2 Neuropathology of human hydrocephalus
Adult Hydrocephalus, ed. Daniele Rigamonti. Published by Cambridge University Press. © Cambridge University Press 2014.
Introduction
Hydrocephalus can be defined as a pathologic enlargement of the intracranial cerebrospinal fluid (CSF)-filled spaces. Usually this refers to the cerebral ventricles, but enlargement of the subarachnoid space can be an associated phenomenon. Most CSF is produced by the choroid plexus, while ~20% is water derived from brain as product of glycolysis [1] and movement of water across the blood–brain barrier [2]. Absorption of CSF is via arachnoid villi, which are mainly associated with venous sinuses, as well as via lymphatics associated with the exit sites of cranial and spinal nerves. The relative contribution of the two routes is likely species specific; the exact proportionate role in humans is not known [3,4]. CSF has multiple functions including cleansing of potentially noxious byproducts of brain metabolism, a route for molecular communication, and protection of brain by cushioning from vascular and extraneous shock waves [5,6]. It should be noted that the relative density of brain (1.036 g/cm3) compared to CSF (1.006 g/cm3) precludes the brain actually floating in CSF.
The monographs by Russell [7] and by Milhorat [8] summarize well the malformation, post-inflammatory, post-hemorrhagic, and tumor-associated causes of hydrocephalus. The author has reviewed the pathology and pathophysiology of hydrocephalus previously [9,10]; most of the recent reviews focus on developmental aspects particular to hydrocephalus in the immature brain including relationships to spina bifida and associated cerebellar changes, as well as potential pharmacologic interventions [11–13]. The reader is referred to these and other reviews [14,15] for a more comprehensive coverage of the subject. This chapter will focus on aspects of hydrocephalus that are common to all ages with a particular emphasis on the aging brain and the so-called normal pressure hydrocephalus (NPH) syndrome, which is a chronic disease that likely evolves over a period of years. It has been clear for decades that the rate of ventricular dilatation and the state of brain maturation have a significant impact on the pathology, and possibly the pathogenesis of brain damage. It is critical to recognize that the vast majority of experimental work related to hydrocephalus has utilized immature or young adult animals in which the ventricles dilate over a period of days to weeks. The extent to which this information can be generalized to a chronic disease of aging brain is not clear and the reader must use caution. Limitations and valid aspects of the animal models are revealed by autopsy descriptions of adults with chronic hydrocephalus including NPH [16–40].
Factors leading to ventricular enlargement
Idiopathic NPH has been distinguished from chronic adult hydrocephalus associated with prior meningitis, brain trauma, or subarachnoid hemorrhage (secondary NPH) [41]. This may be useful from a clinical and prognostic vantage, but from a pathophysiological perspective there is likely a spectrum rather than a boundary. Mild head trauma or “aseptic” meningitis, forgotten after decades, could create the basis for eventual impairment of CSF movement. Even mild subarachnoid hemorrhage causes inflammation and cellular proliferation in the subarachnoid compartment [42]; this, added to the gradual leptomeningeal fibrosis that occurs with aging [43], may eventually serve as a detriment to CSF movement. Brain biopsies on patients being investigated for NPH showed arachnoid fibrosis in approximately half the cases [44]. The fact that this did not correlate with CSF outflow resistance is not surprising given the limited sample. In addition to changes in the subarachnoid compartment, another potential factor to explain the onset of symptoms in the elderly is the parallel accumulation of brain tissue and vascular changes associated with aging [45–47].
It is now apparent that some individuals with NPH have larger heads than unaffected individuals; they might have previously unrecognized congenital hydrocephalus that becomes symptomatic only late in life [48,49]. Discussion of the CSF forces that cause the ventricles to dilate is beyond the scope of this chapter although it should be noted that experimental studies show that very small pulsatile forces, only slightly greater than the normal pulse pressure, are capable of compressing the brain [50–52].
Consequences of ventricular enlargement and pathogenesis of brain damage in hydrocephalus
As the ventricles enlarge, adjacent brain tissue is displaced and later stretched or compressed (depending on the location). The initial displacement (e.g. elevation of the corpus callosum) may be at the expense of the subarachnoid compartment (especially if there is brain atrophy), the venous compartment, and the extracellular compartment with negligible damage to brain cells. Initially the opposite surfaces of the ventricles are spread apart but there is no change in the surface area. Beyond this threshold, when the surface area of the ventricles is forced to expand (Figure 2.1), periventricular white matter begins to sustain injury and functional changes become apparent. Atrophy of the ependymal lining of the ventricle surface is one of the first histopathological changes. However, this can be caused by a variety of factors and consequences in the adult brain are likely negligible [53].
Figure 2.1 Diagram explaining the postulated threshold effect for tolerance to ventriculomegaly. In the normal state the ventricles are narrow. In the early presymptomatic stages of normal pressure hydrocephalus (NPH), the ventricle volume increases considerably by displacement of the ventricle walls but there is no stretching of the ventricle surface. In the symptomatic phase, the ventricles might be only marginally larger but the periventricular structures are stretched and experience decreased blood flow.
