2 Age-Related Changes in the Spine
Abstract
The progressive degenerative changes associated with aging gradually affect all components of the spine and associated structures. The degenerative process begins early, during the first decade of life in the intervertebral disc. This process involves biochemical changes that are followed by macroscopic alterations, including tears and fissures. Ultimately, this may lead to loss of disc height and disc herniation. Facet joint hypertrophy and laxity often follow. Other accompanying changes include osteoporosis of the vertebral bodies, hypertrophy of the ligamentum flavum, and weakening of the paraspinal musculature. The accumulation of these age-related modifications causes narrowing of the spinal canal and collapse of the vertebral body to varying degrees. In addition, the dynamic adaptation of the spine to the forces involved in daily activities also contributes to the alterations. The excessive compensation can sometimes lead to pathological conditions, such as thoracic hyperkyphosis, which contributes to global sagittal imbalance and deformity. Furthermore, the flexibility and range of motion in both cervical and lumbar spines diminish with age. Degenerative changes in the spine are features of the normal aging process. This chapter provides the reader with an understanding of these age-related changes to provide an understanding of the natural history of spine degeneration in order to guide diagnosis of pathological conditions and determine treatment strategies.
Key Points
Progressive degenerative changes associated with aging gradually affect all components of the functional spinal unit and adjacent structures
The intervertebral discs lose water content, facet joints undergo arthritic changes, spinal ligaments hypertrophy, and paraspinal muscles weaken – eventually leading to narrowing of the spinal canal
Excessive thoracic kyphosis may become pathologic (hyperkyphosis), causing sagittal imbalance and deformity
Flexibility and range of motion in both cervical and lumbar spines diminish with age
Understanding age-related changes in the spine may guide diagnosis of pathological conditions and treatment strategies
2.1 Background
As the spine naturally ages and endures physical stressors associated with the activities of daily living, a gradual decrease in strength and range of motion ensues. At the cellular level, the musculoskeletal system undergoes senescence, apoptosis, alterations in the extracellular matrix due to accumulation of posttranslational modifications of matrix proteins as well as an increase in oxidative stress due to the production of reactive oxygen species. Systemically, the body experiences reduced levels of trophic hormones, decreased function of organs, and generalized impairment of mobility. These multifactorial changes may affect any part of the musculoskeletal system, particularly the spine. Among the age-related pathological processes of the spine, degenerating spondylosis, or spinal osteoarthritis, is the most common. This condition is radiographically associated with narrowed disc spaces and osteophytes, or bone spurs, at the margins of the vertebral body. Autopsy studies have demonstrated spondylosis in 60% of women and 80% of men by age 49, and in 95% of both genders by age 70. 1
2.2 The Functional Spinal unit
The bony spine is comprised of multiple segmental levels and columns at each level, providing structural stability as well as functional mobility, while protecting the nerve roots and the spinal cord. The functional spinal unit refers to the smallest anatomical unit that includes all the basic functional characteristics of the entire spine, first described by Schmorl and Junghanns. 1 Each segmental level consists of two adjacent vertebrae separated dorsally by facet joints and ventrally by the intervertebral disc. These components collectively constitute the functional spinal unit (Fig. 2‑1). The vertebrae are also supported by ligaments, joint capsules, and paraspinal musculature. The spinal ligaments include the interspinous and supraspinous ligaments, the ligamentum flavum, and the anterior and posterior longitudinal ligaments. The muscles spanning over two vertebrae include the splenius, erector spinae, transversospinal, and segmental muscles. The stability and mobility of the spine are primarily achieved via the interplay between the aforementioned structures and the powerful flexor and extensor muscle groups. Normal spinal function also relies on the proper interaction with the three-joint complex, which is comprised of the intervertebral disc and the two facet joints (functional spinal unit). Any significant alteration of these components may cause dysfunction, eventually leading to pain, deformity, and neurologic compromise.

