retrospective study showed that any residual postoperative volume affected negatively the OS regardless of the tumor’s molecular profile, even if the residual volume was only 0.1 to 5.0 cm3. 15
15.4.2 Insular Low-Grade Gliomas
EOR has been shown to strongly influence OS and PFS, among additional factors such as age, performance status, tumor volume, and histological grade and molecular profile. 16 , 17 , 18 , 19 , 20 Multiple studies have shown that a greater EOR in low- and high-grade insular gliomas is associated with improved OS and PFS, 5 , 21 , 22 as well as seizure control. 17 , 23 , 24 Transient immediate postoperative worsening of functional status is not uncommon in some of the reported series (14.4–59%), 5 , 7 , 23 , 25 but permanent neurological deficits in more recent series are low (0–6%). 5 , 7 , 23 , 25 , 26
One research group has proposed four factors as indicators to select the candidates that are most likely to have reduced risks and improved outcomes following radical resection of insular gliomas: patients with clear tumor boundaries, no enhancement, lack of involvement of the lenticulostriate arteries, and preservation of the superior extremity of the central insular sulcus. 27 Another group found that preoperative identification of the IFOF on diffusion tensor imaging (DTI) was associated with a high probability of achieving a resection of > 80%. 28
In a consecutive series of 115 patients with insular gliomas, 70 low-grade (WHO grade I and II) and 45 high-grade (WHO grade III and IV), a 16% increase in 5-year OS in the low-grade group was noted when EOR was 90% or more; a 16% increase in the 2-year OS was noted in the high-grade group also with an EOR of 90% or more. 5 Crucially, EOR ≥ 90% was associated with an increased malignant-progression-free survival by 17%. 5 In one German study of 72 patients undergoing intraoperative continuous motor mapping for insular gliomas, intraoperative motor evoked potentials (MEPs) remained stable in 40 (56%) cases. 29 In the remainder, a deterioration was observed that was reversible in 21 (29%) cases, with no new permanent deficit in 9 (13%) of these cases. Furthermore, a higher rate of permanent motor deficit occurred in those where no useful MEP monitoring was obtained compared to those where irreversible MEP loss was associated with permanent paresis (18 vs. 4%).
A single-surgeon series of 51 consecutive WHO grade II insular gliomas operated on with DES (some awake) found that although there was an immediate postoperative deficit in over half of the patients (59%), the condition in all but two returned to baseline or actually improved over baseline status during follow-up. 7 The same study also found that 78% of patients, with chronic epilepsy preoperatively, had relief of seizures following surgery. 7 A study from the same author focusing on 24 patients with WHO grade II insular gliomas who underwent awake craniotomy with intraoperative language mapping involving DES, found similar results: exactly 50% of patients had immediate postoperative language worsening, but all recovered within 3 months, and six patients improved their preoperative neurological deficits following surgery. 26
A retrospective review from a single center of 22 patients with insular gliomas operated via a transopercular approach found that the majority (91%) of patients were seizure free and only 1 had persisting neurological deficit after a mean follow-up of 33.4 months. In this series, 73% of patients had an EOR ≥ 90%, and the remainder had macroscopic gross total resection. Finally, a recent retrospective series of patients with insular gliomas who were reoperated on for recurrence has shown that such operations can be undertaken safely with an acceptable degree of resection, irrespective of insular zone and pathology. 30
15.4.3 Anatomical Basis of Insular Mapping and Surgery
Using a modified Klinger technique, the authors used formalin-fixed cadaveric brains which were subsequently frozen at −10 to −15°C for 10 to 14 days and then thawed under running water in a technique described extensively in the literature. The brains were dissected by the authors, under a Zeiss operating microscope (Zeiss, Oberkochen, Germany) with use of microinstruments and wooden spatulas. The anatomical structures were observed. Institutional permission for dissections obtained and photographs were taken from identifiable and electronically tagged specimens, according to Human Tissue Act, 2004, Human Anatomy Unit best practice guidelines and the Data Protection Act, 1998. Authorization was obtained from the Human Anatomy Unit, Division of Surgery, Imperial College London, London, UK. Structures were sequentially identified and are described below, with accompanying photographs.
