16 Hydrocephalus shunt procedures
Adult Hydrocephalus, ed. Daniele Rigamonti. Published by Cambridge University Press. © Cambridge University Press 2014.
Introduction
Cerebrospinal fluid (CSF) shunting is one of the most common neurosurgical procedures performed, and has been used for many years with excellent results in the treatment of multiple forms of hydrocephalus in both pediatric and adult patients. However, despite many advances in shunt technology over the years, the rate of shunt failure requiring revision or replacement has remained unacceptably high. This chapter will describe many of the techniques and protocols that can be used to minimize the risk of shunt failure, with a specific focus on the surgical procedure at each anatomic location for proximal and distal catheter placement. Therefore, extended discussions of ventricular and lumbar proximal catheters, and peritoneal, atrial, pleural, and other distal catheters are included. For each shunt location, a description of our preferred surgical protocol is presented, followed by a review of the literature to discuss why we have selected our current protocols. Furthermore, the indications and complications for the different shunt locations will be addressed.
Valves
Over 100 different shunt valves are currently available from a multitude of manufacturers. Although different valve types and their characteristics will be discussed in detail elsewhere in this publication and have been reviewed in detail previously [1], they can essentially be divided into four different categories: differential pressure valves, flow-regulated valves, antisiphon valves, and adjustable valves. Differential pressure valves allow drainage once a pressure gradient across the valve is established, allowing for a linear correlation of flow rate with intracranial pressure (ICP), which can result in overdrainage in some situations such as coughing or straining that may transiently increase ICP. Flow-regulated valves allow for a constant flow rate with no dependence on ICP, although they generally have a safety mechanism that is activated at very high ICPs when their function converts to that of a differential pressure system. Additionally, flow control valves have increased flow rates with increased distance from the proximal catheter tip, so generally they need to be placed close to above the vertical location of the proximal catheter tip [2]. Horizontal-vertical valves (Integra Life-Sciences, Plainsboro, NJ), a modification of flow control valves, are used in conjunction with lumbar shunts, and include two valves for differential control of drainage in the upright or supine positions. Antisiphon valves add an antisiphon component to differential pressure valves to prevent the effects of siphoning when a patient is upright. These will be discussed in further detail below. Finally, adjustable shunt valves allow for percutaneous adjustment of the pressure setting, often with the use of a magnet. As such, some of them must be reprogrammed following an MRI and can be affected by some household magnets placed nearby, although some newer designs have locking mechanisms and don’t require reprogramming following MRI.
In general, underdrainage of CSF leads to persistence or recurrence of the initial symptoms while overdrainage of CSF leads to complications such as subdural hematomas or hygromas, overdrainage headaches, slit ventricle syndrome, or restricted head growth in pediatric patients. As such, the optimal shunt setting is usually the highest setting that still allows for the patient’s improvement. Therefore, at initial placement, we generally prefer using a midrange setting for normal pressure hydrocephalus (NPH) patients and a setting in the higher range for patients with pseudotumor cerebri (PTC). Along these lines, Bergsneider et al. [3] placed adjustable valves in 13 patients with idiopathic NPH along with intraparenchymal ICP monitors to assess the effects of patient position on ICP and determine an optimal valve setting. They found that lower pressure settings caused a significant reduction in ICP when the patient was in the supine position, which potentially explains why there can be a high incidence of overdrainage complications in patients with NPH. Additionally, based on optimizing the shunt setting with ICP monitoring, the median setting at discharge of the Codman Hakim adjustable valve (Codman & Shurtleff Inc/Johnson & Johnson Co, Raynham, MA) was 140 mmH2O (range, 30–200 mmH2O).
Valve and manufacturer choice can be of personal preference and is beyond the scope of this chapter, but we prefer adjustable valve placement as the setting can be adjusted and optimized postoperatively percutaneously, without requiring additional surgery to replace a valve with an ineffective fixed opening pressure. However, it must be noted that adjustable valves do have a significantly higher cost. Additionally, a recent retrospective review comparing Hakim differential pressure valves (NMT Neurosciences GmbH, Frankfurt, Germany) and Codman Hakim adjustable valves found no significant difference in rates of valve replacement for over- or underdrainage, although interestingly, the fixed pressure valves had a significantly lower rate of nontraumatic subdural hematomas and hygromas [4].
Antisiphon devices (ASD)
As described above, antisiphon valves are used to prevent the siphoning of CSF into the distal compartment. The different available antisiphon valves and their advantages have been reviewed in detail previously [5], but a brief discussion of these devices will be presented here.
In normal physiology, blood and CSF within the craniospinal compartment remain at the same hydrostatic level. In shunting, CSF is diverted outside of the craniospinal compartment, into the peritoneum, atrium, or pleural space. When the patient is horizontal, these spaces remain at the same hydrostatic pressure as the craniospinal compartment, but the hydrostatic pressure will change as the patient is mobilized. Therefore, the greatest pressure gradient is formed when the patient is upright, potentially leading to siphoning of CSF as the distal compartment is at a lower hydrostatic pressure than the craniospinal space. This effect is especially pronounced when distal catheters are placed in the pleural cavity, where negative intrapleural pressures occur with each respiratory cycle.
