3 Diagnosis and Treatment of Osteoporosis in the Aging Spine Patient
Abstract
Low bone mass (osteopenia and osteoporosis) is a common condition that is poorly managed in our current health care system. Poor bone health can increase fragility and fracture risk and also adversely affect outcomes following spinal surgery. The spine practitioner can help patients by taking ownership of bone health. Secondary fracture prevention is aimed at making the first fracture the last fracture by diagnosing and treating poor bone health and intervening to reduce fall risk. Unfortunately, less than 20% of patients who have an osteoporosis-related fracture receive treatment for osteoporosis. Fracture liaison services are becoming increasingly available and provide a comprehensive approach to reduce the risk of subsequent fracture. These programs reduce secondary fractures by 40%. Preoperative bone health optimization is a newer approach aimed at optimizing bone health prior to surgery. Recent investigations show a link between poor surgical outcomes with vitamin D deficiency and osteoporosis. Conversely, as might be expected, treatment with bisphosphonates or bone anabolic medications improves surgical outcomes. The critical components of these programs are assessing the risk of poor bone health, identifying and correcting vitamin D deficiency, eliminating toxins that adversely affect bone (e.g., smoking and excess alcohol intake) recommending fall prevention as required, practicing weight bearing exercises, and maintaining proper nutrition. Dual-energy X-ray absorptiometry (DXA) is an essential part of evaluation, as it classifies bone status and can be ordered and interpreted by all spine practitioners. Advances in DXA testing include vertebral fracture assessment and trabecular bone scoring that may aid in identification of patients with occult fracture and patients with poor quality. Since bone is fundamental to the function of the spine, practitioners should include diagnostic and treatment of bone health when evaluating patients with spinal diseases.
Key Points
Low bone mass is endemic but poorly managed in our current health care system and negatively affects quality of life and independence.
Even after fragility fracture, only 20% of patients receive osteoporosis care
Assessment of bone health can be done by all spine practitioners.
Secondary fracture prevention programs are effective and reduce secondary fracture risk by ~40%.
Low bone mass and associated metabolic abnormalities are associated with poorer outcomes after elective spine surgery
Principles of quality bone health assessment and management include:
All patients with fractures after age 50 need evaluation, including basic laboratory and bone mineral density measurement
This evaluation includes:
Laboratory measurement of calcium, creatinine, alkaline phosphatase, 25-OH vitamin D, CBC
Personal review of the DXA scan image
Consideration of falls, home safety, vision and, if weakness or balance issues are present, physical therapy evaluation.
Calcium intake of 1000 to 1200 mg daily, vitamin D of at least 1,000 IU; consider protein supplementation in older adults.
Treat with pharmaceuticals for 3 to 5 years if fracture risk is high, such as for recent fractures, falls, FRAX risk ≥ 20% for major fracture.
3.1 Background
Low bone mass, (osteopenia and osteoporosis), is an underappreciated health crisis that increases risk for fracture in older adults, with resultant decreased quality of life, morbidity, including loss of independence, and increased mortality. The spine is the most common site of symptoms from osteoporosis due to fracture and deformity. Further, many patients with low bone mass are frequently seeking care for related or unrelated spinal diseases. Spine surgeons need to be aware that poor bone status may adversely influence surgical outcomes and increase the likelihood of complications and revision surgeries (Fig. 3‑1). The purpose of this chapter is to review the epidemiology of osteoporosis. Focus will be on the morbidity and mortality associated with spinal fractures, as well as the influence of osteoporosis on spine surgery outcomes. Additionally, current guideline-based diagnoses and overall management approaches will be reviewed. There will be an emphasis on secondary fracture care and on preoperative optimization of spinal patients for surgery.

The occurrence of a low-energy fracture in an older patient is a sentinel event that is a teaching and management opportunity. The goal is to make this fracture the last one. This is done through a systematic approach, including education, assessment, correction of metabolic abnormalities, treatment, rehabilitation, and follow-up. In 2004, the Surgeon General of the United States reported that there is “the gap between what we know and its application,” “much of what we know is not always applied in practice” and “primary care and orthopedic surgeons rarely discuss osteoporosis in patients having fractures.” 1 Unfortunately, fewer patients are being treated following fractures now than was the case in 2004. 2 Indeed, leaders in the medical osteoporosis community have called this failure to treat a “crisis.” 3 This has spurred efforts to improve delivery of secondary fracture care with the goal to prevent further fractures.