15.4.4 Cortical Insular Anatomy
The pyramid-like, three-dimensional body of the insula lies in the depth of the Sylvian fissure and is covered by the opercula of frontal, parietal, and temporal lobes. The insula consists of paralimbic mesocortex which is phylogenetically between iso- (or neo-) cortex and allocortex. Other paralimbic structures include the caudal orbitofrontal cortex, the temporal pole, and the parahippocampal and cingulate gyri.
The insula is demarcated by the triangular limiting, rather than circular, sulcus of the insula and its three parts—the anterior, superior, and inferior limiting sulci; there is no posterior limiting sulcus. The surface projections of the limiting sulci are the anterior part of the pars triangularis for the anterior limiting sulcus (ALS); the inferior frontal gyrus anteriorly and the supramarginal gyrus (SMG) posteriorly for the superior limiting sulcus (SLS); and the depth of the superior temporal sulcus for the inferior limiting sulcus (ILS) of the insula (Fig. 15‑1). 31 The ALS and SLS are straight sulci but the ILS is curved and divided into an anterior and a posterior part with the junction of the two parts lying medial to the anterior part of the Heschl gyrus. 32

The surface of the insula is divided by the central sulcus of the insula, which lies in the same plane to the central sulcus of the ipsilateral hemisphere, into an anterior and posterior part. The anterior insula has five gyri, the anterior, middle, and posterior short gyri, and also the transverse and the accessory gyrus, placed most anteriorly and concealed by the pars orbitalis. All five gyri converge to the apex of the insula, the most prominent part of the insular surface. The posterior short insular gyrus is considered to be the Dronkers territory, involved in articulatory planning of speech (Fig. 15‑1). 33
The anterior Sylvian point, a visible expansion of the subarachnoid space of the Sylvian fissure at the bottom of pars triangularis, corresponds to the apex of the insula, allowing the surgeon to mentally reconstruct the projection of the insula into the lateral aspect of the brain. The posterior insula displays two long gyri, anterior and posterior, separated by the postcentral sulcus.
The limen insula is a hook-like structure consisting of a narrow strip of olfactory cortex 34 that creates a gap between the ALS and ILS. It is readily seen when the Sylvian fissure is split 32 and represents the lateral limit of the anterior perforating substance. 35 The limen insula, which covers part of the uncinate fasciculus (UF), lies just inferior to the insula apex having the temporal incisura anteroinferiorly (temporal connection) and the posteromedial orbital lobule superoanteriorly (frontal connection).
Four superficial insular points have topographical importance: (1) the anterior insular point, at the junction of the ALS and SLS; (2) the posterior insular point, at the junction of the SLS and ILS; (3) the frontal limen point, at the junction of the ALS and limen insula; and (4) the temporal limen point, at the junction of the ILS and limen insula (Fig. 15‑1). 31
15.4.5 Vascular Anatomy of the Insular Region
Although the focus of this chapter remains on mapping, surgery for insular tumors is obstructed by the dense, complex arterial system of the insular region. Arterial injury of critical branches may result in permanent neurological deficits, rendering mapping irrelevant; therefore, vessel identification and preservation is essential and prerequisite for successful mapping. The insular region is characterized by the presence of approximately 100 (range: 77–112), mostly submillimeter caliber (range: 0.1–0.8 mm), arteries. 35 In addition, the complex arterial anatomy of the normal insula is complicated even further by the presence of gliomas that often encase and distort the expected topography of sphenoidal (M1) and insular (M2) arteries. 36
For the surgeon, it is essential to conceptually and topographically group the arteries of the insular region into three groups:
A superficial group of arteries that supply mainly the insular cortex, extreme capsule, and claustrum.
An intermediate group that, in addition, occasionally may supply the external capsule, putamen, globus pallidus, and rarely, can even reach the internal capsule, mainly in the posterior insula.
The lateral lenticulostriate arteries (LLAs) which do not supply the insular structures but supply the putamen, globus pallidus, and internal capsule. Despite their proximity, the insular arteries and LLAs are separate arterial systems with no direct communication.
All three arterial groups originate from the middle cerebral artery (MCA) and its main segments. The M1 segment of the MCA extends in the posterior continuation of the internal carotid artery (ICA), starting at the branching of the much smaller anterior cerebral artery and extending up to the branching of M1 into, most commonly, two (superior and inferior) segments constituting the M2 segment, covering with multiple branches the insular cortex; the bifurcation is located at the limen insula. The opercular (M3) segment extends from the limiting sulci to the cortex; due to the sharp angulation in the transition, the M3 branches are called candelabra arteries. 35 The para-Sylvian (M4) and terminal (M5) segments are cortical only, with the M5 being visible without parting the Sylvian fissure.