The effects of siphoning can lead to the various complications of overdrainage which have been described above. Therefore, ASDs are primarily used in management of NPH patients in the adult population to prevent the formation of subdural hematomas and hygromas, and in the management of hydrocephalus in the pediatric population to prevent the slit ventricle syndrome and proximal catheter obstruction. For instance, Gruber and Roehrig [6] found a significant reduction in ventricular catheter obstruction in pediatric patients, with proximal obstruction rates of approximately 0.2% over approximately 10 years when an antisiphon device was used. In a different study, Khan et al. [7] found a decrease in shunt obstruction from 20% to 15% over the 5-month follow-up, though this was not a significant difference.
As was mentioned above for flow-control valves, the placement as well as orientation of antisiphon devices has an effect on the performance of the antisiphon device. In general, the antisiphon device blocks drainage once the distal suction pressure exceeds the ICP. In bench studies using Delta valves with siphon control devices, it was found that CSF flow increased with increasing distance between the valve and level of the foramen of Monro [8]. In our practice, we believe that a vertical orientation of the antisiphon device placed in a retro-auricular location under the galea, approximately at the level of the foramen of Monro, has produced the optimal function of the device. This positioning has also been supported in pediatric patients [6]. However, other groups have found that placement as low as 10 cm below the foramen of Monro is optimal [9].
General surgical principles and postoperative care
At our institution, preparation for shunt insertion is performed as follows: a limited shave is performed with a clipper, if necessary, over the area of the incision. The sites of the incisions and tunneling are then scrubbed with povidone-iodine for 5 minutes, followed by application of Duraprep and draping with double layers of drapes with plastic povidone-iodine impregnated sheets overlying the areas of the incisions and tunneling path, to decrease the risk of contaminating the field. Local anesthesia, generally 0.25% Marcaine with 1/200000 epinephrine), is then injected over the course of the incisions. All patients receive a dose of prophylactic antibiotics, generally cefazolin or clindamycin (if penicillin allergy), and antibiotics are continued for 24 h postoperatively.
Additionally, all shunt procedures at our institution involve closure as follows: the valve is secured in its subcutaneous pocket using 4–0 Nurolon sutures. The wounds are then irrigated with antibiotic solution. For closure of the tissue, 0 Vicryl sutures are used for the fascia, 3–0 Vicryl sutures are used for the deep layers, and a 3–0 nylon running, non-locking suture is used for the skin.
For all routine shunt placements, patients are generally brought to the post anesthesia care unit (PACU) and admitted to the neurosurgical floor for overnight observation. A set of shunt survey X-rays is performed on all patients postoperatively as a baseline and to confirm that all connections remain intact. Additionally, a baseline head CT is obtained to verify ventricular catheter location, ascertain presence of hemorrhage, and serve as a baseline for further care. Depending on distal catheter placement site, additional imaging is obtained. For atrial and pleural distal catheter placement, a chest X-ray is obtained while the patient is in the PACU to check for pneumothorax. For peritoneal catheter placement, an abdominal X-ray is obtained approximately 12 h after the shunt survey is performed to verify movement of the distal catheter, thus confirming that the catheter is in fact in the peritoneum and not in a loculated space. Finally, an erythrocyte sedimentation rate (ESR) and C-reactive protein (CRP) are also sent for all patients postoperatively to serve as a baseline value for comparison if a later concern for shunt infection arises.
Proximal catheter placement
Ventricular catheter insertion
In general, there are three anatomical approaches that can be used for ventricular catheter placement. The frontal approach usually incorporates a burr hole overlying Kocher’s point, which is approximately 1 cm anterior to the coronal suture in the mid-pupillary line. The catheter is then placed into the frontal horn of the lateral ventricle in order to avoid the choroid plexus that arises more posteriorly. This same area of the frontal horn can be accessed from a posterior approach in the parieto-occipital region, again with avoidance of the choroid plexus. A final approach is used to target the atrium of the ventricle from a parietal burr hole, as the atrium is often the most dilated portion of the ventricular system. For the past several years we have inserted the proximal catheter using image guidance, because of our preference to use a posterior approach.
Procedure
The general procedure is illustrated in Figure 16.1. The patient is positioned depending on the location of the incision. For a frontal insertion, a curvilinear incision is made approximately overlying Kocher’s point. An additional retro-auricular or cervical incision is also often required as the frontal location requires a longer tunneling path. For a parieto-occipital placement, a single comma-shaped retro-auricular incision is made behind and above the pinna.
Figure 16.1 Ventriculoperitoneal shunt insertion. The distal catheter generally is inserted off midline, through the rectus abdominis muscle and fascia, although many other techniques can be used.
For both procedures, a 10 blade scalpel and the Bovie, in cutting and coagulator mode, are used to make the incision through the skin and galea, while preserving the pericranium. A subgaleal pocket is then made to house the valve and reservoir away from where the incision is located. A cerebellar self-retaining retractor is inserted. Next, using a perforator drill, a burr hole is made. The dura is then coagulated and cut using the Bovie knife at power of 18. The pia-arachnoid is then gently coagulated with the bipolar coagulator.
