18 Ventilator Management
Abstract
A single episode of hypotension or hypoxia doubles the morbidity or mortality associated with severe brain injury. The neurointensivist has available the mechanical means to lessen the risk of hypoxia, and the ventilator should be used timely and appropriately to prevent ischemia. In the setting of central nervous system disease quick timing to restore oxygenation can prevent the neurons in the ischemic penumbra from dying and is required to prevent surrounding cells from infarction. However, it should never be thought that giving oxygen through the ventilator is the only means to restore oxygenation to the central nervous system tissue. Delivering oxygen is dependent upon a functional airway and is the first consideration. Once intubated, the meticulous control of the oxygen levels, carbon dioxide levels, and airway pressures both in the patient and in the ventilator can improve brain tissue oxygenation as long as the act of breathing and moving the oxygen across the alveoli into the circulation can be accomplished.
Case Presentation
A 43-year-old man presents after a motor vehicle accident with obvious contusions to his chest, abdomen, and head. At the scene he easily makes quick swallow breaths, he does not follow commands nor open his eyes to pain, he makes incomprehensible sounds and has flexor/decorticate posturing. His pupils are equal and briskly reactive. There is no obvious asymmetry of chest movement or chest deformity, and the abdomen is slightly distended but not hard. Should the patient be intubated?
See end of chapter for Case Management.
18.1 Reasons for Intubation
The Brain Trauma Foundation’s Guidelines for the Management of Severe Traumatic Brain Injury (4th edition) address blood pressure and oxygenation as follows:
Guideline: hypotension (systolic blood pressure < 90 mm Hg) or hypoxia (apnea or cyanosis in the field, PaO2 < 60 mm Hg, or SpO2 < 90%) must be scrupulously avoided, if possible, or corrected immediately.
The brain and spinal cord require constant adequate blood flow for oxygen and energy substrates. Ischemia must be prevented at all costs to prevent or minimize secondary injury. Depending upon the severity and onset of central nervous system tissue hypoxia, glucose and glycogen are depleted in 4 minutes. In the setting of brain injury, hypoxia exacerbates the primary injury and leads to secondary injury. This is because, in addition to the general lack of energy substrates, there is a demand for additional energy from the injured brain as demonstrated by an increased metabolism and an increased brain temperature in the layer surrounding the penumbra. When the brain tissue partial pressure of oxygen (PbO2) is less than 20 mm Hg, anaerobic respiration becomes the predominant energy exchange mechanism. This leads to glucose-store-dependent lactic acidosis, decreased pH, vasodilatation, mitochondrial damage, and cell death (aka secondary injury).
Intubation may help prevent significant secondary brain injury from hypoxia, which is oxygen saturation less than 90%, PaO2 less than 60 mm Hg, apnea, or cyanosis. Stocchetti et al 1 demonstrated that hypoxic patients without hypotension had three times the mortality rate and 20 times the severe morbidity rate of the nonhypoxic patients. Furthermore, prehospital intubation of severe trauma victims significantly reduced mortality. 2 Systematic withholding of endotracheal intubation in patients with severe head injury is not recommended.
18.2 Requirements for Intubation
The patient in our case study has a Glasgow Coma Scale (GCS) score of 6; 3 for decorticate/flexor posturing, 2 for incomprehensible sounds, and 1 for not opening his eyes. The definition of severe head injury is a GCS score of 8 or less, and according to the Guidelines for the Management of Severe Traumatic Brain Injury initial management and resuscitation should include intubation: GCS ≤ 8 intubate.
Careful and rigorous neurologic examination, including assessment of brainstem reflexes, might help to identify patients with a very high probability of death despite mechanical ventilation. 3
Considerable national variation in the care of severely head injured patients still persists even though the Guidelines for the Management of Severe Traumatic Brain Injury were first published in 1995. Those that actively follow those management strategies have an associated decreased mortality rate for patients with severe head injury, with no significant difference in functional status at discharge among survivors. 4
In addition to data supporting intubation for severe head injury the literature does support intubation for stroke as well. However, intubation in the face of stroke still remains somewhat controversial. Magi et al 5 concluded that the overall prognosis of patients with acute stroke intubated and ventilated at presentation in hospital for deterioration is severe, but the observed survival rate is sufficient to justify this treatment, even in cases not requiring other invasive procedures such as neurosurgery or angiography. The severity of the ischemic event often leads to mechanical ventilation, and in two-thirds of those individuals, death in the hospital; the remainder are severely disabled. In a study of a multiethnic urban population, survival was unlikely if patients were deeply comatose or deteriorated clinically after intubation. However, the authors concluded that mechanical ventilation for stroke was relatively cost-effective for extending life but not for preserving quality of life. 6
18.3 Methods of Intubation
18.3.1 Preintubation
Prior to intubation the patient is preoxygenated with 100% oxygen to maintain the arterial oxygen percent saturation (O2 saturation) at the highest level. Maintaining cervical spine precautions, with previous placement of a cervical collar, a jaw thrust technique keeps the airway open for oxygenation. Do not use a head tilt technique in a patient with any suspected spinal injuries. After suctioning the upper airway using spinal immobilization the patient then undergoes rapid sequence intubation with in-line orotracheal, blind nasotracheal, esophageal-tracheal (ET) Combitube (Moore Medical), or laryngeal mask airway. The equipment must be available, and tube cuffs checked prior to delivering the paralytics. If tracheal intubation cannot be performed because of facial fractures then intubation after cricothyrotomy or tracheotomy must be considered.
