16 Nutrition
Abstract
Nutritional management is medical treatment. Nutrition should be considered equal to all other medical managements, for its proper use can optimize nervous system recovery and prevent further injury. Once the brain is injured, even to the point of coma, brain metabolism is increased and should be treated as early as possible. Withholding nutrition is dangerous, leading to decreased gastrointestinal mucosa integrity, depression of the immune response, and other abnormalities, which in turn leads to increased morbidity and mortality. The amount of nutrition, the type of nutrition, the additives to the nutrition, and the method of delivery of the nutrition are specific for patients with central nervous system injuries.
Case Presentation
A 60-year-old man (154 lb [70 kg], 5 ft 5 in [166 cm]) was in a motor vehicle accident and suffered a traumatic subarachnoid hemorrhage. His Glasgow Coma Scale score is 7. The patient was in the neurosurgical intensive care unit for 2 days, intubated, with a heart rate of 90.
See end of chapter for Case Management.
16.1 Nutritional Requirements
Only half of neurologic injury occurs at the time of the ictus, with the remaining damage occurring as secondary damage. The biggest impact on avoidable exacerbations can be made in the neurosurgical intensive care unit (NICU). The survival for patients with severe head injury depends largely on critical management, including, but not limited to, oxygenation, blood pressure, intracranial pressure/cerebral perfusion pressure, and optimal nutrition. 1
Adequate nutrition is critical to maintaining internal systems, meeting the metabolic demands of increased activity in illness, and helping in the repair and healing of wounds. Considering all the secondary insults occurring in the patient while in the NICU, nutrition may play an even larger role than once imagined, especially if nutrition is considered not as an adjunct but as a necessary therapy. 2 , 3 , 4 Supplements, such as vitamin C, zinc, selenium, and multivitamin concentrate infusions (MVIs), among others, are also necessary to promote wound healing.
Nutritional management of the neurosurgical patient is a high priority in the NICU setting. Central nervous system (CNS) injury can stem from trauma, metabolic disorders, stroke, ischemia, neoplasm, and neuromuscular dysfunction, all of which in the acute stage can significantly increase metabolic needs. Adequate nutrition is critical to sustaining body systems, meeting the increased metabolic demands of illness, and supporting healing. Yet nutritional support has not been considered a primary treatment modality; subsequently the incidence of malnutrition in ICU patients may be as high as 50%. 5 Thus, in all admissions to the NICU, nutrition must be promptly and adequately addressed in the management of patients with CNS injury.
Contrary to what was once thought, after neurologic injury there is a severe profound systemic hypermetabolic reaction that results in the rapid depletion of whole-body energy stores. If not attended to, a catabolic injury cascade results in the loss of gastrointestinal (GI) mucosa integrity, reductions in body muscle mass, reductions in systemic protein stores and synthesis, and the compromise of both humoral and cellular immune competence. 6 Nutrition can help the patient with neurologic injury fight infections, improve ventilator function, and promote wound healing.
16.2 Undernutrition
Inadequately feeding patients results in the following:
Loss of GI mucosa integrity.
Reductions in body muscle mass.
Reductions in systemic protein stores and synthesis.
Compromise of both humoral and cellular immune competence.
Decrease in the absolute number of circulating T cells.
Promotion of cutaneous anergy.
Depressed production of new antibodies in response to a novel antigen.
Suboptimal nutrition is dangerous; it has been shown to decrease the absolute number of circulating T-cells, promote cutaneous anergy, and depress the production of new antibodies in response to a novel antigen. The depression of the immune response caused by undernutrition can significantly increase the probability and virulence of infectious complications in surgical patients.
Energy stores and fat stores allow the body to function for a long time without devastating effects. The earliest studies show that a 30% preoperative weight loss led to a 10-fold increase in morbidity and mortality of gastric surgery. By extrapolation, using fuzzy logic, there is the assumption that a 15% weight loss in the bedridden patient is of little consequence, bearing in mind that a 30% weight loss is potentially very deleterious. Nutritional problems are usually discussed only after visual signs of weight loss, which is the reason nutrition during the first several days to as much as 2 weeks has not been studied. Three randomized class I studies have evaluated the relationship between caloric intake and patient outcomes. 7 , 8 , 9 , 10 Rapp et al 9 showed that the consequence of severe undernutrition for a 2-week period after injury was an increased mortality rate when compared to those individuals that had full caloric replacement by 7 days. In Young et al’s study, 10 there was no difference in those patients that were fed parenterally at 3 days when compared to those fed enterally at 9 days.