2.3 The Intervertebral Disc
Intervertebral discs are located between the vertebral bodies, transmitting the load from body weight and muscle activity and simultaneously facilitating motion. Such motion includes bending, twisting, and cushioning (axial load-related deformation). The intervertebral disc (Fig. 2‑2) consists of a gelatinous core (nucleus pulposus) and a fibroblast-like cell matrix with parallel collagen fibers arranged in concentric rings called lamellae (annulus fibrosus). 2 , 3 Elastin fibers, also within the lamellae, contribute to restoration of the disc morphology after movement of the spine. 4 The nucleus pulposus dissipates the compressive forces on the disc by exerting hydrostatic pressure on the annulus fibrosus. The nucleus pulposus contains collagen fibers, elastin fibers, and aggrecan-containing proteoglycan gel with interspersed chondrocyte-like cells. 5 Collagen comprises up to 70% of the annulus fibrosus but only 20% of the nucleus pulposus. 6 On the other hand, up to 50% of the nucleus pulposus contains proteoglycans, whereas the annulus fibrosus only contains 20%. Finally, the contents of the disc matrix vary due to continuous turnover by enzymes such as matrix metalloproteases and aggrecanases produced by the disc cells. 7 , 8

Degenerative changes can occur as early as 10 years of age, with microscopic tears in the nucleus pulposus, focal disc cell proliferation, and granular matrix transformation, along with an increase in acidic mucopolysaccharides in the disc matrix. 9 These changes progress through young adulthood, and by the third decade of life, the nucleus pulposus begins to lose its gelatinous consistency and develops multiple large clefts with significant granular changes in the matrix. At the molecular level, there is also a loss of proteoglycans and water content, with an increase in collagen. An adult disc, aged 30 to 50 years, has less clear distinction between the nucleus pulposus and the annulus fibrosus. By the fifth decade, the nucleus pulposus is dry, with a solid, rubbery consistency, merging with the inner annulus and containing even more collagen and fewer cells. In individuals older than 70 years, the intervertebral disc becomes more scar-like, with large tissue defects. The progressive alterations in the matrix composition often results in a loss of disc height, which subsequently increases the load on the facet joints and predisposes the bony segment to arthritic changes and the ligamentum flavum to hypertrophy, eventually leading to narrowing of the spinal canal.
Aging and degeneration are also associated with dramatic changes in vascularization and innervation of the disc. A healthy adult disc is avascular. On the other hand, in the setting of degeneration and herniation, blood vessels penetrate through the disc space in response to angiogenesis factors, metalloproteases, and various cytokines. 10 , 11 , 12 In addition, nociceptive nerve fibers expressing substance P have been found in the nucleus pulposus of discs associated with chronic pain. 13
2.4 The Cartilage Endplate
The thin hyaline cartilage endplate separates the disc from the bony interface of the adjacent vertebral body, providing biomechanical integrity and disc nutrition. At birth, the cartilage endplate is approximately 50% of the intervertebral space, in comparison to approximately 5% in adults. 9 Over time, vascular channels are gradually replaced by the extracellular matrix. The endplate acts as a growth plate for the adjacent vertebral body, similar to the epiphyseal growth plate of long bones. In adulthood, the endplate is approximately 0.6 mm thick with calcification, occupying the central 90% of the interface between the disc and the vertebral body. 9 The endplate forms a physical barrier to prevent bulging of the nucleus pulposus into the spongiosa of the vertebral body, acts as a filter between the disc and vertebral body, and partially absorbs the hydrostatic pressure dissipated by the nucleus pulposus under loading conditions (Fig. 2‑2). 14 The endplate is also the main pathway for nutrients to reach the avascular nucleus pulposus by diffusion from the blood supply of the vertebral body. 15
By the third decade of life, the endplate has sustained various alterations, including fissure and cleft formation, microfractures, and decreased chondrocytes, as well as extensive ossification. The diminished vasculature results in reduced nutritional supply to the intervertebral disc and dense sclerosis of the adjacent vertebral bodies.
2.5 The Facet Joints
The bony articulations between the posterior elements of adjacent vertebrae are called facet, or zygapophyseal, joints. These synovial joints of the spine contain hyaline cartilage overlying subchondral bone. They prevent excessive mobility of the spine and distribute axial load over a broad area, resisting most of the intervertebral shear forces. 16 Furthermore, the posterolateral fibrous capsule, comprised of multilayered fibrous tissue and synovial membrane, is richly innervated with small C-type pain fibers. 17
Similar to large synovial joints, suboptimal orientation and malalignment of the facet joints increase the risk of arthritic changes, including complete loss of articular cartilage, bony cysts and pseudocysts, dense bone sclerosis, and large osteophyte formation (Fig. 2‑3). In late stages, endplate fractures can occur that resemble breaches in the subchondral bone plate, with protruding portions of the articular cartilage into the subarticular bone. These progressive degenerative changes, often with concomitant loss of intervertebral disc height and segmental instability, lead to increased load and subsequent subluxation of the facet joints, then ultimately multilevel spondylosis and stenosis.

