17 Fluid Management
Abstract
Edema develops at times of brain injury. The edema occurs as fluids and nutrients cross a broken blood–brain barrier or cross a cell membrane. It was once thought appropriate that the restriction of fluid would decrease the brain or spinal cord edema. However, it is now recognized that the meticulous use of the proper fluids can ameliorate edema, improve ischemia, and improve neurologic outcome. Hypotension should be avoided at all cost. Fluids are regulated in the body and brain through numerous factors, including hormones, fluid osmolality, osmolarity, tonicity, and specific cellular water receptors. Because fluids are used for medical treatment, the monitoring of the fluid volume may be required through devices other than the skin tone, mucosal dryness, urine color, blood pressure, and electrolyte concentrations in the serum and urine.
Case Presentation
A 60-year-old woman presents with a loss of consciousness, right-sided weakness, and a Glasgow Coma Scale score of 9. Her husband found her in the morning on the kitchen floor. She was wearing her nightclothes, and her husband does not know when or if she made it into the bedroom to sleep. She has a past medical history of mild chronic obstructive pulmonary disease and hypertension. She was and still is intubated because of mild respiratory difficulties. In the field, intravenous fluids (dextrose 5% in water) were started because of presumed dehydration.
See end of chapter for Case Management.
17.1 Need for Intravenous Fluids
The body is composed of 50 to 70% water and requires euvolemia to survive optimally and to overcome illness. Intravenous fluids are administered to preserve the extracellular volume and preserve electrolyte balance–allowing for adequate tissue perfusion in the critical care setting, such as severe sepsis or traumatic brain injury (TBI). 1 The understanding of sodium and water balance is key to managing the patient in the neurosurgical intensive care unit (NICU). Although water content is different among individuals, in general, men have a higher percentage of body fluid, and, in either sex, the amount decreases with age. Fifty-five to 75% of the body’s total fluid is intracellular, and 25 to 45% is extracellular. The extracellular fluid is located in intravascular plasma (25%) and interstitial spaces (75%). Therefore, in a 70 kg man, of the 48 L of total body fluid, 67% is intracellular (32 L), 25% is interstitial (12 L), and 8% is intravascular (4 L).
Fluid balance is extremely complicated, governed by antidiuretic hormone (ADH) (also known as arginine vasopressin [AVP]), aldosterone, and the natriuretic peptides: brain (BNP), atrial (ANP), and C-type (CNP). The main control of fluid balance is the tonicity made mostly by sodium, with the minor constituents chloride and bicarbonate ions of the extracellular fluid adding to the remainder. Potassium, magnesium, and phosphate constitute intracellular ions and tonicity. The hormones mentioned react to as little as a 1 to 2% change in vascular tonicity.
17.2 Hormone Control of Fluids
ADH/AVP is made in the magnocellular part of the supraoptic nuclei of the hypothalamus and conveyed to the posterior pituitary, where it is secreted. It binds to the distal renal collecting tubules to stimulate water reabsorption and to make concentrated urine in response to opioids, barbiturates, and carbamazepine, as well as high osmolality, hypovolemia, stress, hypoglycemia, and pain. ADH/AVP is a potent vasoconstrictor. In addition to affecting renal tubule cells, it affects brain cells. ADH/AVP V receptors appear to control fluid entry into the brain cell by activation of aquaporin-2, allowing fluid into the cell, which, if increased excessively, increases brain edema and infarction. 2
Aldosterone is released in response to baroreceptors sensing hemorrhage, decreased intravascular volume, or decreased blood pressure. The baroreceptors stimulate renin angiotensin, leading to aldosterone release and causing sodium resorption followed by water retention.
Natriuretic peptides not only cause renal sodium loss and fluid loss but they also reduce water and sodium in areas of brain edema by directly reducing brain cell water and brain capillary permeability. 3 , 4 ANP increases cerebral blood flow and causes significant cerebral vasodilation. It is also produced in the hypothalamus and is found in the median eminence, midbrain, choroid plexus, and spinal cord. 5 BNP fibers are found along the carotid, middle cerebral, posterior communicating, and anterior cerebral arteries (► Table 17.1). 6
Normally, a person is able to drink and will become thirsty when the osmoreceptors in the anterior hypothalamus become stimulated at approximately 295 mOsm/kg. However, when the patient is comatose from stroke, trauma, tumor, infection, or other devastating neurologic disease, the physician must prescribe appropriate fluids. The average dose of intravenous fluids (IVFs) can be approximated several ways using a general or graduated, weight-based formula (► Table 17.2). There are several physiological circumstances that require increased fluids above the normal amount (► Table 17.3).