Following tunneling of the catheter from the distal site to the proximal incision, the valve is secured to the proximal and distal catheters with silk ties, and is positioned in the aforementioned subgaleal pocket. The ventricles are then again outlined using the CT-based wand and the best trajectory to enter the ventricles is again confirmed. Next, the wand, which is covered by a 9 French peel-away sheath, is advanced gently into the lateral ventricle, while confirming optimal trajectory in real time with the stereotactic system. For most patients with parieto-occipital trajectories, we cross midline with the wand to enhance communication between the lateral ventricles, maximize drainage, and minimize proximal catheter obstruction or differential ventricle collapse. Once the optimal location is found and CSF is obtained, the wand is removed, leaving the peel-away sheath in place. The appropriately sized ventricular catheter (generally 5 ± 2 cm for frontal approach and 9 ± 2cm for parieto-occipital approach), which is already connected to the valve, is then advanced into the peel-away sheath. The peel-away sheath is then stripped away. Next, 4–0 Nurolon sutures are used to anchor the new valve to the pericranium. Pumping of the valve is then performed, and the exit of CSF from the distal end is confirmed prior to insertion of the distal catheter. The incisions are then closed as described previously.
Discussion and complications
It has been demonstrated that the location of the catheter tip has a significant correlation with the rates of shunt malfunction, as a catheter tip entirely in CSF space had a failure rate of around 20%, compared to around 33% for a catheter tip only partially surrounded by CSF [10]. Wan et al. [11] studied different factors that affected optimal ventricular catheter placement in a retrospective study of 141 patients of all ages who underwent freehand catheter placement, and found that, as expected, patients with ventriculomegaly had better catheter placement. Additionally, they found that patients who underwent a frontal burr hole approach to the frontal horn had better catheter placement than patients who underwent a parieto-occipital burr hole approach to the frontal horn. Lind et al. [12] demonstrated similar results in patients over age 16 using a freehand method, as frontal catheters were found to be in the frontal horn target zone at a significantly higher rate than parieto-occipital catheters, with rates of 64% and 42%, respectively. The same group also performed a morphometric study based on imaging of normal adults and those with acute hydrocephalus to determine the margin of error of the different ventricular catheter approaches [13]. They found that the parieto-occipital placement had a significantly smaller margin of error than the frontal or parietal approaches, with mean range of successful trajectories of 8° in the sagittal plane and 11° in the coronal plane for parieto-occipital placement, compared to 42° in the sagittal plane and 30° in the coronal plane for frontal placement, and 23° in the sagittal plane and 36° in the coronal plane for parietal placement. They also found that there was no significant difference in successful trajectories with slight variations in the burr hole location. However, even with the wide range of successful trajectories with frontal placement, there was only an 86% chance of successful catheter placement.
As such, the authors advocated the use of image guidance or stereotactic navigation for catheter placement, particularly for the parieto-occipital burr hole. We agree with this recommendation at our institution and perform the majority of our adult shunts using stereotactic navigation with the Vector Vision BrainLab software (Heimstetten, Germany). All patients receive preoperative CT scans with thin (2–3 mm) slices of the brain and skull with six radiopaque fiducial markers in place. MRI can be used alternatively, but is generally more difficult and time-consuming and at a greater cost than CT. The patient is positioned in the Mayfield clamp, and a reference star is then used to register the navigation system. As described above, the wand, surrounded by a peel-away catheter, is then passed into the ventricle. This protocol was described previously by our group in the treatment of PTC, in which patients typically have small ventricles [14]. MRI guidance was used to place frontal ventricular catheters, and all catheters were successfully placed in slit ventricles with a single pass, with no shunt malfunction due to proximal obstruction found over the 24-month follow-up period. A similar setup was used by Gil et al. [15] in a small series of children with slit ventricle syndrome or small ventricles, and had no complications during surgery; additionally, none of the patients required revision of the proximal catheter during the 8-month follow-up period. A different group utilized an alternative CT-based negative system, the Stealth Station (Medtronic Sofamor-Danek), which does not require the placement of fiducial markers preoperatively. In a series of 26 adult and pediatric patients with ventricles of varying sizes, the operative time was still short at an average of 46 min, and 20 of the 21 patients had excellent catheter positioning while 1 patient had acceptable catheter positioning [16].
Although there has been success with stereotactic guidance for ventricular catheter placement, other methods have been used to improve proximal catheter location. For instance, Villavicencio et al. [17] utilized direct visualization of catheter placement using an endoscope system in which a semi-rigid endoscope was used as the catheter stylet. In a retrospective review of pediatric patients who underwent shunt placement with or without the endoscope, they demonstrated that endoscopic placement did not affect overall shunt survival, but did significantly decrease the rate of proximal catheter failure, with no increase in the infection rate. Additionally, although the use of intraoperative guidance has been used previously for insertion of ventricular catheters in infants with an open fontanelle [18], Whitehead et al. [19] adapted the procedure for use in older children with closed fontanelles. They made 1.5–2 cm burr holes that were large enough for placement of the ultrasound transducer head and catheter tip simultaneously, allowing for catheter placement under ultrasound guidance. In their series of 10 children, all catheters were placed with a single pass, postoperative CT scans confirmed good catheter placement within the ventricle, and there was no evidence of intraventricular hemorrhage postoperatively.