2.6 The Vertebral Bodies
The vertebral bodies provide most of the static stability of the spine, and age-related degeneration decreases the structural strength. Over time, the bone undergoes modifications in cytoarchitecture, density, remodeling, and repair. The increasingly fragile bone is then predisposed to osteoporotic fractures, which may ultimately cause disc herniation into the vertebral bodies and spinal deformities.
2.7 The Spinal Ligaments
The ligamentous complex surrounding the spine provides structural support and limits excessive motion in all planes. Copious amounts of well-organized collagen fibers provide tensile strength in all ligaments except the ligamentum flavum, which contains a high percentage of elastin. 18
The spinal ligaments undergo various age-related changes, including reduced concentration of collagen and water content, decrease in reducible collagen cross-links, and disorganization of collagen fibers. 19 There is also an increase in elastin with age, which decreases the tensile strength and affects the stabilizing function of the longitudinal ligaments. Hypertrophy of the ligamentum flavum, along with loss of intervertebral disc height, contributes to the gradual narrowing of the spinal canal (Fig. 2‑4). 20 , 21

2.8 The Spinal Muscles
The trunk and pelvic muscles are crucial for maintenance of posture and active movement. During motion, equilibrium and control of stability are maintained by the antagonistic actions of the extensor dorsal musculature and abdominal flexors. The presence of muscles reduces the load and stress on the vertebral body, intradiscal space, and other mechanical components of the spine.
Aging leads to loss of muscle mass, fatty infiltration, and deposits of connective tissue. Starting as early as age 25, the muscle mass decreases 3 to 8% per decade until age 50, when the degeneration rate increases to 10% per decade. 