The M1 segment gives rise to three arterial groups:
Seven to eight (range: 1–15), straight, slim (< 0.5 mm), parallel to each other LLAs arising from the inferomedial aspect of the M1 which do not supply the insula or adjacent superficial structures, but instead, after penetrating the anterior perforating substance, supply the putamen, anterior commissure, substantia innominata, globus pallidus, caudate nucleus, and internal capsule.
Frontal branch.
Temporal branch.
This bifurcation, called by Yasargil “false,” is observed before the bifurcation of the M1 into superior and inferior trunks. The frontal and temporal branch of M1 should be distinguished from the superior and inferior trunk of the M2, although their course may be parallel. A number of branching variations exist; most commonly a temporal branch only is observed (>50%) and the second most common variation is the presence of both frontal and temporal branches (35%). 35
The insular cortex is supplied by the M2 segment with a few branches occasionally coming from M1 or, more rarely, M3 segments. The superior and inferior trunks of the M2 give rise to approximately 10 branches (range: 8–12), and most consistently, from anterior to posterior, the prefrontal, precentral, central, anterior and posterior parietal, and angular arteries. The temporal branch of M1 often gives rise to middle and posterior temporal arteries.
The M1 (proximal or sphenoidal) segment of the MCA results from the bifurcation of the ICA in the region of the anterior perforating substance and extends to the region of the limen insula where it branches to a number of patterns, including the most commonly observed bifurcation to a superior and inferior trunk, trifurcation, quadfurcation, or no branching. The M2 segment most commonly includes the superior and inferior trunks and gives off 9 to 12 arterial branches mostly from its superior trunk before transitioning with acute angles (candle tree arteries) at the level of the SLS to M3 segment. 35
15.5 Classification Schemes of Insular Tumors
A number of surgical classification schemes of insular tumors have been proposed, aiming to better understand the extent of anatomical involvement, 36 , 37 resectability, and surgical morbidity. 5
15.5.1 Yasargil Classification System
In 1992, in a thoughtful article, Yasargil reviewed 177 cases of tumors affecting the mesocortical limbic system (insula, temporal pole, parahippocampal and cingulate gyri) and the phylogenetically older allocortical paralimbic system (amygdala, hippocampus, septal region, substantia innominata, and piriform cortex), and observed a propensity for tumors to prefer expansion within meso- or allocortical regions. He grouped these tumors into mediobasal temporal, insular, and orbitofrontal-insular-temporal pole tumors. 36 Yasargil’s classification is an anatomical/developmental classification model (Table 15‑1): Type 3 tumors involve all, or part, of the insula (3A) or may involve adjacent opercula (3B); type 5 tumors may involve either or both of the orbitofrontal and temporopolar (paralimbic) regions (5A) and may involve the limbic system (5B). 38
15.5.2 Berger–Sanai Zone Classification System (2010)
Using two planes, one along the Sylvian fissure, and one across the foramina of Monro, the insular region is divided into four quadrants: clockwise starting from the frontal operculum I and II superiorly, and III and IV inferiorly to the Sylvian plane (Table 15‑2). Consequently, quadrants I and IV are anterior, and quadrants II and III are posterior to the plane of Monro (Table 15‑2). As insular tumors do not respect arbitrary planes, the grading depends on the location of the majority of tumor volume. Berger–Sanai model is a surgical planning/surgical morbidity prediction model. It has been shown that this classification can reliably predict EOR 5 , 21 and morbidity due to insular gliomas. 21 Due to its relevance to topographic anatomy and surgical planning, the Berger–Sanai classification system will be used in the remaining of the chapter.