Daily Fluid Requirementsa |
100 mL/kg for the first 10 kg in 24 hours, then |
50 mL/kg for the next 10 kg in 24 hours, then |
20 mL/kg for remainder weight in kg in 24 hours age < 60 years |
15 mL/kg for remainder weight in kg in 24 hours age > 60 years |
a For 70 kg male, age 55: (1,000 + 500 + 1,000)/24 = 104 mL/h |
Hourly Fluid Requirementsa |
4 mL/h for the first 10 kg |
2 mL/h for the next 10 kg |
1 mL/h for remainder weight in kg |
a For 70 kg male, age 55: 110 mL/h |
Daily Fluid Requirements, Averagea |
Average 35 mL/kg, elderly 30 mL/kg |
a For 70 kg male, age 55: 2,450/24 = 102 mL/h |
If these conditions are not taken into consideration, a patient will become hypovolemic. Volume status can be approximated using the following clinical criteria:
Heart rate: > 110 beats/min.
Systolic blood pressure (SBP): < 90 mm Hg.
Dry mucosal surface.
Crackling heard in lungs.
Evidence of skin edema or turgor.
Central venous pressure: < 6 to 8 mm Hg.
Urine specific gravity: < 1.010 or > 1.030.
Urine color: clear or amber.
Urine output: < 0.5 mL/kg/h.
Daily weight change: increase or decrease of 1 kg.
Input and output, 8 hours: < 500 mL (24 hours: < 1,500 mL).
Each value by itself may not be diagnostic, but when weighted, it can lead to a clearer idea of fluid balance.
17.3 Osmolality, Osmolarity, and Tonicity
Just knowing the fluid balance of the patient or even the cell will not allow proper maintenance. The amount of solutes concentrated in fluid is also vital. Osmolality is a measure of the number of osmotically active particles measured in osmoles or milliosmoles per kilogram of solvent (mmol/kg or mOsm/L). A highly concentrated osmotic solution will have a high osmolality. That is, it will have more osmotically active particles per unit of solvent than a solution with a low osmolality.
Osmolarity is the total quantity (concentration) of dissolved substances in a solution both penetrating and not penetrating the semipermeable membrane.
Tonicity reflects only the concentration of nonpenetrating solutes in the extracellular space compared with the nonpenetrating solute concentration inside the cell.
The intracellular space is a large reservoir, accounting for 67%, or 32 L, of fluid, and can easily control the solutes in the extracellular volume of 25%, or 12 L An iso-osmotic cell has an osmolarity of 300 mOsm; that is, it contains 300 mOsm of penetrating and nonpenetrating solutes. When the extracellular fluid also contains 300 mOsm of penetrating and nonpenetrating solutes, the extracellular solution is isotonic, and the cell will not change fluid volume. When the extracellular solution contains 250 mOsm (equivalent to lactated Ringer’s solution) of penetrating and nonpenetrating solutes, the extracellular fluid is hypotonic, and the cell will swell as the fluid rushes into the large cellular reservoir in an attempt to make the extracellular space isotonic. If the extracellular solution contains 921 mOsm concentration (equivalent to 3% saline) of penetrating and nonpenetrating solutes, the extracellular solution is hypertonic, and the cell will shrink; fluid will rush out in an attempt to make the extracellular space isotonic.