As mentioned above, we advocate the use of a peel-away sheath to aid with placement of the proximal catheter. This serves two functions: it prevents obstruction of the ventricular catheter perforations with brain tissue during passage into the ventricle, and it allows for passage of the catheter through the exact trajectory that the wand is passed under image guidance. The use of a similar system, though with a stylet in place of the wand in the peel-away sheath, led to a reduction in proximal ventricular catheter obstruction in the first year postoperatively from 18% to 5%, though this result was not significant due to the small sample size.
The most common cause of proximal shunt malfunction is obstruction of the catheter tip with choroid plexus and ependymal tissue [20]. This can cause additional problems during proximal shunt revision as the catheter is often difficult to remove from the ventricle, and more forceful removal will often result in intraventricular hemorrhage. Although the use of a peel-away sheath as described above can diminish the risk of occlusion of the catheter perforations with brain tissue, an alternative solution was proposed recently in which a metal guidewire inside a plastic sheathed 21-gauge needle was passed percutaneously through a Rickham reservoir and then coagulated with a monopolar in 5 s bursts to coagulate any debris or choroid plexus obstructing the catheter tip. This technique relieved the ventricular catheter blockage in their entire series of seven patients [21]. A downside to this approach is that it requires a Rickham reservoir as the reservoir access point is directly in line with the proximal catheter. We have adapted a similar approach that aids with proximal catheter removal during shunt revision or removal [22]. In our approach, intraoperatively, the proximal catheter is disconnected from the reservoir, and a stylet is passed down the proximal tubing. The stylet is then coagulated with a monopolar as described above, and the proximal catheter can then generally be removed with minimal force.
If the proximal catheter removal or placement does result in intraventricular hemorrhage, there are several approaches in management. If there is severe intraventricular hemorrhage, often placement of a ventriculostomy catheter is required. Simultaneously, if there is an adjustable shunt valve, the valve can be dialed up to the highest setting in an attempt to encourage drainage of the intraventricular blood through the ventriculostomy in an attempt to salvage the shunt. Before the ventriculostomy is removed, the shunt should be accessed to check for proximal flow, or alternatively, a radionuclide shunt patency test can be performed to verify the patency of the shunt. If a shunt revision is required, as the blood clears, the CSF can be sampled serially for protein level. We generally refrain from revising the shunt until the protein level drops below a level of 100, as levels above 100 tend to lead to shunt obstruction.
Distal catheter insertion
Peritoneal catheter insertion
The historical first-line approach for distal catheter placement has been the peritoneal cavity due to the familiarity with the anatomy, lower risk of complications, and ease of revision if needed.
Procedure
We make a paramedian incision in the upper quadrant, just lateral to the midline. The skin and subcutaneous layers are incised, and a self-retaining retractor is inserted. Next, the fascia is incised parallel to the direction of the rectus muscle fibers. The fibers of the rectus muscle are then separated bluntly, followed by incising the inner fascia of the muscle and the parietal peritoneum. When this is done, small mosquito clamps are used to hold the edges of the incision. After inserting an Olivecrona dissector in the peritoneum to verify the proper location, a purse-string suture is applied to the incision. The distal peritoneal catheter can then be tunneled to the proximal incision site. Once the valve is in place and catheters are attached and secured, the distal catheter is advanced into the peritoneal incision, and the wound is closed as described previously (Figure 16.1).
Discussion and complications
Although we typically utilize an open approach to peritoneal shunt placement due to comfort with the procedure, other groups have utilized laparoscopic insertion of the peritoneal catheter, either alone or in conjunction with general surgeons. For instance, Stoddard and Kavic [23] described an approach in which a small incision was made for passage of a Veress needle, and a separate small incision was used to place a trocar to pass an endoscope. The distal catheter was then passed into the peritoneal cavity with a Hickman introducer kit. This offered the advantage of a less invasive incision, as well as visual confirmation of distal shunt placement and drainage of CSF from the distal catheter. Furthermore, due to the smaller fascial incision, there is theoretically a lower risk of distal catheter withdrawal and hernia formation, though this has not been studied in detail yet. Other variations of this technique using a single trocar and access hole have been described as well [24,25].
Although the most common complications result from distal catheter infection, obstruction, or fracture, and management of these is described elsewhere in this chapter, a multitude of other more obscure complications have been documented in isolated case reports. Some of these complications include volvulus [26], bowel obstruction [27], inguinal hernia or hydrocele [28], pleural effusion/hydrothorax [29], or migration and erosion into other organs such as the bladder [30] and heart [31].
Atrial catheter insertion
Placement of the distal catheter in the right atrium is often a second choice following complications with peritoneal placement or when peritoneal placement is contraindicated. For instance, it is often pursued in patients who have undergone multiple prior abdominal surgeries or peritoneal shunt placements with significant adhesions, have severe ascites, prior peritonitis, frequent distal catheter obstruction or malfunction, or when body habitus precludes peritoneal shunt placement. Although the internal jugular vein has traditionally been accessed with a venous cut-down procedure, many groups, including our own, have moved to a percutaneous ultrasound-guided approach. The average percutaneous procedure was 22 min faster than the cut-down procedure [32].