Preintubation:
Maintain C-spine precautions.
Preoxygenate with 100% oxygen.
Check ET-tube cuff inflation and deflation.
Suction patient.
Do not use head tilt in trauma patient.
Rapid sequence sedation and paralysis.
Intubate.
Orotracheal.
Blind nasotracheal.
Esophageal-tracheal Combitube.
Laryngeal mask.
Cricothyrotomy or tracheotomy.
18.3.2 Rapid Sequence Intubation
Medications in order of use for rapid sequence intubation are as follows: (1) Preoxygenate with 100% oxygen by mask. (2) Premedicate with lidocaine 1.5 to 2 mg/kg intravenous (IV) over 30 to 60 seconds to help prevent a spike in increased intracranial pressure (ICP), if the latter is an active issue. Maximal effect requires allowing approximately 3 minutes prior to attempted intubation. (3) Use of a sedating agent (see later discussion) if there is any semblance of alertness or awareness prior to paralyzing a patient. (4) The use of a nondepolarizing paralytic, such as rocuronium, may be considered at the 10% intubating dose of 0.06–0.1 mg/kg to lessen fasciculations from the next drug, the depolarizing agent succinylcholine, at 1.5 mg/kg IV push, which acts in 30 seconds to 1 minute. While intubating, raise the head of the bed to 30 degrees when possible as one would in general to control elevated ICP. Attempt tracheal intubation with cricoid pressure if needed. If unable to intubate within 20–30 seconds stop and ventilate with a bag-mask for 30–60 seconds before once again attempting orotracheal intubation. After the tube is visualized passing through the vocal cords confirm placement by listening to breath sounds over the lateral chest, watching the chest rise and fall, not just the abdomen, seeing a mist in the tracheal tube with expiration, and using a CO2 detector after five to six breaths or an esophageal detector device. Treat intubation-induced bradycardia with atropine 0.5 mg IV push in adults or 0.1 mg minimum in children (0.01 mg/kg).
Rapid sequence intubation: 7 , 8 , 9
Preoxygenate with 100% oxygen.
Lidocaine 1.5–2 mg/kg IV.
Rocuronium 0.06–0.1 mg/kg.
Succinylcholine 1.5 mg/kg intravenous push (IVP).
Intubate.
If unsuccessful bag-mask ventilate for 30–60 seconds.
Reattempt.
To determine whether the intubation has been successful, perform the following:
Listen for breath sounds over lateral chest.
Watch chest, not abdomen, rise and fall.
Observe mist in ET tube.
Use CO2 detector after five or six breaths.
Treat intubation-induced bradycardia with atropine.
Premedication/induction/sedation in head injury patients may prevent the increased ICP that can occur from pain or suctioning. Use drugs depending upon the time requirement for intubation and the need to assess the neurologic exam again after intubation. Agents include those listed in ► Table 18.1.
Paralytic prophylaxis prior to succinylcholine or paralytics in place of succinylcholine are shown in ► Table 18.2. Use an alternative agent if there are significant risk factors against succinylcholine. These include hyperkalemia or high risk of hyperkalemia that may result from prolonged immobilization, myopathies, renal failure, or extensive burn injury or muscle crush injury, as well as already dangerously elevated ICP, or personal or family history of malignant hyperthermia. 7 , 8 , 9 Cisatracurium may be preferred in those with renal or liver failure or very advanced age, due to organ-independent Hofmann degradation, thus avoiding prolonged effects. 10 , 11 Remember after intubation the patient will need to have a neurological exam. Sometimes the patient may need to be examined for brain death as the intubation was a reaction to a code or simply finding the patient. It is best to decide quickly prior to intubation the neurologic status. However, the GCS score should be determined after resuscitation and not in place of resuscitation.