It is necessary to know how to determine weight in order to determine if there is weight loss. The ideal body weights for people with a medium frame are the following:
Men: 106 lb. for 5 ft, then add 6 lb for each additional inch.
Women: 100 lb for 5 ft, then add 5 lb for each additional inch.
For both: Subtract 10 lb for small frame and add 10 lb for large frame.
When protein calorie malnutrition occurs there is a depletion of skeletal muscle and visceral muscle in a 30:1 ratio. The measured level of the serum protein albumin can approximate visceral muscle stores. Mortality risk increases by about 37% for each gram deficit of serum albumin. Decreased albumin is also responsible for altering colonic resorption of water and salt, manifested by edema and ascites, and it causes gastric stasis, prolonged small bowel retention, delayed wound healing, and increased wound infection. When a patient has not been fed or when a patient has been hypermetabolic because of neurologic injury, a nutritional blood test panel should be obtained that includes albumin, prealbumin, transferrin, total iron-binding capacity, and serum creatinine. To further help with nutritional assessment there should be strict monitoring of total input and output and daily weights (► Table 16.1).
Nutrition must not be thought of as preventing large stores of excess fat from being lost but as a form of therapy. At the same time nutrition must not be haphazardly given, for it has side effects just as any drug does. The nutritional formula must be beneficial and should aid in treatment of the neurologically injured patient.
16.3 Hypermetabolism following Central Nervous System Injury
Patients have an inflated hypermetabolic response to neurologic injury. Hadley et al 7 , 8 demonstrated a mean resting energy expenditure 46% above the normal predicted basal metabolic rate in a study of 45 head injury patients. Most other investigators who measured metabolic expenditure in the rested comatose patient also demonstrate a metabolic rate that is approximately 120 to 250% of the predicted normal basal metabolic rate. During these times, in order to meet the needs of the increased energy production, there is a marked increase in gluconeogenesis, hepatic protein synthesis, and use of protein, carbohydrate, and fat. The increased use of fats, carbohydrates, and proteins not only makes supplying nutrition difficult, it accelerates the development of malnutrition in patients who are not being fed. In the CNS-injured patient there is a change in the ratio of nutrients required; there is an increased demand for protein and lipid calories and a relative decrease in carbohydrate needs. These requirements must be taken into account when designing a nutritional formula for the CNS-injured patient.
The basic requirements in neurologic injury include the following:
Increased metabolism.
Increased protein.
Increased lipid.
Decreased carbohydrate.
The adaptive response to CNS injury is an increase in catecholamines, cortisol, and glucagons and a subsequent increase in metabolism and hyperglycemia. 8 Both gluconeogenesis and lipolysis continue. Even with hyperglycemia, 75 to 90% of energy is still supplied by fat oxidation. In moderation, fat oxidation is not a major problem; however, when there is decreased blood return and continued fat oxidation there is free radical production and further neurological damage. Although protein is required for the repair of injured brain, the changes in catecholamines, cortisol, and glucagon lead to proteolytic metabolism. Catecholamines are released and in turn stimulate the release of adrenocortictropic hormone, growth hormones, glucagon, and insulin. Catabolic hormones, such as glucagons, cortisol, and catecholamines, cause use of alternate energy sources when there are no further nutrients supplied. As the injury continues, stress increases and proteolysis persists, making it impossible to achieve a positive nitrogen balance in the early period after CNS injury. Even if CNS-injury patients are paralyzed or put into barbiturate comas, they still require 100 to 120% of the resting energy expenditure. 11 A major part of the increase in resting energy expenditure in comatose patients is due to muscle tone. 12
The following are normal responses to CNS injury:
Increased catecholamines.
Increased cortisol.
Increased glucagons.
Proteolysis.
Gluconeogenesis.
Lipolysis.
Increased adrenocortictropic hormone, growth hormones, and insulin.