Procedure
Our approach has been described in detail previously [32], and is illustrated in Figure 16.2. The patient is placed in slight Trendelenburg position to minimize the risk of air embolism. The optimal location of the incision is determined using ultrasound to visualize the carotid artery medially and the internal jugular vein laterally prior to preparation of the skin and draping. A 1 cm incision is then made over the vein using a 10 blade scalpel and the Bovie in coagulator mode through the skin and underlying soft tissues. Under ultrasound guidance, an introducer needle attached to a syringe is then passed, medial to the sternocleidomastoid muscle edge and pointing slightly laterally, into the vein while gently aspirating venous blood. The syringe is then removed and the straightened “J” tip of guidewire is then inserted into the needle and advanced until some electrocardiographic changes are noted, at which point it is pulled back slightly. The needle is then removed and the guidewire is anchored. At this point, the distal catheter is tunneled to the proximal incision and connected to the proximal valve system prior to proceeding, with confirmation of distal CSF flow obtained.
Figure 16.2 Ventriculoatrial shunt insertion. The distal catheter is introduced through the internal jugular vein (IJV), with a goal termination at the cavoatrial junction. SVC, superior vena cava.
Next, the vessel dilator and sheath introducer are passed together over the guidewire using a rotational motion. The length of the distal catheter is calculated as described previously [33], using a preoperative chest X-ray. The guidewire and vessel dilator are then removed from the peel-away introducer, and the atrial catheter is then passed through the peel-away introducer into the superior vena cava. At this point, the peel-away introducer is stripped away and gentle pressure is applied over the neck incision to stop the venous oozing. The incision is then closed as described previously.
Discussion and complications
The main complications occur from the development of a fibrous capsule and eventually occlusion of the distal catheter or vessel thrombosis if the catheter length is too short [34], or risk of intra-atrial clot formation and subsequent shunt occlusion, pulmonary embolism, or congestive heart failure if the catheter is too long. As such, we have adopted our approach as outlined above. Using this approach with preoperative chest X-ray measurement, 14 patients in the series had catheter placement within 0.4 cm of the cavoatrial junction, and no patients required distal revision for a malpositioned catheter tip [34]. Other groups have used intraoperative transesophageal echocardiography to confirm distal catheter location with similarly positive results [35], although the patients are subjected to a more invasive echocardiogram with inherent risks of its own. Typically, duplex ultrasound of the internal jugular vein is obtained preoperatively to ensure patency for passage of the distal catheter; however, if difficult placement is encountered intraoperatively due to anatomical variations, thrombosis, or large venous valves, we have previously used intraoperative venography to navigate around the obstruction [36]. There is an additional risk of pneumothorax or carotid artery cannulation, though this risk is mitigated with a postoperative chest X-ray and the use of ultrasound guidance during venipuncture.
Pleural catheter insertion
Placement of the distal shunt in the pleural space has been considered a third-line treatment in most situations when peritoneal or atrial placement is contraindicated, due to the concern for pneumothorax and pleural effusion from drainage of CSF. Pleural shunting generally has a siphoning effect due to the negative intrapleural pressure, although this is mitigated with the use of some of the newer antisiphon devices.
Procedure
Our approach is demonstrated in Figure 16.3. A curvilinear incision is made laterally to the breast, centered on the anterior axillary line and following along the course of the underlying rib (often the seventh). The skin and subcutaneous layers are incised. The most anterolateral attachment of the fascia (of the serratus anterior muscle) is then incised, and the attachments of the external intercostal muscle, intermedius intercostal muscle and internal intercostal muscle are separated from the upper margin of the rib using the Bovie knife. The parietal pleura is then visualized and the lung can be observed moving underneath it with each respiratory cycle. A tunneler is then used to pass the distal catheter from the proximal incision to the chest incision. The distal end of the new catheter is inserted into the pleural cavity, with closure as described above.
Figure 16.3 Ventriculopleural shunt insertion. The distal catheter is introduced above the sixth rib in order to avoid the intercostal vessels and nerve.
Discussion and complications
Tension hydrothorax is the most severe complication following placement of a pleural distal catheter [37]. Following a shunt malfunction or decreased drainage of CSF from the pleural space, fluid accumulation can occur generally subacutely, leading to tachycardia, hypotension, desaturation, and eventually cardiopulmonary collapse if not recognized. Treatment is with emergent chest tube placement to decompress the fluid, and often shunt externalization and distal revision once the patient is stabilized. As such, a patient with a pleural distal catheter with any concern for malfunction should have an urgent chest X-ray.
Other distal catheter locations
In cases of shunting where distal catheter failure has occurred at the above locations, other locations have been utilized, including gallbladder placement, as reviewed in detail previously [38], and ureteral placement, although these locations have had mixed results and are often considered end-stage locations. These methods are exceedingly rarely used at our institution.
Lumbar catheter insertion
Lumbar shunts have been used in the treatment of communicating hydrocephalus, pseudomeningocele, and CSF leaks, but are predominantly used in the treatment of PTC, and are contraindicated in the treatment of obstructive hydrocephalus.