Drug | Paralytic (mg/kg) | Prophylaxis (mg/kg) | Onset | Duration (min) |
Succinylcholine | 1–2 | – | 30–60 s | 4–6 |
Rapacuronium | 1.5 | – | 60–90 s | 15 |
Rocuronium | 0.6–1.2 | 0.06 | 2 min | 30–90 |
Vecuronium | 0.15–0.25 | 0.01 | 2–5 min | 25–40 |
Cisatracurium | 0.15–0.2 | – | 1.5–3 min | 44–81 |
Atracurium | 0.4 | 0.04 | 3–5 min | 20–35 |
Pacuronium | 0.1 | 0.01 | 3–5 min | 45–60 |
Side effects of commonly administered drugs include the following:
Succinylcholine: depolarizing effect may cause fasciculations and increase ICP or cause muscle pain—give with prophylaxis. May cause dysrhythmia and hyperkalemia. May cause cardiac arrest in healthy children and adolescents. May cause malignant hyperthermia.
Pancuronium: vagolytic; can increase cardiac output, ICP, and pulse rate. Reversible with neostigmine and other anticholinesterases. 12
Ochs et al 13 evaluated the ability of paramedic rapid sequence intubation to facilitate intubation of patients with severe head injuries in an urban out-of-hospital system. They examined adult severe head injury patients more than 10 minutes away from the hospital. The patients were premedicated with midazolam, paralyzed with succinylcholine, and given rocuronium after tube placement was confirmed. The Combitube was used as a salvage airway device. Outcome measures included intubation success rates, preintubation and postintubation oxygen saturation values, arrival arterial blood gas values, and total out-of-hospital times for patients intubated en route versus on scene. Of the 114 enrolled patients, 84% underwent successful endotracheal intubation, and 15% required Combitube intubation, with only 1 (0.9%) airway failure. There were no complications and blood gases were superb with paramedic-performed rapid sequence intubation of patients with severe head injuries.
18.3.3 Emergent Cricothyroidotomy
Emergent cricothyroidotomy should only be performed by individuals familiar with the anatomy after unsuccessful or unavailable endotracheal intubation in upper airway obstruction. The procedure requires a knife blade and handle, antiseptic solution, and a tracheostomy tube, and a pediatric ET tube or 14-gauge catheter over needle. Palpate the cricothyroid membrane between the thyroid and cricoid cartilages; it is the soft space between the “Adam’s apple” and the first hard ring toward the chest. A vertical incision is made in the skin and then the cricothyroid membrane is incised horizontally. Next the knife handle is inserted into the opening and rotated 90 degrees. The tube is passed through the opening and the cuff inflated. If a 14-gauge catheter-overneedle is available it may be used to puncture the cricothyroid membrane, removing the needle and mounted with a 12-mL syringe, which will serve as a connector for ventilation. The cricothyrotomy may be converted under controlled conditions in the operating room by a skilled person to a tracheostomy once the airway has been secured. Tracheostomies are not appropriate procedures for urgent situations. 14
Emergent cricothyroidotomy:
Palpate the first space between Adam’s apple and hard ring.
Make vertical incision in skin.
Make horizontal incision in cricothyroid membrane.
Insert knife handle and turn 90 degrees.
Pass tube through opening and inflate cuff.
18.4 Hyperventilation after Intubation
Normal ventilation is currently the goal for severe TBI patients in the absence of cerebral herniation and normal partial pressure of carbon dioxide in arterial blood (PaCO2) ranges from 35–45 mm Hg. 15
Guideline: The use of prophylactic hyperventilation PaCO2 less than or equal to 25 mm Hg is not recommended.
Brain tissue oxygenation is dependent upon cerebral blood flow, or the related measurement of cerebral perfusion pressure. Therefore, treatment modalities that cause vasoconstriction and decrease cerebral blood flow, such as aggressive hyperventilation, must be questioned and modified in the management armamentarium.
Hyperventilation works by decreasing plasma PCO2, which causes an increase in the ambient pH in or around the brain cerebrovasculature, leading to cerebral vasoconstriction decreasing the cerebral blood flow and in some cases decreasing ICP. Early after closed head injury cerebral blood flow is low. Cerebral blood flow during the first day after injury is less than half that of normal individuals; it then increases for at least 3 days thereafter, except in those patients who have uncontrollable ICP.