3.2 Epidemiology
Low bone density (osteopenia and osteoporosis) is common and is present in an estimated 53 million Americans in 2010. 4 This is expected to increase to 65 million in 2020 and over 71 million in 2030. Twenty percent of these patients have osteoporosis with dual-energy X-ray absorptiometry (DXA) T-scores below -2.5. Fractures occur in 2.1 million older adults annually, a number greater than that of stroke, heart attack and breast cancer combined. 5 It is estimated that these fractures cost greater than $20 billion per year; an amount expected to significantly increase. In 2011, over 325,000 patients were hospitalized for hip fracture, while 260,000 were hospitalized for spine fracture. 6 However, only one in three spine fractures are clinically appreciated, and many do not require hospitalization. As the population ages, hospitalization for fractures is expected to increase. Approximately 1% of patients in their 50s are admitted to the hospital because of fragility fractures, while of those over 80 years, approximately 5.5% receive a diagnosis of fragility fracture. 5 This is greater for females than for males. Burge estimated that between the years of 2005 and 2025, there will be a 76% increase in the number of women with fragility fracture and a 24% increase in men. 5 Costs will correspondingly increase by 73% in females and 27% in males. Schousboe has shown that clinical vertebral fractures increase significantly after the age of 65 and are more frequent in the United States than Northern Europe. 7 For example, the vertebral fracture incidence triples per 100,000 person-years between the ages of 70 and 80 for women in the U.S.
3.3 Morbidity and Mortality of Fractures in Older Adults
Fractures in older adults are life-changing events. The adverse effects of fracture on quality of life and independence have not received adequate attention by the medical community and may be unappreciated by patients. There is a significantly increased chance of loss of independence, higher mortality, chronic pain, loss of function, and reduced health-related quality of life. Tajeu compared 43,000 patients with hip fractures against age-matched comparators at one year follow-up. 8 Mortality was 28.6% at one year compared to 12% for the control group, with a risk ratio of 2.2. Debility, defined as transfer from community living to long-term care, occurred in 20% of those with hip fracture and 5.5% of controls. Destitution, defined as transfer to Medicaid or Medicare supplement, was twice as likely after hip fracture than among controls, occurring in 6.6% compared to 3.1%.
Near-term mortality after vertebral fractures is similar to that of hip fracture. Lau used the Medicare database of 97,300 patients with vertebral fracture and compared mortality to control group. 9 The Hazard ratio ranged 2.2 to 3 compared to no-fracture controls, depending on age at the time of the original fracture. Kaplan Meier analysis shows significant increased mortality over time after vertebral fracture. For instance, at 5 years, only 30% of patients with osteoporotic fracture had survived, compared to 70% of controls. Chen noted that a patient’s independence was significantly altered after vertebral fracture. At 2 years, 24% of patients were living at home, with another 15% requiring home health care services. 10 Forty-one percent were transferred to skilled nursing facilities, and 19% to other long-term care facilities.
Although only one-third of vertebral fractures are clinically evident, they may be associated with significant pain. Chen reported that average baseline pain was 7.8, improving significantly to 3.4 over 6 months in conservatively treated patients. 10 This high pain level is notable in that it is significantly greater than those patients enrolled in the SPORT trial for spinal stenosis. Tosteson, using the SF-36, reported long-term, health-related quality of life at 5 years for 215 patients having hip and spine fracture compared to 200 controls. 11 Self-reported limitations and activities of daily living (ADLs) were commonly reported in 25% of those with spine and 58% with hip fractures. If patients had combined hip and spine fractures, over two-thirds reported self-limited ADL limitations. The SF-36 physical components score was a mean of 40 in spine fracture patients, 34 in hip fractures, and 30 if the patient had combined hip and spine fractures, compared to normal age-matched control of 50. Interestingly, the mental health components scores did not vary despite the fractures. Svensson reported patients’ perceptions after vertebral compression fractures using a structured narrative technique of 10 community ambulators over the age of 80. 12 Fear concerns were dominant; they particularly struggled to understand a deceiving body and breakthrough pain and reported feelings of fear: of a trajectory into isolation, of dependency, and of an uncertain future.