22 During age-related sarcopenia, the reduced number and size of myocytes results in a significant decrease in the strength of the muscle fibers. In women, sarcopenia is affected by levels of estrogen, vitamin D, and IL-6; whereas, in men, testosterone, physical performance, and TNF-α are the most significant contributing factors. 23 The loss of muscle mass compromises spinal stability, and the resulting imbalance may contribute to progressive structural disorganization as well as development of degenerative scoliosis.
2.9 Changes in Spinal Balance
The degenerative changes of the spine accumulate and contribute to the development of progressive kyphosis with aging. In childhood and through the third decade of life, the angle of thoracic kyphosis averages 20 to 29°. 24 After 40 years of age, the mean thoracic kyphosis increases to 53°in individuals aged 60 to 74 and to 66°in those older than 75 years of age. 25 The kyphosis angle increases more rapidly with age in women relative to men. 26
The age-related thoracic kyphosis may be difficult to distinguish from a pathological condition described as hyperkyphosis – a thoracic kyphosis angle greater than 40°, which represents the 95th percentile of normal for young adults. 24 In older adults, the incidence of hyperkyphosis ranges from 20 to 40% among both men and women. 27 The multifactorial contributing factors include chronic vertebral compression fractures, osteoporosis, and degenerative disc disease, as well as functional changes in muscle strength and posture. 28
The overall spinal alignment depends on the regional alignment of individual spinal segments as well as the pelvis. 29 The combinatorial effects of various degenerative changes, including thoracic hyperkyphosis and loss of lumbar lordosis, contribute to a global sagittal imbalance. 30 The disequilibrium of forces along the spinal column may eventually lead to deformity as well as adaptive changes in the pelvis, lower limbs, and overall spinal posture. 31 , 32 , 33
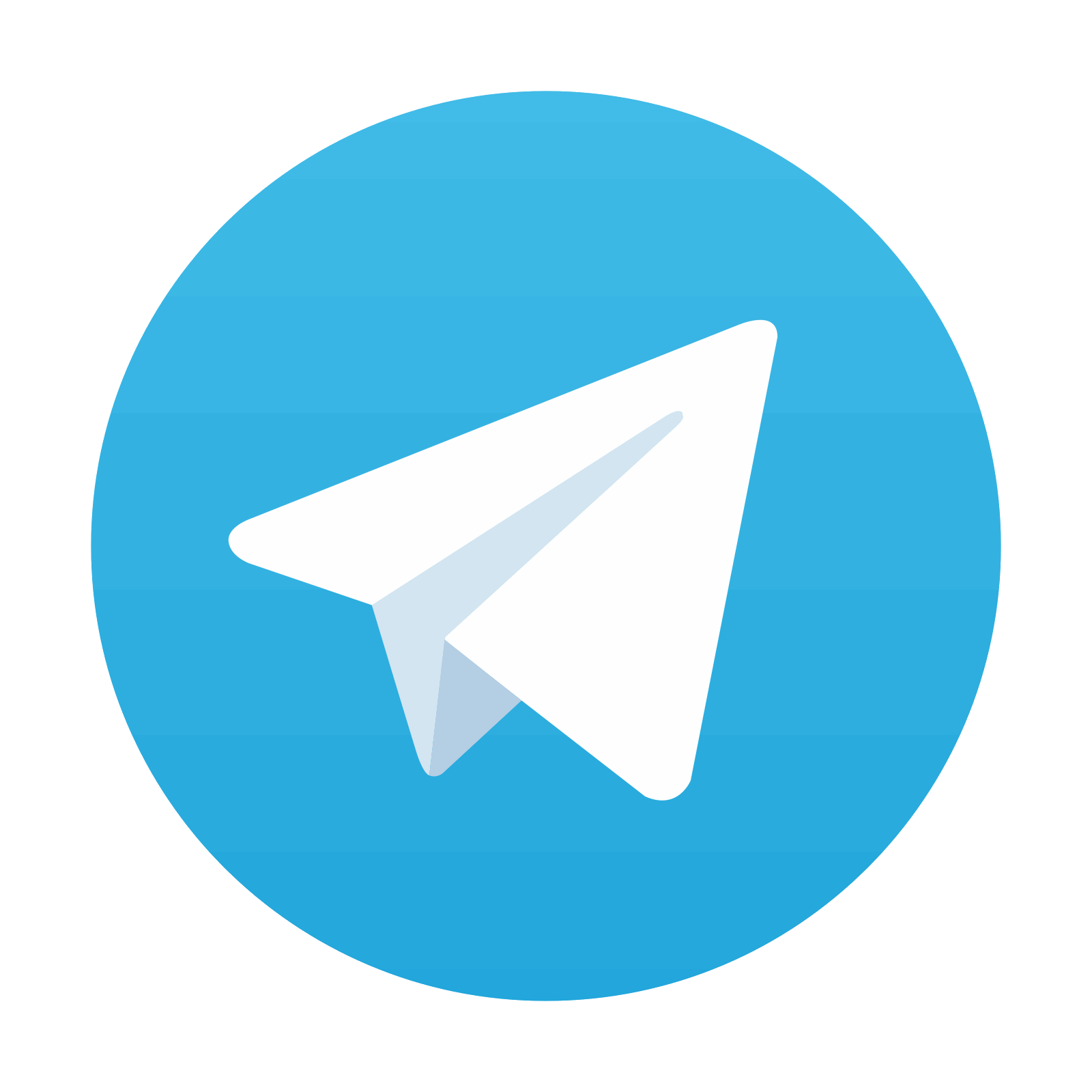
Stay updated, free articles. Join our Telegram channel

Full access? Get Clinical Tree
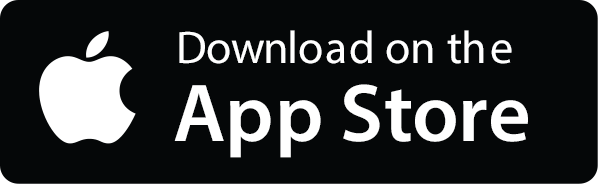
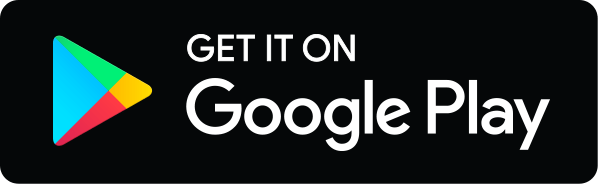