Cerebral blood flow is generally less than 30 mL/100 g/min during the first 8 hours after injury and may be less than 20 mL/100 g/min during the first 4 hours after injury in patients with the worst trauma. The cerebral blood flow around contusions is lower than the global blood flow. The blood flow in punctate hemorrhages may be even lower. Individuals with severe head injury may actually have increased oxygen extraction for 24–36 hours, requiring at least baseline blood flow, and possibly higher.
Cerebral blood flow has been measured with hyperventilation, and there are significant differences in and around contusions, even having local variability secondary to hyperventilation-induced steal. Cerebral blood flow does change with hyperventilation; there is a 3 to 4% change in cerebral blood flow per millimeter change in PCO2, as demonstrated by Obrist et al. 16
When cerebral blood flow is decreased, the level at which irreversible ischemia or infarction takes place is not precisely known. The same study by Obrist et al suggested that, in patients with severe head injury, there is a depression of normal cerebral metabolism, and the reduced cerebral blood flow that occurs may in many cases be appropriate for the metabolic needs of the brain. Cerebral blood flow is lowest in patients with subdural hematomas, diffuse injuries, and hypotension, and highest in those with epidural hematomas or normal computed tomographic scans. There is a direct correlation between cerebral blood flow and Glasgow Coma Scale score during the first 4 hours after injury, as demonstrated by Bouma et al, 17 and therefore the consequences of cerebral blood flow reducing hyperventilation become more apparent.
Aggressive hyperventilation to a PCO2 of 25 or less has been the cornerstone in the management of severe head injury for more than 20 years because it can cause a rapid reduction of ICP. However, there are no studies showing an improvement in outcome in neurologic patients with severe head injury using hyperventilation empirically. Hyperventilation reduces ICP by causing cerebral vasoconstriction and a subsequent reduction in cerebral blood flow. As stated earlier, cerebral blood flow is reduced by 50% during the first day after severe head injury and, therefore, hyperventilation can only exacerbate this already deadly condition. Raichle et al 18 looked at a group of healthy individuals treated with hyperventilation. When these individuals had their PCO2 decreased by 15 to 20 mm Hg from normal, there was a 40% decrease in cerebral blood flow after 30 minutes. Four hours later, despite continued hyperventilation, the cerebral blood flow increased to 90% of baseline, even though the PCO2 was still decreased, demonstrating that hyperventilation to reduce ICP may only be a temporary phenomenon. This suggests that hyperventilation, if necessary, should only be used in short bursts.
In the same study when the original PCO2 was quickly restored the cerebral blood flow was increased to 31% above baseline. The quick restoration increases cerebral blood volume and in turn can increase ICP. Therefore, when elevating PCO2 from below normal levels, gradually increase the PCO2.
In the autoregulatory intact individual, there is a 3–4% change in cerebral blood flow per mm Hg change in PCO2. This decrease in cerebral flow occurs less when cerebral blood flow is already reduced, and this lower CO2 vasoresponsivity is associated with a poorer outcome. Local CO2 responsivity can differ from values by more than 50%. Cold et al 19 demonstrated that in some patients cerebral autoregulation is preserved with normocapnia and lost with hypocapnia. Others have found varying responses with hyperventilation. Crockard et al 20 found an increase in ICP associated with a decrease in PCO2, and Obrist found a decrease in ICP in only half of the patients treated with hyperventilation; however, over 90% of those patients also had a decrease in cerebral blood flow.