3.4 Risk of Secondary Fracture
It is well known that “fracture begets fracture.” For example, Hodsman calculated the risk of secondary fractures out to 10 years in patients over 65. 13 At 2 years, 11% of hip fracture patients and 12% of spine fracture patients had secondary fractures. This increased to 15 and 16% at 5 years and 25% at 10 years. Center reviewed 2,245 patients at 16-year follow-up. 14 The risk per 1,000 patient- years in females was 80 and was even higher in males, at 101. The relative risk compared to those patients without a fracture was 2.5 in females and 6.2 in males. Kanis performed a meta-analysis of drug trials including 60,121 patients, 26% of whom had prior fractures. 15 The risk of a future fracture was doubled in patients who already had a fracture and was greater for males and younger-aged patients. Anderson performed a meta-analysis of nine randomized controlled trials of vertebroplasty compared to nonoperative treatment. 16 Secondary fractures occurred within 12 months in 19% of nonoperatively treated patients, and there was no difference in those having had vertebroplasty. Lindsay noted that with each new fracture, there was a significant and increasing risk of incident fractures. 17 Patients with one fracture had a 12% risk of secondary fractures, while those with 2 or more fractures had a 25% risk. Thus, the presence of a clinical or subclinical fracture in an older patient or from a low-energy mechanism should prompt investigation and consideration of treatment for related osteoporosis.
3.5 Diagnosis of Osteoporosis
3.5.1 Dual-energy X-ray Absorptiometry (DXA)
Dual-energy X-ray absorptiometry (DXA) is the gold standard to determine in vivo bone mineral density (BMD). The technique utilizes high and low energy X-rays which have different, nonlinear X-ray attenuation. As a result, the density which these two X-ray energies pass through tissues can be determined. DXA calculates the areal BMD (gm/cm2) of a region of interest. Typical regions of interest are the proximal femur, lumbar vertebrae, and distal radius, all three of which are common fracture sites. The bone mineral density (BMD) is then compared to a reference standard, specifically the BMD of 20- to 30-year-old females. The T-score is equal to the BMD of the patient minus the BMD of the reference standard divided by the standard deviation of the reference standard.
The Z-score is calculated using age- and gender-matched controls as a reference standard. A Z-score is used for males and premenopausal females.
One strength of DXA is that larger and therefore stronger bones will have a higher areal BMD and, as such, DXA is a good tool to predict future fracture risk. However, there are many limitations of DXA. Arthritic or degenerative changes, deformity, and the presence of surgical implants prevent accurate measurement of BMD. DXA is only measuring planar bone mineral density and will include cortical trabecular bone. In the spine, trabecular bone is far more significant in preventing fractures than the cortices. Further, variation occurs between scanners and technicians, with different resulting outcomes. Finally, recent investigations suggest that over 25% of scans have incorrect reporting. 18
3.5.2 Classification of Osteoporosis
The World Health Organization (WHO) classified bone status based on DXA T-score (Table 3‑1 ). 19 However, the WHO classification poorly predicts fracture, with more than 50% occurring in people with low bone mass or normal T-scores. Further, this system provides limited guidance for treatment. The National Osteoporosis Foundation and the National Bone Health Alliance (NBHA) recognized that bone mineral density as defined by the WHO criteria does not explain risk of fracture in half the cases. 20 As such, they recently proposed that the definition of osteoporosis be expanded to include any one of the following: a T-score of less than -.2.5; a fragility spine or hip fracture; low bone mass (-1.0 to -2.5) plus fragility fracture; and low bone mass and high Fracture Risk Assessment Tool (FRAX) probability (Table 3‑2).
Classification | T-Score* |
Normal | Greater than -1.0 |
Osteopenia | Between -1.0 and -2.5 |
Osteoporosis | Less than -2.5 |
* T-score measured at femoral neck |
T-score < -2.5 of hip, lumbar spine, or distal third of radius |
Low energy spine or hip fracture (independent of bone mineral density) |
Low bone mass (T-score -1.0 to -2.5) with fragility fracture of proximal humerus, wrist, pelvis |
Low bone mass (T-score -1.0 to -2.5) and high FRAX* risk probability |
* Fracture Risk Assessment Tool |
The Fracture Risk Assessment Tool (FRAX) is a prediction tool that calculates the 10-year hip and 10-year major osteoporotic (spine, hip, wrist and humerus) fracture risk. 21 , 22 FRAX is standardized for many countries and is based on 12 criteria, including demographics, height and weight, a history of prior fractures, parents with fractures, cigarette use, use of glucocorticoids, having rheumatoid arthritis or secondary osteoporosis, consuming more than 3 alcoholic beverages per day, and femoral neck bone mineral density based on either gm/cm2 or T score (Table 3‑3). The FRAX risk can also be calculated without the use of DXA. The FRAX tool can be accessed at https://www.sheffield.ac.uk/FRAX/index.aspx.