In addition to the loss of protein stores throughout the body, injury results in other changes, such as electrolytes being lost from GI tract secretions. Just as protein stores must be replaced, electrolytic losses must be replaced in nutritional therapy (► Table 16.2). 2
GI Loss | Sodium mEq/L | Chloride mEq/L | Potassium mEq/L |
Gastric | 60 | 100 | 10 |
Bile | 140 | 100 | 10 |
Pancreas | 140 | 75 | 10 |
Small bowel | 100 | 100 | 20 |
Diarrhea | 60 | 45 | 30 |
16.4 How Much Nutrition Is Needed?
The average normal calorie requirement is 30 kcal per kg per day for a Glasgow Coma Scale (GCS) score>12, 35 kcal per kg per day for a GCS score from 8 to 12, and 40–45 kcal per kg per day for a GCS score from 3 to 7. However, indirect calorimetry performed with the aid of a portable metabolic cart once a day for the first several days is the most accurate way to determine the patient’s resting energy expenditure and nutrition requirements. This method is unnecessary since the general energy requirements are known using the Harris–Benedict formula (► Table 16.3), although the Harris–Benedict equation contains systematic error of 5 to 15% overestimation.
Clifton et al 13 simplified the adjustment factors correcting the Harris–Benedict overestimation. They showed that the required percentage of resting energy expenditure of total calories calculated from part I of the Harris–Benedict formula can be estimated accurately using GCS score, heart rate, and days since the injury. The Clifton equation is

In the first 2 weeks after injury energy expenditure rises regardless of neurological course, but its extent beyond this is unknown. The hypermetabolic response is also prolonged in patients who have other major organ system trauma. This hypermetabolism can be exaggerated for up to a year in severe head injury patients; however, this should not be thought of as the norm.
In the neurologically injured patient the resting energy requirements will be supplied by muscle breakdown or other body stores of proteins, fats, and carbohydrates (► Table 16.4).
Substrate providing calories | Kcal per gram |
Muscle | 1 |
Carbohydrate | 4 |
Protein | 4 |
Fat | 9 |
Initially in the neurologically injured patient, the hypermetabolic state often is combined with undernutrition and compromising protein synthesis, resulting in lean muscle mass catabolism for essential amino acids. As the hypermetabolism hypercatabolic process continues, nitrogen is lost through the urine and feces. There is essentially no nitrogen gained through normal levels of nutrition, and a great deal of nitrogen is lost, leading to a negative nitrogen balance. For each gram of nitrogen in the urine and feces, 6.25 grams of protein have been catabolized. Nonfed patients with severe brain injury continue to lose 14 to 25 grams of nitrogen per day. The maximum excretion occurs during the second week. The average nitrogen loss of a head injury patient is 0.2 grams of nitrogen/kg/d, which will result in a 10% loss of lean mass in 7 days. 8 This is about double or triple the loss in the normal person. Unlike the Studley paper that showed that a 30% preoperative weight loss led to an increased morbidity and mortality of gastric surgery by 10-fold there are no studies that examine nitrogen loss or nitrogen replacement with outcome; therefore nitrogen balance may not be a major issue. Nonetheless, a study by Clifton et al 14 examined two matched groups of comatose head injury patients and determined that the level of nitrogen intake should be 20% of the core composition of a 50 kcal/kg/d feeding protocol. For the hypermetabolic patient 20% is the maximal protein content of most enteral feedings and 20% is the maximal amino acid content of most parenteral formulations.
Any feeding has to take into consideration fluid requirements, which averages 35 mL per kilogram per 24 hours, more in athletes and less in the elderly. The following is an additional way to measure normal fluid requirements (average 35 mL/kg, elderly 30 mL/kg):
100 mL per kilogram for the first 10 kg in 24 hours then
50 mL per kilogram for the next 10 kg in 24 hours then
20 mL per kilogram for remainder weight in kg in 24 hours age < 60
50 mL per kilogram for the next 10 kg in 24 hours
The protein content of the fluid should be 1, 1.5, or 2 kcal/mL in order to meet partial nutritional needs and to continue a fluid euvolemic state. There are many physiological circumstances that require increased fluids from the normal amount. Fever increases fluid requirements by 12.5% for each 1°C above normal, sweating 10–25%, and hyperventilation 10–60%. 15
Fluid types must also be used judiciously. The deleterious effects of hypotonic intravenous solutions on brain injury have been known since 1919; 16 therefore, immediately after neurological problems, intravenous hypotonic fluid such as lactated Ringer’s (Na = 130 mEq/L; 273 mOsm/L), dextrose 5% in water, and 0.45 normal saline should be avoided. Decreasing intravenous fluids 1 mEq/L decreases osmotic pressure 38.6 mm Hg 16 and can lead to brain edema. On the other hand, it is also known that hypertonic saline solutions lower intracranial pressure. 17 , 18
There have been multiple class I investigations of the amount of feeding, the route of feeding, the use of steroids, and nitrogen balance, but none of these studies have looked at patient outcome or complications. Even the evidence of what to feed the CNS-injured patient remains unclear. The formulas must be specifically tailored; even the ingredients once thought to be the foundation of nutritional therapies need to be reexamined. High osmolar total parenteral nutrition (TPN) increases brain edema after cryogenic injury in animals 19 because of changes in serum osmolarity. The increased edema does not occur with lower osmolarity TPN. Besides hyperosmolar nutrition causing worse outcome after CNS injury, hyperglycemia is well recognized as worsening outcome after CNS injury and must be avoided. 20 , 21 , 22 , 23 It has even been suggested that hypoglycemia may be neurocytoprotective. 23 , 24 Hyperglycemia is common in trauma patients even without supplementation and is exacerbated by the administration of any TPN, hyper- or iso-osmolar. 10 Therefore, TPN should not be given during the first 48 hours after CNS injury, and serum glucose concentrations must be closely monitored and treated vigorously and kept below 110 mg/dL. 25 , 26
Nutritional treatments should not deliver less than the minimal daily requirements, and as nutritional science matures nutritional requirements in specific diseased states will be developed.