In a landmark class I study Muizelaar et al 21 published the results of a prospective randomized clinical study in which 77 patients were randomized to a group treated with chronic prophylactic hyperventilation for 5 days after injury (PCO2 of 25, ± 2 mm Hg) or to a group that was kept normocapnic (PCO2 35 ± 2). Those patients who were treated with prophylactic hyperventilation had a significantly worse outcome than those in the normocapnic group at 3 and 6 months. They did say that hyperventilation may be beneficial with hyperemia, and hyperventilation is needed in signs of acute herniation. Multiple studies demonstrate that aggressive hyperventilation causes secondary injury of decreased brain tissue oxygen. 22 , 23 , 24 , 25
The literature is replete with documentation of improved survival for severe head injury using modern treatment paradigms. The majority of physicians who are exposed to head trauma patients, explicitly the emergency department doctors, are managing severe head injury patients in accordance with the Guidelines for the Management of Severe Traumatic Brain Injury, with the exception of avoiding prophylactic hyperventilation. However, it is hoped that more education and exposure to the evidence regarding prophylactic hyperventilation of severely head injured patients may improve adherence to the hyperventilation guidelines as well. 26
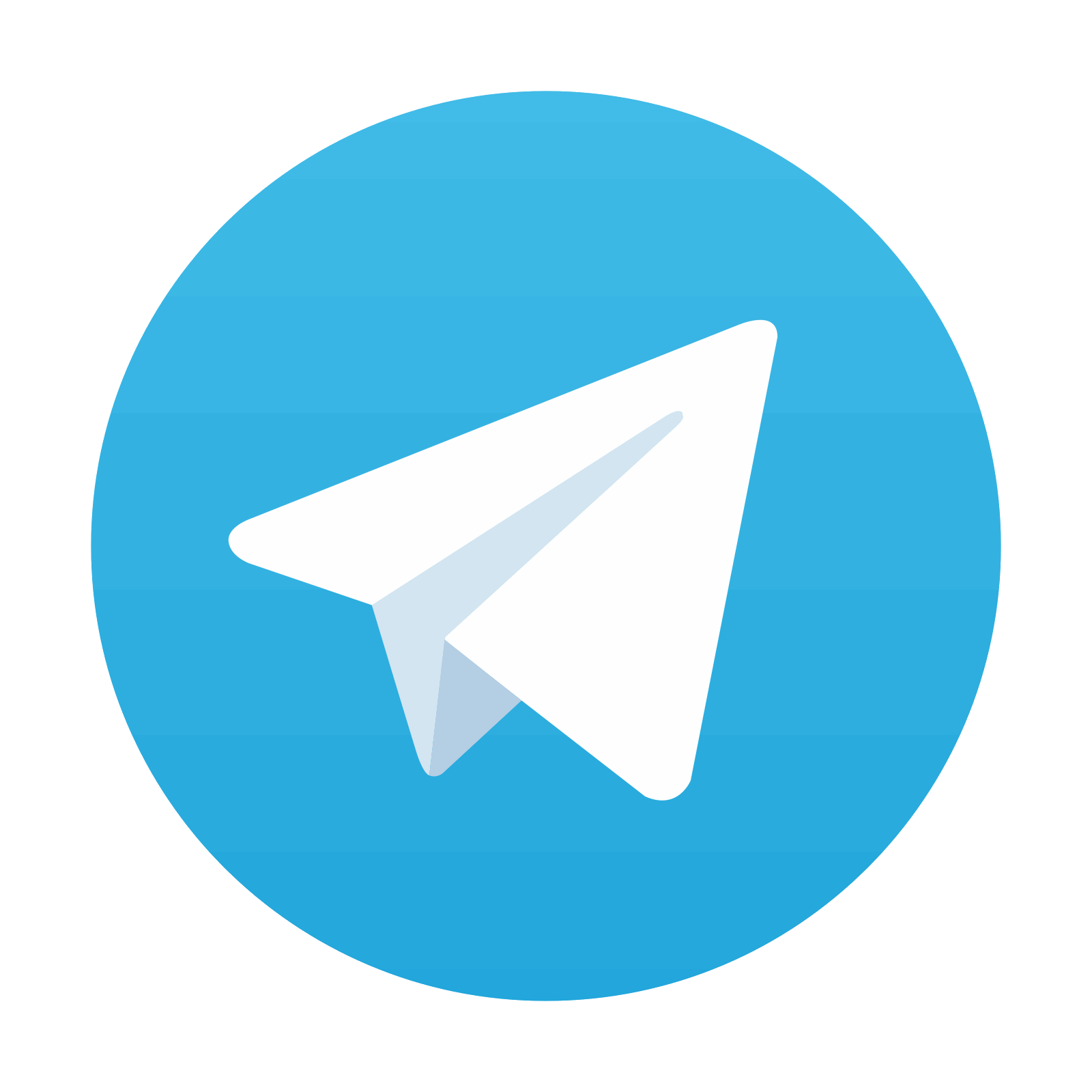
Stay updated, free articles. Join our Telegram channel

Full access? Get Clinical Tree
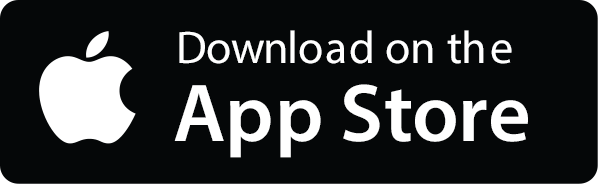
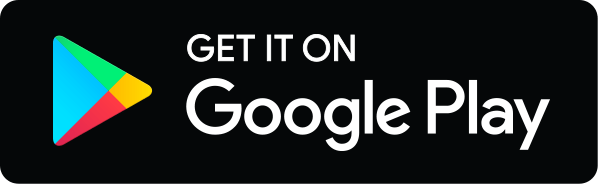