Procedure
Our approach is illustrated in Figure 16.4. The patient is positioned to minimize the risk of injury during surgery on a bean bag in a lateral position, as is done to perform a spinal tap. The first incision is made in the midline in the lumbar region, and the fascia is exposed. A special Touhy needle is then advanced between two adjacent spinous processes into the subarachnoid space, until good return of CSF is obtained. After removal of the stylet, the proximal catheter is advanced in the subarachnoid space for at least 5 cm and the Touhy needle is withdrawn. Next, the catheter is tunneled to a separate incision in the flank, where a special pocket is created in the subcutaneous tissue to accept the valve. A third incision is made in the abdomen for the distal shunt. The proximal and distal catheters are then connected to the valve, and a silk tie is used to secure the connections. The catheter can then be tunneled to its intended destination, with wound closure as described above.
Figure 16.4 Lumboperitoneal shunt insertion. The proximal catheter is often introduced at the L3–4 level, although adjacent levels are also used commonly.
Discussion and complications
Lumbar shunting holds some advantages over ventricular catheterization, including a decreased risk of parenchymal injury as no catheter is passed through the brain. Additionally, since drainage occurs through the lumbar cistern for communicating hydrocephalus, drainage occurs symmetrically throughout the entire ventricular system without the risk of unilateral ventricular collapse leading to shunt malfunction that can be seen with ventricular catheters. Furthermore, there is some evidence that lumbar shunts are associated with a lower rate of infection than ventricular shunts [39]. Finally, lumbar shunts are often easier to place than ventricular shunts, particularly in PTC patients who typically have small slit ventricles. Additionally, a lower rate of subdural hematomas and hygromas has been noted with lumbar catheter placement compared to some of the series examining ventricular catheter placement [40,41]. Finally, lumbar shunts are believed to have a lower risk of siphoning as the distal catheter is typically at a similar height as the proximal catheter.
However, lumbar catheter placement is not without complications. Catheter obstruction is the predominant complication that has been noted with lumbar shunt placement, and was the cause of 65% of the revisions in one series [40]. Additionally, lumbar shunting may lead to overdrainage causing intracranial hypotension. Overdrainage was responsible for 15% of the revisions in a series [40], although this could be alleviated somewhat with the use of adjustable valves. This overdrainage has even led to an increased risk of Chiari malformations in the pediatric population [42,43], resulting in a 4% rate of suboccipital decompression for symptomatic Chiari. However, this complication does not seem to be as significant in the adult population [40,41]. Furthermore, lumbar radiculopathy and back pain has been noted in patients with lumbar shunts, and was responsible for 4.5% of the revisions in a series [40]. Also, although lumbar catheterization is generally less invasive than ventricular catheterization, patients with lumbar stenosis often require a partial laminectomy in order to pass the catheter in the subarachnoid space. There is an additional risk of spinal cord injury or extra-axial hematoma formation, though these complications are rarely seen with proper technique. Another major drawback of lumbar shunting is that the shunts are often inserted without a reservoir, or the reservoir is difficult to access given the patient’s body habitus; thus, it is much more difficult to assess shunt function with a shunt tap or radionuclide shunt patency study, and opening pressure often must be assessed with a lumbar puncture. This is especially problematic in PTC patients who frequently present with worsening headaches and concern for shunt malfunction. Finally, in a series of 76 patients who underwent lumbar shunt placement, a complication of lumbar shunt placement with a horizontal-vertical valve included malposition of the valve, as it requires the components to be in a horizontal and vertical orientation for proper functioning [41]. This complication was addressed by suturing the valve in place, although this method is not always foolproof especially in PTC patients who tend to have a larger body habitus.
There is not a consensus on which type of shunt to use for communicating hydrocephalus, although many groups advocate the use of lumbar shunting for PTC, particularly given their typically difficult-to-access slit ventricular system. Although we previously demonstrated that ventricular catheterization could be reliably performed under image guidance for PTC patients [14], we have progressed to generally using lumbar shunts in this patient population due to the greater ease of this procedure and minimization of siphoning effects. Tarnaris et al. [44] compared the outcomes of ventricular versus lumbar catheterization in PTC patients, and found similar outcomes in visual symptoms and headache improvement between the two locations, with no significant difference in the length of time of catheter patency. However, Abubaker et al. [45] found a higher revision rate of lumbar shunts compared to ventricular shunts. Similarly, McGirt et al. [46] showed that lumbar shunts had a 2.5-fold increase in shunt revision and 3-fold increase in shunt obstruction compared to ventricular shunts. Based on the conflicting data, it is difficult to make a final recommendation regarding the ideal shunt type for PTC, though if ventricular catheterization is selected, it should always be performed under image guidance.
Shunt infections
Shunt procedures have a high rate of infection, with a recent review of the literature documenting an average infection rate of 5.4% [47], although an older study documented an infection rate of almost 10% [48]. In general, shunt infections are found within the first 4 months postoperatively [49,50], although other studies have defined shunt infection as those occurring within the first 6 months postoperatively [51]. Shunt infections are suspected when there is evidence of meningismus or ventriculitis, erythema or inflammation along the catheter tract, or new discharge from or dehiscence of the wound. Additional abdominal findings including pain or distension, cysts or collections of CSF can be indicative of shunt infection.