16.5 The Gut Benefits from Nutrition
Early nutritional support increases CD4 cells, the CD4:CD8 ratio, and the T-lymphocyte responsiveness to concanavalin-A, which assists immune function in patients sustaining CNS injury. 27 For nutrition delivery to the body to optimally occur in a physiological manner, the GI tract cells must be functioning. A functioning GI tract is necessary to protect against infection and sepsis from its own bacteria, particularly after neurologic injury or surgical stress. If the gut is maintained there will be no bacterial overgrowth, no proliferation of specific pathogens within the bowel, and no penetration of the pathogens into the bowel wall. Furthermore, with nutrition, the GI tract will remain the principal organ in the regulation of interorgan amino acid exchange, with glutamine being the most important amino acid available to the GI cells. After CNS injury, the GI tract cells’ uptake of glutamine increases despite decreased oral intake and decreased delivery of glutamine to the intestinal mucosa. Glutamine is the most abundant amino acid in the blood, possibly because it is a precursor for purines and pyrimidines. When there is no added nutrition available, glutamine must come from another source; it originates from the muscle catabolism.
In summary, the primary benefits of maintaining GI mucosa glutamine nutrition are as follows:
Protects against infection and sepsis from its own bacteria.
Prevents the proliferation of specific pathogens within the bowel.
Enables the GI tract to remain the principal organ in the regulation of interorgan amino acid exchange.
Enables protein production.
Enables nitrogen transport and ammonia excretion.
Prevents muscle degradation.
Decreases hypermetabolism.
In undernutrition, muscle undergoes proteolysis and releases essential glutamine into the circulation, supplying the basic requirements for GI enterocytes and for renal aminogenesis. However, the GI cells quickly use the glutamine from the muscle, thereby decreasing the circulating glutamine level available to other cells of the body for protein production, nitrogen transport, and ammonia excretion. In functioning cells throughout the body, glutamine is catabolized by mitochondrial glutaminase to glutamate and ammonia, and once released into the circulation and across the blood–brain barrier, it can destroy brain cells. However, not all glutamine by-products are neurotoxic; alanine is used by the liver for gluconeogenesis, and ammonia is converted to urea. Circulating glutamine is necessary for the gut to prevent infection, necessary to cells for protein synthesis, important for nitrogen and carbon transport and for ammonia excretion, the major substrate for gluconeogenesis, the substrate for renal angiogenesis, and a fuel source for rapidly replicating cells, but too much glutamine can be a problem. Although the by-products of glutamine metabolism can cause neurological problems, there is no evidence that an exogenous supply to the gut increases neurologic injury when the kidneys and liver are functioning. Therefore, the net overall requirement is for glutamine to be provided during CNS injury as soon as possible at appropriate levels to maintain homeostasis.