Criteria | Results: 10-year major fracture risk (%) 10-year hip fracture risk (%) | |
Age | Current smoker | |
Gender | Glucocorticoid use | |
Height | Rheumatoid arthritis | |
Weight | Secondary osteoporosis | |
Prior fracture | Alcohol ≥ 3 units per day | |
Parent with hip fracture | Femoral neck BMD (gm/cc) May use T-score |
Many guidelines recommend use of FRAX estimated fracture risk to determine the optimum medical treatment, including the therapeutic intervention threshold based on fracture risk. The most commonly used high-risk FRAX thresholds are a 10-year major fracture risk of 20% and a 10-year hip fracture risk of 3%, indicating patients who should be considered for pharmaceutical treatment.
3.5.3 Indications for DXA
The indications for DXA testing are: all women aged 65+ and men aged 70+; women aged 50 to 64 with a FRAX score greater than 9.3 percent; major osteoporosis-related fracture risk per the United States Preventative Service Task Force (USPSTF); men between the ages of 50 to 69 who exhibit one or more of the following: a prior fracture, glucocorticoid use, or rheumatoid arthritis; and, finally, anyone with a recent fracture (Table 3‑4). 23
Females > 65 |
Males > 70 |
Females 50–64 and FRAX >9.3 for major fractures |
Males 50–64 with history of prior fracture, glucocorticoid use, or rheumatoid arthritis |
Anyone with recent fragility fracture |
3.5.4 Recent Advances in Diagnosis of Osteoporosis
Two recent advancements available in some DXA scanners have improved fracture-risk predictive capabilities. Vertebral Fracture Assessment (VFA) uses a lateral radiographic image of ideally the entire thoracolumbar spine (Fig. 3‑2). 24 The Genant visual semiquantitative (VSQ) scale is usually applied to identify individuals with mild, moderate or severe vertebral fractures. 24 In conjunction with this approach, the anterior, middle, and posterior vertebral body height at each level can be measured and compared to controls. With the VSQ system, fractures are classified as mild (20–25% height loss), moderate (25–40% height loss) and severe (>40% height loss). The VFA allows identification of occult fractures and correlates to functional deficits in BMD. For example, Muszkat reported that 17% of patients having routine DXA had moderate or severe vertebral fractures based on VFA, and of those, 88% were occult. 25

The trabecular bone score (TBS) provides an index of bone quality by serving as a surrogate of bone microarchitecture. 26 The TBS uses special software to assess bone texture and can be applied to existing lumbar spine DXA data. A region of interest identical to that for DXA is chosen, and each pixel on 2-dimensional imagesis assigned a value based on bone thresholding. The TBS is calculated based on the variation between pixels which are greater in osteoporotic bone than normal trabecular bone when compared to normal. The trabecular bone score can be independent of BMD and have greater predictive value than T-score (Fig. 3‑1e). The TBS can be utilized to improve fracture-risk estimation in FRAX, with high TBS scores reducing risk and vice versa. TBS values > 1.31 are consistent with intact bone microarchitecture, < 1.23 with degraded bone microarchitecture, and a score in between these values with partially degraded bone microarchitecture.
3.5.5 Opportunistic Screening using CT
Computed tomography (CT) is a widely used diagnostic tool. In the U.S. in 2015, there were 240 CT examinations per 1,000 patients. 27 In 2013, over 31 million patients had abdominal or pelvic CTs that examined the lumbar spine and proximal femur, which could provide useful information regarding bone mineral density. CTs obtained for other indications can be used to quantitatively assess bone status; this has been termed “opportunistic osteoporosis screening.” 28
The CT scan uses rotating X-ray emitters and detectors that allow calculation of energy attenuation in each 3-dimensional piece (voxel) of tissue. The X-ray attenuation is normalized and is calculated as the linear attenuation coefficient, known as the Hounsfield unit (HU). Each scanner calculates an HU for each voxel and is displayed on planar images using a grey scale. The X-ray attenuation is proportional to the atomic mass cubed and the number of atoms in each voxel of tissue. Thus, in general for bone, HU is proportional to the BMD.