In reality, the cell contains different amounts of penetrating and nonpenetrating solutes compared with the interstitial and intravascular fluid. A cell may have 300 mOsm of nonpenetrating solutes and 20 mOsm of penetrating solute, such as urea, causing it to be hyperosmotic (320 mOsm). If the extracellular fluid has 300 mOsm of nonpenetrating solutes, the extracellular solution is isotonic, and the cell will not change fluid volume. The tonicity is always the relationship of the nonpenetrating solutes across the cellular membrane. Even though the cell’s osmolarity is 320 mOsm (300 mOsm nonpenetrating and 20 mOsm penetrating solutes), the extracellular solution is isotonic because it has the same concentration of nonpenetrating solutes as the cell. There are multiple osmotically active components, or osmoles, of solute concentrated in body fluid, expressed as mOsm/L. The intracellular penetrating solute concentration, such as urea and ethanol, does not affect fluid movement because the penetrating solutes will equilibrate across the semipermeable membrane. 11
The nonpenetrating particles or solutes making up the tonicity of the intravascular fluid are inorganic ions of sodium, chloride, bicarbonate, and potassium, as well as organic osmolytes of amino acids, sorbitol, and methylamines, among others. These osmoles do not easily cross the cell membrane, are restricted to the extracellular or intracellular compartments by the semipermeable cellular membrane, and therefore drive the fluid shifts or the tonicity of the fluid. Compounds such as ethanol, urea, and other solutes easily cross the intravascular boundary and therefore do not significantly add to the tonicity of the fluid, although they do add to the osmolarity.
Sodium is the major component of intravascular fluid whose regulation is closely tied to the volume of the same intravascular fluid. It is the main determinant of tonicity or effective osmolality, which determines where fluid will move. The normal plasma osmolality is 280 to 290 mOsm/L (although some sources suggest as low as 275 to as high as 295 mOsm/L) and is closely controlled by thirst osmoreceptors in the anterolateral hypothalamus. Intracellular components consist of potassium and other tightly regulated anions, leading to an osmolality of 300 mOsm/L, driving fluid into the cell. Tonicity affects fluid movement. The normal concentrations of intra- and extracellular components in osmoles are given in ► Table 17.4.
Component | Intracellular fluid (mOsm/L) | Extracellular fluid (mOsm/L) |
Sodium | 5–15 | 135–145 |
Potassium | 140–150 | 3.5–5.0 |
From the average extracellular concentrations, the daily requirements of those same constituents can be deduced (► Table 17.5).
Component | mEq/L | mEq/kg | Change in mEq |
Sodium | 60–150 | 1.0–2.0 | 80–120 |
Potassium | 55–80 | 0.5–1.3 | 50–100 |
Chloride | 60–150 | 1.0–1.7 | 80–120 |
Calcium | 0.2–0.3 | 6–10 | |
Magnesium | 20 | ||
Phosphorus | 30 | ||
Glucose | 100–200 |
17.4 The Blood–Brain Barrier and Intravenous Fluids
The blood–brain barrier (BBB) has an effective pore size of 8 angstroms (Å) and is impermeable to sodium, ions, water-soluble compounds, and protein, but is permeable to lipophilic substances and gases. The BBB is unique in that it separates the central nervous system environment from the rest of the body. This is accomplished via tight junction-connected endothelial cells and specialized transporter proteins that ensure only select molecules cross the barrier. 12 The majority of substances are actively transported across the brain capillary endothelial cell to the brain interstitial space. The cerebrospinal fluid (CSF) flows along a gradient to the ventricle and is absorbed through the arachnoid villi, where a pressure differential allows it to pass into the venous sinus. Likewise, the larger reservoir, the cellular fluid, is actively transported into the brain interstitial space and follows the same pathway. Ninety-one percent of the circulating fluid is in the intracellular and interstitial spaces; this is the area where fluid and solute need to be regulated.
The optimal treatment would be to actively remove solutes out of the cell into the brain interstitial space, through the ventricles, and into the blood. This would be accomplished with high-tonicity, low-osmolality fluid flowing from the brain capillary endothelial cell into the interstitium, increasing the tonicity of the interstitial fluid with nonpermeable sodium. Low molecular weight solutes would then leave the damaged swollen brain cell, followed by water. The damaged cell contains increased osmoles of H+ and K+. Therefore, if ion exchange mechanisms are functioning, lower interstitial solutes of H+, K+, and others would simultaneously draw the cellular toxins out of the cell into the CSF, followed by water, then drained out of the ventricle into the venous system; simulating CSF ventricular dialysis.
Inhibiting CSF formation or decreasing intravascular volume does not decrease intracellular and interstitial edema. Decreasing edema is accomplished by removing CSF.