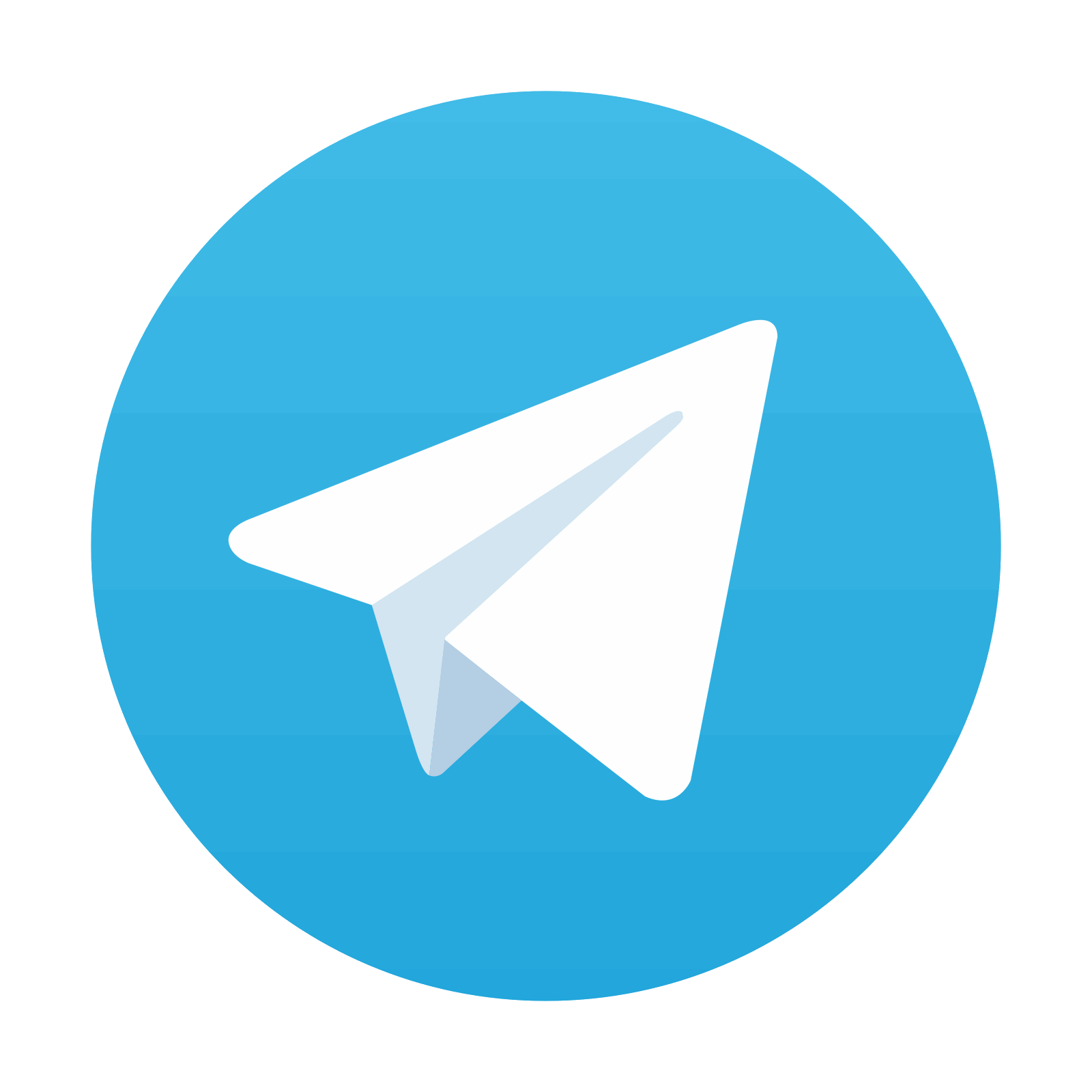
Stay updated, free articles. Join our Telegram channel

Full access? Get Clinical Tree
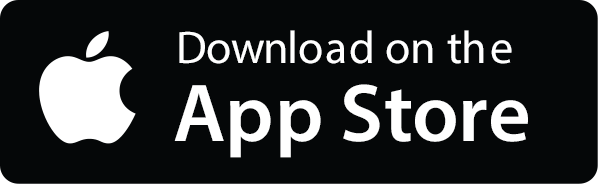
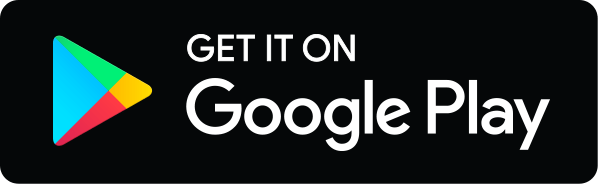