Several methods to assess bone status using CT have been developed. The simplest is to determine the mean HU in an elliptical region of interest (ROI) using standard PACS software (Fig. 3‑1c). 29 Typically, for the spine this will be drawn in the anterior two thirds of the vertebral body on either axial or sagittal sections. A representative section, usually through midbody or through the pedicle, is selected, and a region of interest is drawn to include only trabecular bone. 29 It is important to avoid any bony defects, fractures, or bony islands. Standard thresholds to determine the likelihood of osteoporosis are determined for L1, which is also available on both chest and abdomen CTs, making it an ideal vertebra to use if available. The author recommends drawing as large an ellipse as possible. Reliability studies show no difference between averaging multiple small ellipses and drawing one large region of interest.
More accurate quantitative CT scans can be obtained by simultaneously scanning the patient and a phantom containing various concentrations of calcium hydroxyapatite. These are then used to interpolate the bone mineral density of any region of interest. This has been termed synchronous quantitative CT (qCT). 30 This is an excellent research tool but is not widely used clinically. Asynchronous qCT utilizes calibration data obtained daily on CT scanners and does not require the simultaneous scanning of patient and phantom. This approach is as reliable as standard synchronous qCT and may be used to calculate bone mineral density and to determine T-scores similarly to DXA. 30 Phantomless quantitative CT uses water and air density as standards to calculate bone mineral density. At present, this is used mainly as a research tool.
There are notable factors that affect X-ray attenuation and thus HU. CT manufacturers and day-to-day fluctuations are minor. Intravenous contrast increases spinal HU by approximately 11. 31 The most important factor is voltage tube energy of the CT scanner. 32 Most CT scans use 120 kV; however, newer scanners allow dual-energy CT scans, and a significant negative correlation exists between higher voltage and lower HU.
Thresholds have been determined to estimate the presence or absence of osteoporosis based on Hounsfield units. Pickard recommends an HU threshold for L1 vertebral body of 135, which optimizes the sensitivity and specificity. An HU greater than 160 will rule out osteoporosis, while a value less than 110 most likely indicates it. Schreiber compared HU to DXA and T-scores. He found that normal bone (T-scores > -1.0) had a mean HU of 133, osteopenic patients (T-score -1.0 to -2.5) had a mean of 101, and osteoporotic patients (T-score < -2.5) had a mean of 78.
Determining Hounsfield units has proven helpful in managing spine patients. A significant linear correlation exists between elastic modulus, apparent yield strain, and pull-out forces and HU, although thresholds have not been determined. 33 Further, Weiser found a linear correlation between bone mineral density obtained from synchronized CT and pedicle screw cycles to failure and load to failure. 34
Opportunistic CT scans have been evaluated in spine patients. Meredith reported a significant correlation between decreased preoperative HU and proximal junction kyphosis due to fracture in patients having spinal deformity. 35 Patients with fracture had mean HU of 146, compared to 199 in the nonfracture group. Du reported that the incidence of incidental durotomy was twice as high in patients with low HU, although the cause for this phenomenon was not reported. 36 Fusion success was negatively affected by osteoporosis diagnosed by HU. Schreiber evaluated 140 patients and determined that the mean HU for those with successful lumbar fusion was 203, while it was only 140 in patients with nonunion. 37 Okuyama similarly reported that union success was strongly correlated to higher bone mineral density. 38 Nguyen performed a match cohort study and found that in patients with nonunion, the HU value was significantly lower (167 vs. 201) than in those who had union. 39 Wagner found a strong correlation between CT HU and DXA in patients undergoing lumbar fusion. Twelve percent were osteoporotic, of whom two-thirds had never been diagnosed or treated. 40 Mi et al found that patients having cage subsidence after posterior interbody fusion had lower HU than those without subsidence, and at follow-up had less fusion mass. They calculated that an HU threshold in L4 of 132 identified those patients at risk for subsidence. 41
An important opportunistic use for CT scan is to identify occult fractures. In patients having abdominal CT scans, Graffy determined that 8.2% of patients had occult vertebral fractures, which strongly correlated to decreased HU. 42 Lee noted that DXA poorly predicted fractures in patients having abdominal CT scans. 27 The HU was less than 145 in 97% of patients with occult fracture, while all had DXA T-scores were greater than -2.5. Eighty-two percent of patients with occult fractures on CT were not mentioned in the radiologic reports.