As reviewed previously [52], shunt infection likely arises from bacterial colonization of the hardware intraoperatively, potentially from contact with the skin or skin edges. As the shunt is a foreign body, some of the normal immune response is blunted and eventually a biofilm develops on the shunt hardware, reducing or eliminating response to antibiotic therapy without hardware removal. The majority of shunt infections are caused by Staphylococcus species, although species such as Enterococcus, Pseudomonas, Haemophilus influenzae, Escherichia coli, and several other species, can cause infection, as described in a prior review [47].
To decrease the infection rate, Choux et al. [51] developed a shunt insertion protocol in which procedures were completed in under 40 min, presterilization packages were opened just prior to implantation and shunt materials were immersed in a gentamicin bath, a minimal area needed to complete the procedure was exposed under adhesive sterile drapes, and the abdominal dissection was completed first to decrease the amount of time that the cranial incision was open to air. Although these factors were not assessed individually, the authors were able to decrease their infection rate from 7.75% to 0.17% using this protocol. Faillace [53] developed a similar protocol for shunt insertion and decreased the shunt infection rate from 9.1% to 2.9%. Some modifications of that protocol included the same scrub team was kept throughout the case, traffic in and out of the operating room was limited during the procedure, and all staff received in-service education on the protocol. Additionally, preoperative vancomycin and gentamicin were given and continued 48 h postoperatively, a wide shave and 5 min scrub with povidone-iodine scrub solution was performed followed by povidone-iodine painting, two layers of iodine-impregnated adhesive sheets and a double layer of drapes were applied to avoid contact with the skin as much as possible, and wide draping was used as a barrier between the surgical field and the anesthesia area. Furthermore, Faillace [53] utilized a no-touch technique in which all personnel double-gloved, contact with the skin edges was avoided, the shunt materials were handled minimally and only with forceps, a separate table and instruments were used for handling the shunt after skin incision, and the equipment was opened immediately before use and placed in a bacitracin solution. At our institution, we utilize and advocate a very similar protocol, with modifications of using Duraprep rather than povidone-iodine painting, and preoperative cefazolin or clindamycin, given within 1 h of incision, and continued for only 24 h postoperatively.
In terms of perioperative antibiotics, we advocate the use of perioperative antibiotics for 24 h only, as described above. Along these lines, for ventriculostomy placement, there was no difference in infection rates between patients receiving 24 h of antibiotics starting immediately prior to placement compared to patients receiving antibiotics for the entire time the drain was in place. Additionally, Ratilal et al. [52] performed a meta-analysis of the literature and found no support for continuing prophylactic antibiotics for more than 24 h postoperatively.
An additional important method for shunt infection control has been the development of antibiotic-impregnated shunts (AIS), in which the proximal and distal shunt catheters are impregnated with antibiotics. The AIS in use at our institution is the Bactiseal (Codman, Johnson & Johnson, Boston, MA), which is impregnated with 0.54% rifampicin and 0.15% clindamycin. The predominant function of the AIS is prevention of bacterial adherence and colonization of the shunt hardware.
In a retrospective analysis of pediatric patients at our institution, Sciubba et al. [54] compared the infection rates of Bactiseal AIS catheters versus non-AIS catheters, and found that AIS catheter use significantly decreased the infection rate, from 12% to 1.4%, over the 6-month period of follow-up. In a similar retrospective analysis of adult patients at our institution, Farber et al. [55] also demonstrated a significant decrease in infection rate with Bactiseal AIS catheter use, decreasing the infection rate from 4.0% to 1.2%. However, a different series of 258 adult and pediatric patients found no significant difference in infection rate between AIS and non-AIS catheters, although their baseline infection rate with AIS catheters was somewhat higher than has been encountered at our institution [56]. One concern with the use of AIS has been the selection of resistant organisms, but similar rates of oxacillin-resistant S. aureus infections were noted when using the AIS compared to regular catheters [57].
Once shunt infection is encountered, intravenous antibiotics are usually insufficient to eradicate the organism, and revision or replacement of the shunt hardware is required. A common practice in the treatment of shunt infection is shunt externalization, near the clavicle or cranially, with external drainage and initiation of intravenous antibiotics. CSF is then sampled serially until negative cultures are obtained, at which point the entire shunt system is removed and replaced. Patients are often continued on a prolonged course of intravenous antibiotics postoperatively as well. This treatment often results in a prolonged hospital stay and a significant expense. For instance, Attenello et al. [58] found that in pediatric patients with an admission for shunt infection, the mean hospital stay was 12 days, an average of 3 shunt surgeries, 4 CT scans, and 6 X-rays were performed, and an average antibiotic course of 3 weeks was prescribed, all at a mean hospital cost of almost $50 000 per patient. Similarly, a study of shunt infection in adult patients found a mean hospital cost per shunt infection of $40 000 [59]. Although the AIS catheters have a significantly higher initial cost, the direct cost savings from a lower infection rate have been estimated to range from $25 000 per 100 patients [60] to $50 000 per 100 patients [59]. Based on all of these results, we strongly recommend the use of AIS catheters in shunt surgery.