The microvascular endothelial cells are highly susceptible to hypoxia and are prone to destabilization in certain conditions. During times of trauma and disease, the cell may be swollen in an initial response to dilute the solute toxins. Severe TBI is a condition that predisposes the BBB to such a state. 13 There is additional swelling when fluid increases in the brain interstitial space in an attempt to dilute those increased solutes that entered through the broken BBB. If the interstitial fluid does not drain out of the ventricle, the brain will swell, compressing blood vessels and leading to further ischemia.
The brain initially attempts dialysis by opening the BBB, allowing water and solutes to enter the brain interstitium, attempting to have a hypo-osmolar, hypertonic solution, so that solutes may leave the cell, followed by the increased cellular water.
The BBB, which is formed by the cerebral capillary endothelial cells, contains channels regulated by aquaporins through which water flows somewhat passively down an osmotic gradient. The cerebral capillary endothelial cells are different in function and regulation from those of the brain arteriole or venule. The other brain fluid barrier, albeit much smaller in area, is between the blood and CSF and consists of the choroid plexus epithelium. Tight gap junctions, low pinocytic activity, and specific energy-dependent membrane transporters regulate the passage of substances into and through the capillary endothelial cells to the brain interstitial fluid. Substances can freely diffuse into the capillary endothelial cells if they are gases, lipid soluble, and have a molecular weight less than 400 to 600 daltons (Da). However, most substances, such as choline, glucose, glutamate, and lactate, are associated with either a carrier-mediated transport system or solutes, such as cationized albumin, insulin, insulin-like growth factor, and transferrin. Others are associated with a receptor-mediated transport system. Both systems transport substances through the capillary endothelial cell into the brain interstitial space.
The intact BBB only mildly restricts the passive diffusion of water from a cellular hypotonic solution to an interstitial hypertonic space through aquaporin-regulated channels. In the adult, the BBB regulates most common IVF types. However, the brain damaged by trauma, ischemia, tumors, and increased pressure does not have an intact BBB, nor is the barrier intact with changes in normal brain homeostasis that occur with edema, hyperventilation, mannitol infusion, hyperthermia, hypotension, hypertension, or tumor necrosis factor secretion. During these times of stress, when the BBB is open, vasogenic edema has begun, and the brain is at further risk of damage if the incorrect therapeutic fluids are used. The damaged brain does not benefit from dehydration hypovolemia but from a euvolemic to slightly hypertonic state. 14 , 15 During times of initial brain injury systolic blood pressure spikes above 140 mm Hg must be avoided at all costs, as well as hypotonic fluid, and hyperthermia.
In addition to vasogenic edema from an opened BBB, the more common cellular cytotoxic edema forms in an effort to combat further injury, diluting the cellular toxins accumulated because of decreased blood and plasma flow (e.g., hydrogen ions and potassium). Therefore, dehydration hypovolemia becomes deleterious for combating cytotoxic edema. Euvolemia must be maintained to flush the toxins from the cell. The cell must maintain an osmotic gradient in the brain interstitial space, which allows the transport of solutes and water out of the cell.
Since 1919, the deleterious effects of hypotonic solution have been known. 16 Decreasing the IVF 1 mEq/L will decrease the osmotic pressure 38.6 mm Hg; likewise, decreasing the IVF 5 mEq/L (10 mOsm/kg) will decrease the osmotic pressure 193 mm Hg. This change in fluid drive is minimal under normal homeostasis but will aggravate the swelling and increase the intracranial pressure (ICP) in an opened BBB, a condition that occurs with tumors, ischemia, and trauma. The best fluid approximates or is slightly above normal sodium concentration and normal tonicity without having an additional glucose load. The calculated mOsm/L of individual fluids is shown in ► Table 17.6; however, it should be noted that the actual measured osmolality is ~ 20 mOsm/kg water fewer 17 Administering hypertonic saline decreases intracellular volume from 32.0 to 30.6 L, increases interstitial volume from 12.0 to 13.2 L, and increases intravascular volume from 4.0 to 4.4 L. Normal saline has no significant effect on intracellular volume, increases interstitial volume from 12.00 to 14.25 L, and increases intravascular volume from 4.00 to 4.75 L. Dextrose 5% in water (D5W) increases intracellular volume from 32 to 34 L, increases interstitial volume from 12.00 to 12.75 L, and increases intravascular volume from 4.00 to 4.25 L. 18 Therefore, normal saline should be used for resuscitation and any time there is brain damage or the potential for brain damage. D5 W, half-normal saline, and lactated Ringer’s solution should be avoided as they can exacerbate cerebral edema resulting in increased ICP. Hypertonic saline must be considered during times of increasing cerebral edema (► Table 17.6).