Emohar compared HU in a nonfracture control group to patients with cervical, thoracolumbar, and pelvic fractures. There was strong correlation with lower HU values in patients with fracture compared to control. In a similar study, authors found that patients with ankylosing spondylitis had markedly lower trabecular HU, suggestive of osteoporosis. 43
3.5.6 Secondary Osteoporosis
Primary osteoporosis is bone loss due to aging and, in postmenopausal women, the loss of estrogens. Secondary osteoporosis occurs frequently and is due to other medical conditions and medications. It is important that laboratory testing be performed to identify secondary causes of bone loss in patients with a known diagnosis of osteoporosis or prior fragility fracture. 19 In one study, approximately 40% of patients had secondary osteoporosis contributors. 44 Simple laboratory tests include a complete blood count, a comprehensive metabolic panel, 25(OH)D, intact parathyroid hormone, phosphate, and 24-hour urine collection for calcium, sodium and creatinine. Other tests are indicated depending on history and physical findings. In patients with suspicious spine fractures, multiple myeloma should be considered and is assessed by serum and urine protein electrophoresis.
3.5.7 Bone Turnover Markers
Bone turnover markers (BTM) are proteins and peptide molecules used to assess dynamic cellular activity of bone formation and bone resorption. These markers are useful to monitor the effectiveness of treatment but not for the diagnosis of osteoporosis. Current guidelines of the American Association of Clinical Endocrinology (AACE) recommend the use of serum C-terminal telopeptide (s-CTX) to assess bone resorption and serum N-terminal propeptide of type I collagen synthesis (P1NP) to assess bone formation. 19 Patients who respond effectively to antiresorptive therapy should have lower bone turnover and thus lower BTMs. Elevated s-CTX indicates continued high bone turnover and suggests poor treatment compliance, malabsorption, or secondary causes of osteoporosis. In contrast, both formation and resorption BTMs are increased by bone anabolic therapies.
3.6 Treatment Of Osteoporosis
3.6.1 General Principles
Universal recommendations for management of patients with osteoporosis are to ensure adequate calcium and vitamin D intake, a high protein diet, treatment of any vitamin D deficiency, regular weight-bearing exercises, fall prevention, cessation of smoking and reduction of excess use of alcohol (Table 3‑5). The intervention threshold for use of pharmaceutical medications in many countries is based on fracture risk, as calculated using FRAX. With this approach, patients at high risk (cut points vary by country) should be considered candidates for medical therapy. In other regions of the world, notably the U.S., a hybrid approach of recommending treatment for those with a T-score below -2.5 and for those at elevatedfracture risk (>20 major osteoporosis related fracture or > 3% hip fracture) is utilized. Patients with major fragility fractures are candidates for either antiresorptive or anabolic therapy.
3.6.2 Supplements
Vitamin D is essential for proper bone physiology since it promotes calcium absorption, osteoblastic differentiation, osteoblastic mediated mineralization, and calcium regulation, and it collagen cross-linking. Clinically, low vitamin D is associated with sarcopenia and increased fall risk. Vitamin D is associated many other disease states such as cardiovascular disease, diabetes, hypertension, and cancer.
Vitamin D is formed in the skin upon exposure to UV radiation where 7-dehydro-cholesterol is converted to cholecalciferol (vitamin D3). To become active, cholecalciferol requires two hydroxylations. The first occurs in the liver, where vitamin D3 is converted to 25-hydroxyvitamin D [25(OH)D]. The kidneys subsequently hydroxylate 25(OH)D to the active form 1–25(OH)2 D.