Conclusion
Although there has been significant success in optimizing shunt placement in order to decrease the rates of shunt failure, clearly there is still work to be done in this arena as there is still a non-negligible rate of shunt failure. As such, along with encouraging the development of new and more advanced shunt technology, we must strive to continue to revise and optimize our surgical procedures and management of hydrocephalus.
References



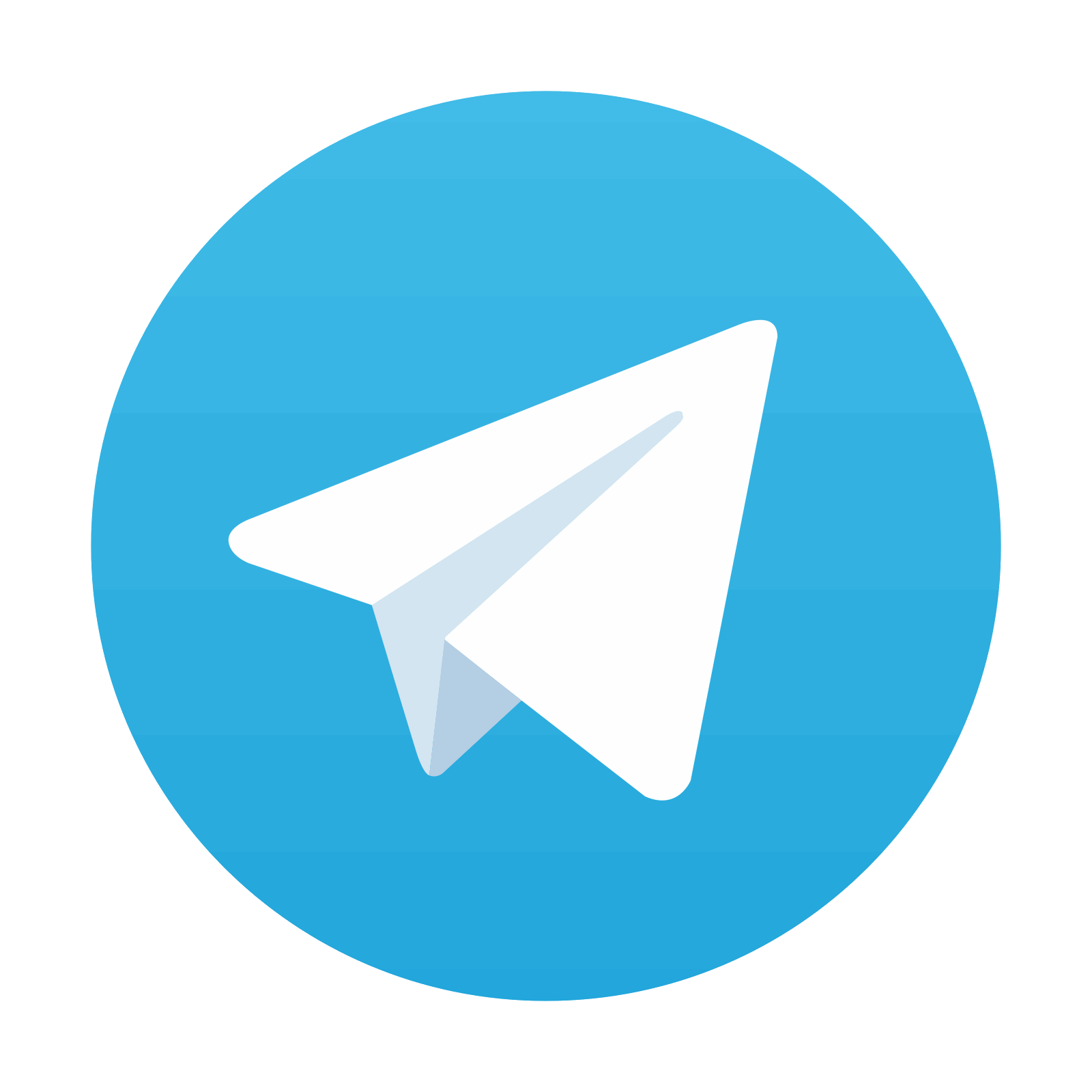
Stay updated, free articles. Join our Telegram channel

Full access? Get Clinical Tree
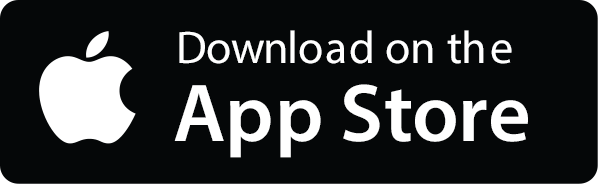
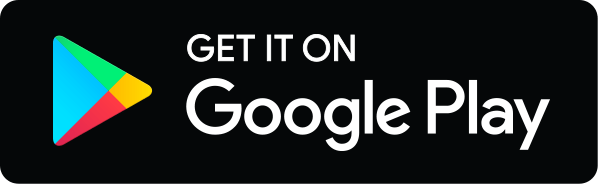