Prescribing additives to IVFs must be done with care. Hyperglycemia at the time of primary or secondary injury aggravates ischemic insults and worsens neurologic outcome by increasing lactic acidosis. The condition is not from previously unknown or untreated hyperglycemia; rather, it is usually from reaction to trauma, stress, glucocorticoid administration, sepsis, or other causes.
Hyperglycemia causes a dose-dependent increase in the severity of ischemic and severe head injury. In one study, glucose > 150 mg/dL had worse outcome than glucose < 150 mg/dL. 19 Hyperglycemia-induced lactic acidosis increases neurologic damage, increases infarct size, and causes secondary injury, a condition that appears to be a major cause of decreased outcome in patients with severe head injury. 20 , 21
Therefore, under conditions of suspected brain injury, as seen with ischemia, tumors, and trauma, do not give IV solutions that would worsen edema, such as those containing dextrose, low tonicity, and low sodium. 22 Start fluid maintenance with IV normal saline to keep the patient euvolemic to slightly hypervolemic.
17.5 Hypovolemia
Hypotension must be avoided at all costs. Per the Brain Trauma Foundation Guidelines, even one episode of hypotension (SBP < 90 mmHg) doubles morbidity and mortality. Hypovolemia decreases cardiac output and nervous tissue perfusion. It may be seen with a blood urea nitrogen/creatinine (BUN:Cr) ratio greater than 15/20:1 or any other number of clinical conditions. Also, it does not improve outcome after brain injury.
The body attempts to correct hypovolemia by upregulation of norepinephrine, ADH, renin-angiotensin II, and aldosterone, each increasing sodium reabsorption and water retention. Sodium absorption is increased in the renal proximal tubule cells by rennin-angiotensin II, and reabsorption of sodium occurs at the distal tubule and collecting duct by the stimulation of aldosterone. Norepinephrine decreases glomerular filtration rate–enhancing sodium reabsorption at the proximal tubule. However, these hormones also affect brain cell water absorption in a similar fashion, further increasing water retention in the brain interstitium and cell. Therefore, not only trauma patients but also patients with cerebral ischemia, vasospasms, and spinal cord injuries require fluid resuscitation. Euvolemia to slight hypervolemia improves outcomes in head trauma. Each effect of hypotension further worsens the effects of trauma. Daily maintenance fluids should be administered to compensate for the normal loss, for example, in a 70 kg man of 1,200 to 1,500 mL/d and to maintain a urine output of 0.5 to 1.0 mL/kg/h. If there are questions concerning volume status that cannot be determined using clinical and laboratory data presented in this chapter, then a FloTrac sensor (Edwards Lifesciences), a Pulse Contour Continuous Cardiac Output, PiCCO (GE Healthcare), or a Swan-Ganz catheter (Edwards Lifesciences) should be inserted to attempt to obtain normal 23 , 24 or optimal values in the management of head injury (► Table 17.7). 25
Normal values | Optimal values (mm Hg) | |
Central venous pressure | 0–8 | 6–8 |
Pulmonary artery diastolic pressure | 6–16 | 12–16 |
Pulmonary capillary wedge pressure | 6–14 | 10–14 |
Patients with spinal cord injury have decreased sympathetic tone, leading to vasodilation, bradycardia, hypotension, and decreased perfusion. These patients must have adequate fluid resuscitation with euvolemia and vasopressors to restore sympathetic tone. They should not be overhydrated, which would cause hyponatremia, pulmonary edema, and worsening of spinal cord edema.