Measurement of serum 25(OH)D is accepted as the best approach to determine an individual’s vitamin D status. This metabolite is chosen because it circulates at relatively high concentration and has a long half-life (~3 weeks) and reflects long-term stores. Determination of the optimal level of 25(OH)D has proven difficult because of differences in 25(OH)D assays, which prevents the performance of reasonable meta-analyses. The NIH Vitamin D Standardization Program (VDSP) has developed methodologies allowing use of standardized 25(OH)D data, so progress may be made in this regard. Additionally, but importantly, virtually all previous and ongoing randomized trials of vitamin D supplementation have failed to require volunteers to be low in vitamin D prior to study initiation. It is self-evident that providing more of any nutrient, in this case vitamin D, to individuals that are replete in that nutrient cannot possibly have benefit and could only, potentially, cause harm. In summary, systematic reviews of 25(OH)D status using various assays and also large randomized controlled trials (RCTs) with flawed methodology will not serve to define hypovitaminosis D. In this setting, a common-sense approach to defining vitamin D adequacy was to assume that communities of hunter-gathers in equatorial climates who spend most of their time with high levels of skin exposure represent evolutionary “normal” vitamin D status. In Tanzania, hunter-gather tribes were found to have a mean 25(OH)D of 46 ng/mL. Similarly, surfers in Hawaii had a mean 25(OH)D level (using retrospective assay standardization as recommended by the VDSP) of approximately 36 ng/ml.
Importantly, there is substantial between-individual response in the 25(OH)D increase achieved following initiation of an individual dose of daily vitamin D supplementation. In general, it is recommended to choose larger doses so that as many patients as possible achieve a normal level. Understandably, based on the issues noted above, it is to be expected that expert opinion regarding the optimum 25(OH)D level varies. The 2011 Institute of Medicine report considers a 25(OH)D level of ≥ 20 ng/mL to be adequate, whereas recent AACE guidelines recommend >30 ng/mL as adequate while calling levels between 20 and 30 ng/mL insufficient and those < 20 ng/mL deficient (Table 3‑6). 23 , 45
While controversial, many experts believe that the two available forms of vitamin D (D3, cholecalciferol and D2, ergocalciferol) vary in potency. Some, though not all, studies find supplementation with vitamin D3 to be more potent at increasing circulating 25(OH)D; as such, it is generally preferred for treatment. Cholecalciferol is widely available as a stand-alone supplement in doses of 1,000, 2,000 and 5,000 IU. Additionally, 50,000 IU D3 supplements are available. Vitamin D2 is available in similar doses and is the only form available in the U.S. by prescription. The AACE guidelines recommend 1,000 to 2,000 units per day for general population. 19 Patients who are vitamin D insufficient (less than 20 ng/mL) are treated either with 50,000 units of vitamin D2 or D3 per week for eight weeks or 5,000 IU of vitamin D3 daily. At 12 weeks, the 25(OH)D level is checked to assure that it is greater than 30 ng/mL. Note that it is important to wait at least 12 weeks to reach a new 25(OH)D steady state given the ~3 week half-life of 25(OH)D. Subsequent to attaining an optimal 25(OH)D level, and one similar to the normal population, 1,000 to 2,000 units of vitamin D3 are recommended as a daily maintenance dose. Alternative doses may be required in patients with obesity, malabsorption, or who are taking medications that affect vitamin D metabolism. Indeed, it is not possible to estimate an individual’s intake given the difference in 25(OH)D response noted above; as such, in individuals who have sustained fractures, it is prudent to recheck a 25(OH)D level following initiation of a daily maintenance dose.
Like most issues in the vitamin D literature, the definition of toxicity is controversial. Nonetheless, 25(OH) D levels up to ~150 ng/mL are generally well tolerated without evidence of toxicity. The acute toxicity of vitamin D is elevated serum calcium. Massive doses of vitamin D are unwise, as one study of 500,000 IU found this to increase risk of falls and fractures; the mechanism(s) of these unexpected findings are unknown.
Finally, while controversial, it is widely believed that vitamin D deficiency leads to muscle weakness, with resultant increased fall risk. As such, vitamin D supplementation of older adults with a history of falls or at increased fall risk is recommended by both the American Geriatrics Society and the USPSTF. 23 , 46 If indeed vitamin D deficiency leads to muscle weakness, it conceivably could contribute to the development of sarcopenia, the age-related reduction in muscle mass and strength. Sarcopenia is increasingly recognized as an important contributor to “osteoporosis-related” fractures due to increased fall risk.