Type I | Anterior-superior quadrant |
Type II | Posterior-superior quadrant |
Type III | Posterior-inferior quadrant |
Type IV | Anterior-inferior quadrant |
Source: From (Sanai et al 2010). 5 |
15.5.3 The Putamen Classification (2017)
This classification is based on the work of a Chinese group that studied 211 consecutively treated patients with insular glioma and classified them accordingly to whether or not there was putaminal involvement. 37 It was proposed that the putamen, which is parallel to a large surface of the opposing insula, can stop or delay medial extension of gliomas. In contrast with the claustrum, putamen can easily be identified in high-resolution magnetic resonance imaging (MRI). In the studied group, 47% of patients were found to have putaminal involvement; these tumors were significantly larger, less likely to be associated with seizures, more likely to be IDH1 wild type, and less likely to be removed totally at surgery. In addition, the putamen classification was found to independently predict survival outcome. The binary putaminal classification scheme is survival predictor based on the singular fact of putaminal involvement. However, it should be noted that insular tumors involving the putamen have higher tumor volumes and tend to proportionally involve other adjacent structures and are also less likely to be resected.
15.6 Surgical Anatomy and Mapping Paradigms of Cortical Parcels/Subcortical Segments in Insular Surgery
15.6.1 Opercula
In insular surgery, three parcels of the frontal operculum are relevant and mapped according to their precise anatomical location. There is no consensus in the literature with regard to function and testing of specific cortical parcels and subcortical segments, and variations in practice are considerable among different institutions and teams. 39 The discussion below is based on the senior author’s practice supported by his multidisciplinary team and also a critical review of the literature. Parts of function and testing described below do not apply universally, as many of these functions are still a matter of controversy. 39
The pars orbitalis (Brodmann area 47) is the most prominent part of the inferior frontal gyrus connected anteriorly to the lateral orbital gyrus, and posteriorly it is separated from the pars triangularis by the anterior horizontal ramus of the Sylvian fissure. 40