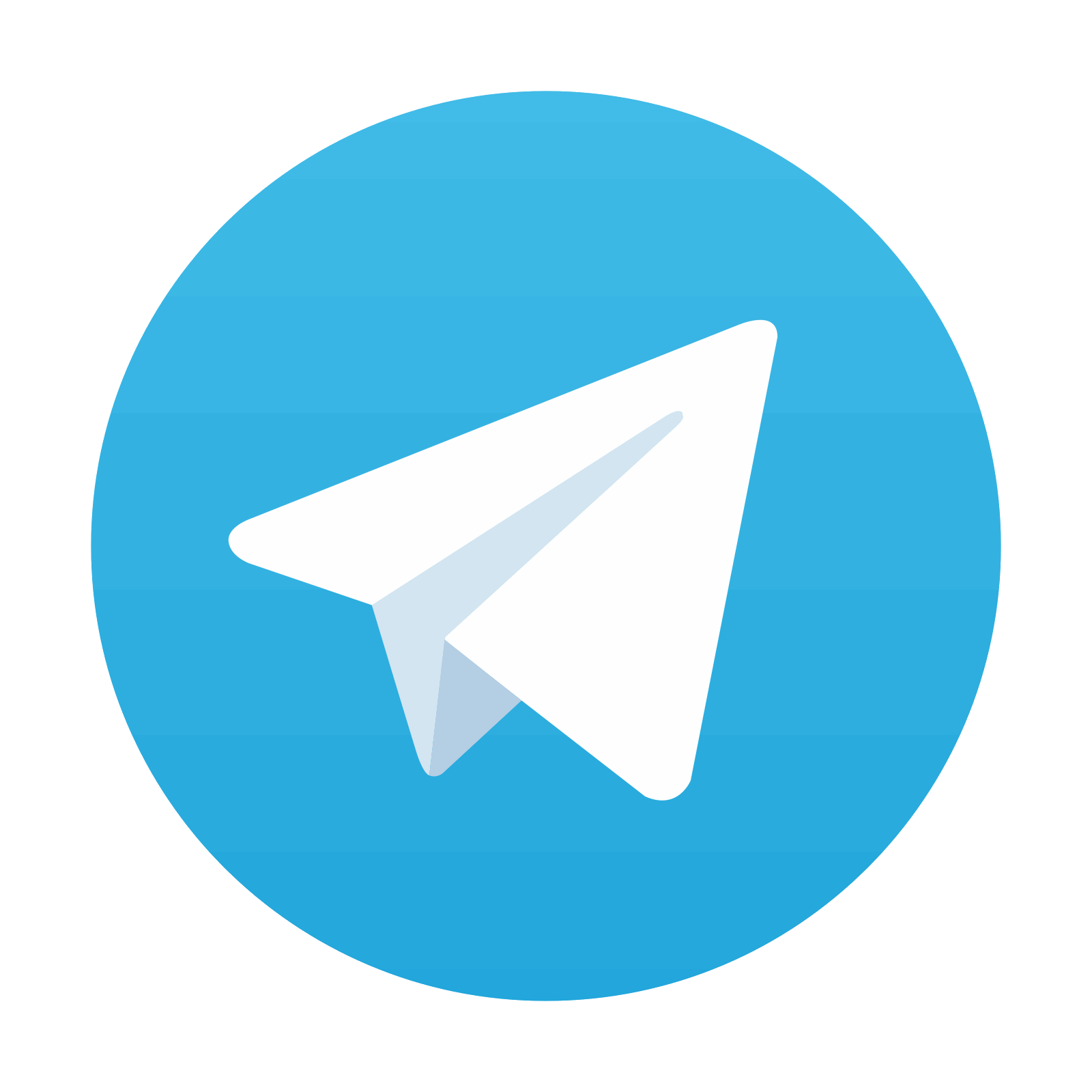
Stay updated, free articles. Join our Telegram channel

Full access? Get Clinical Tree
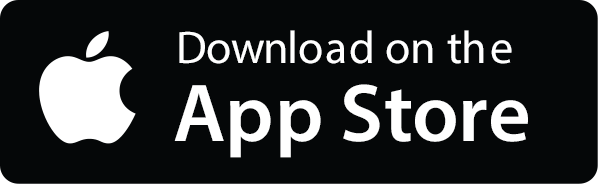
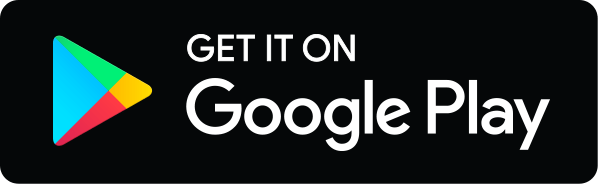