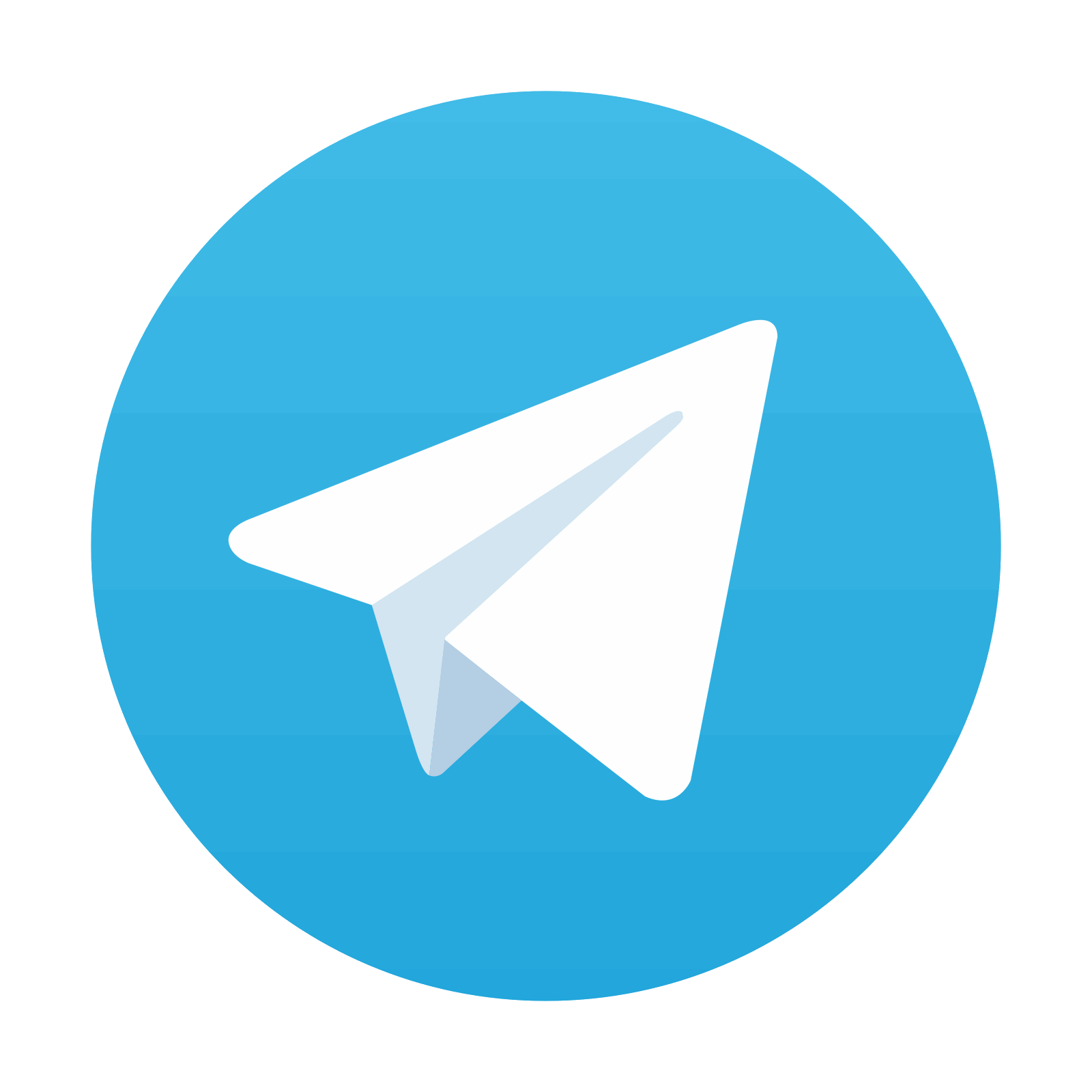
Stay updated, free articles. Join our Telegram channel

Full access? Get Clinical Tree
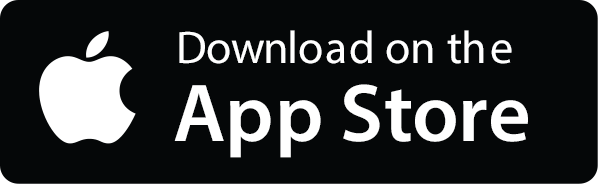
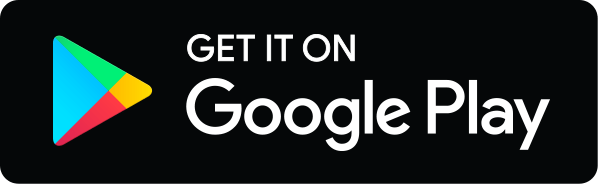