The function of the pars orbitalis is disputed. Most evidence supports the role of the dominant pars orbitalis in semantic processing, 41 but it has also been shown to have a role in phonological processing. 42 , 43 It is therefore likely to have a role in both the processes, and recent evidence also suggests that the pars orbitalis plays a role in converging semantic and emotional aspects of communication. 44 The semantic function of pars orbitalis can be tested with semantic association tasks and word retrieval using the Boston Naming Test, and the phonological function by word generation tasks, such as asking patients to generate words beginning with a specific letter.
The pars triangularis (Brodmann area 45) is defined between the anterior horizontal (anteriorly) and anterior ascending (posteriorly) rami of the Sylvian fissure, 40 , 45 and points to the anterior Sylvian point and the insular apex. The dominant pars triangularis is involved in syntactic processing and grammar and can be tested with sentence completion and negation tasks. Some semantic functions have also been attributed to pars triangularis and can be tested with word retrieval using the Boston Naming Test.
The pars opercularis (Brodmann area 44) lies posterior to pars triangularis, and the two cortical parcels are separated by the anterior ascending ramus of the Sylvian fissure. The pars opercularis continues with a “U” connection to the inferior part of the precentral gyrus.
The dominant pars opercularis is involved in phonological processing and word generation and can be tested asking patients to generate words beginning with a specific letter. The nondominant pars triangularis and opercularis are involved in emotional intonation (I can’t believe this!) and semantic metaphorical meanings (I have butterflies in my stomach). However, these functions are not routinely tested in nondominant insular mapping. It appears that the posterior part of pars opercularis is particularly involved in speech production. 46 , 47
The inferior part of the obliquely arranged precentral gyrus (primary motor cortex or M1; Brodmann area 4) is connected at its base to the inferior part of the postcentral gyrus with another “U,” the subcentral gyrus, which lies over the transverse gyrus of Heschl of the temporal lobe. 40 Resection of gyrus of Heschl, usually required for type III insular gliomas, is not expected to result in auditory deficits. 46 The inferior part of M1, the facial motor cortex, can be resected producing a usually temporary unilateral, central facial paralysis. 38 , 46 For insular gliomas type II, resection of the inferior part of S1 may result in somatosensory deficits of the contralateral face. 46
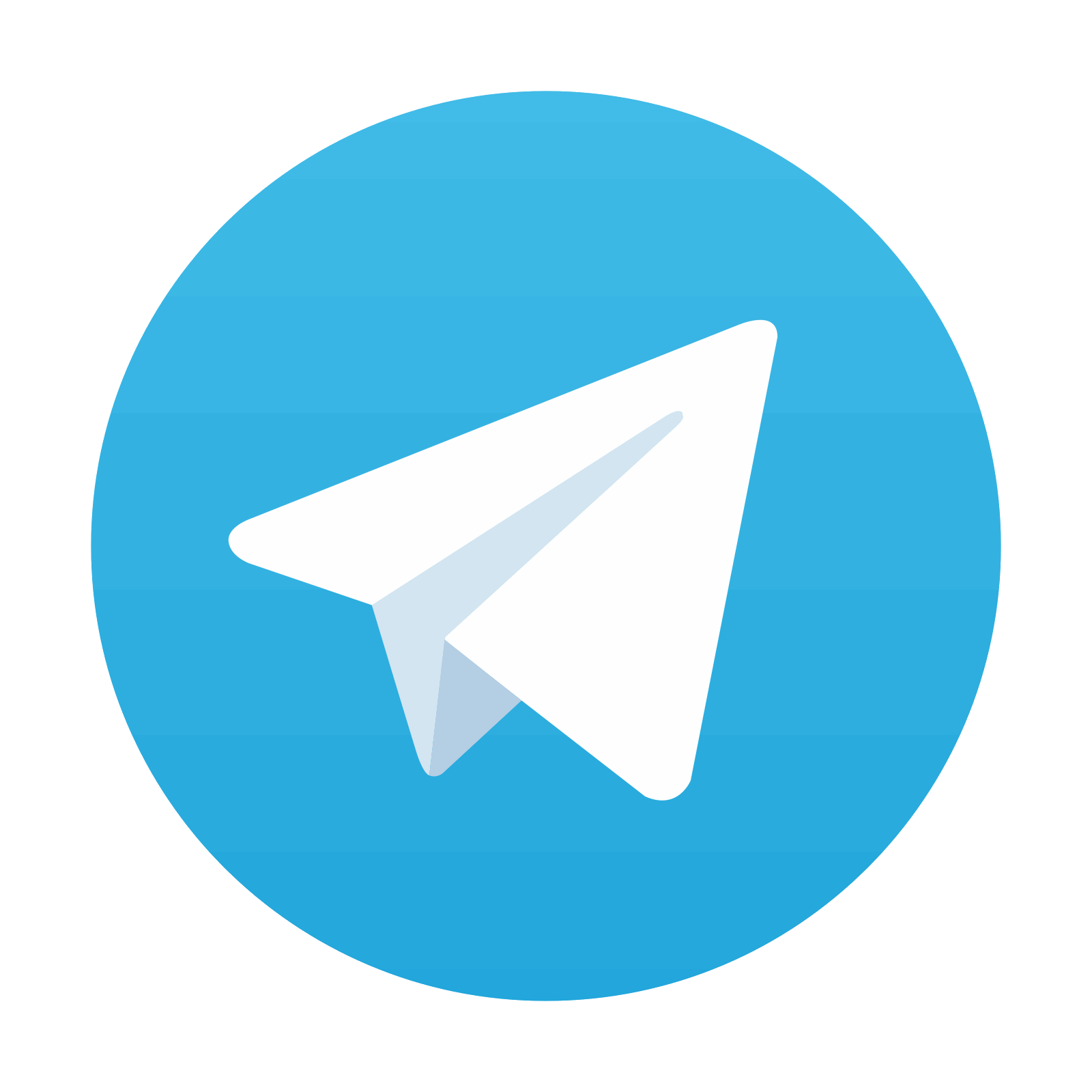
Stay updated, free articles. Join our Telegram channel

Full access? Get Clinical Tree
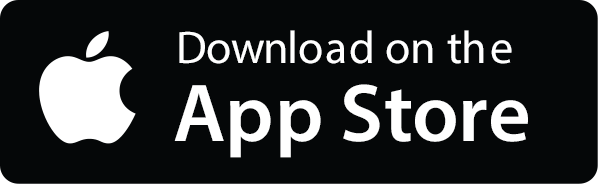
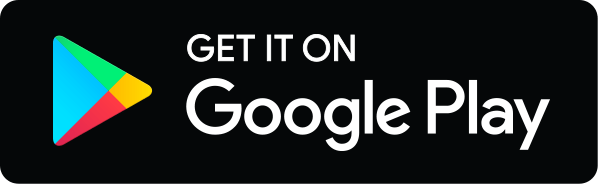