NPH and white matter and cerebrovascular disease
Many imaging studies on NPH patients have shown decreased cerebral blood flow, especially in white matter [54]. Experimental studies in young adult dogs and rabbits suggest that this might be due to compression or constriction of the microvasculature [55,56]. This is likely more than a simple physical compression; regulation of blood flow at the local level is highly complex involving numerous signaling mediators, innervation, and interactions between astrocytes and blood vessels [57]. Calcium ion distribution and nitric oxide production are altered in experimental hydrocephalus [58,59]; although both could be involved in regulation of vascular tone, the hypothesis has not been directly tested in hydrocephalus. Studies of CSF from hydrocephalic humans provide evidence for hypoxic metabolism and lipid peroxidation [60,61]. As a consequence of these changes, oligodendrocytes, myelin, and axons are subject to hypoxic-ischemic stress and injury [59]. The molecular processes that damage axons are similar to those that follow ischemic stroke and head injury.
Imaging studies show atrophy of the fornix and corpus callosum in NPH patients [62]. We reported similar abnormalities in an autopsy study that included the full age spectrum of hydrocephalic patients [63]. Selective analysis of elderly brains from the same dataset shows that the corpus callosum and fornix are atrophic (Table 2.1). In general, however, the most severe ventricular enlargement and white matter atrophy associated with NPH are less than in the most severe chronic childhood-onset hydrocephalus cases. Compounding the situation in the aging brain is the accumulation of vascular changes associated with arterial hypertension and atherosclerosis. Many studies show an association between NPH and vascular disease [64–66], as well as diabetes [67]. However, the presence of white matter “vascular” abnormalities on imaging does not preclude a good response to shunting [68,69]. Although it is well known that rats with spontaneous arterial hypertension (SHR strain) develop moderate enlargement of the ventricles [70], the additive effect between hydrocephalus and cerebrovascular disease has not been addressed experimentally.
Table 2.1 White matter changes in chronic adult hydrocephalusa, b
a The symptomatic hydrocephalus cases are compatible with a diagnosis of NPH. Most measurements were made on the coronal slice of the frontal lobes at the level of the caudate nucleus head. The data are shown as mean ± standard error of mean. Statistical comparison versus control used the Student t test; *p < 0.05, **p < 0.01.
b There was no difference between groups with respect to thickness of the periventricular layer of astrocytes (0.19 ± 0.05 mm), thickness of the alveus (0.31 ± 0.01 mm), or thickness of the frontal cortex (2.5 ± 0.1 mm). No case had more than minimal Alzheimer’s disease neuropathologic change (i.e. senile plaque density sparse).
c Lateral ventricles were measured across the frontal horns at the horizontal level of the mid-caudate nucleus head.
Hydrocephalus and the extracellular fluid
In addition to the cascade of damage caused by diminished periventricular blood flow, alterations in fluid movements can cause changes in composition of the brain extracellular environment. Metabolic byproducts that cannot be recycled or moved across the blood–brain barrier normally flow to the ventricles whereupon they are washed away with CSF flow [6]. There is an accumulation of waste products, degeneration products, and neuromodulatory agents in CSF and brain tissue of hydrocephalic patients [60,71,72]. In hydrocephalic rats the cortical extracellular fluid flow is reduced [73,74], in rats and mice the extracellular spaces of gray matter are reduced in size [75,76], and in rabbits the cerebral cortex is compressed [77]. However, an electron microscopic study of the cortex from NPH patients showed enlargement of the extracellular space in 9/11 cases [78]. It is difficult to reconcile the discrepancy between the human and experimental animal findings. Morphologic and imaging studies indicate that the extracellular compartment is enlarged in periventricular white matter [79]. Disturbance of the extracellular volume and composition has the potential for impairing neuron and axon function. Reversal of this disturbance might account for some of the rapid improvement that occurs following shunting.
NPH and Alzheimer’s disease
One of the main neuropathological features revealed in large series of NPH patients is the coexistence of Alzheimer’s disease type changes, including senile (neuritic) plaques and neurofibrillary tangles. These have been demonstrated in cortical biopsies taken at the time of intracranial pressure monitoring or shunting [80,81], as well as in autopsies. This is the subject of an excellent recent review [23]. The biopsy studies and the larger series of autopsies (9–16 cases each) on NPH patients show that coexisting Alzheimer type changes and/or cerebrovascular changes do not necessarily predict failure of response to shunting [22,23,35]; however, moderate to severe Alzheimer type changes are associated with worse baseline cognitive performance and diminished postoperative improvement following shunting [82].
The only experiments that come close to investigating the combined effects of hydrocephalus and aging are those by Klinge, Silverberg, and coworkers. One-year-old rats were rendered hydrocephalic by injection of kaolin into the cisterna magna; over the following 10 weeks the brains had accumulations of amyloid beta protein and phosphorylated tau protein, both of which play a role in the pathogenesis of Alzheimer’s disease [83,84]. Given that the average lifespan of rats is >2 years, these studies barely met their stated objective; nevertheless they do support the hypothesized interplay between extracellular fluid flow and the development of Alzheimer type pathological changes [85]. The relationship is not a simple one though. A prospective, randomized, double-blinded trial failed to show any benefit of a surgically implanted shunt in subjects with probable Alzheimer’s disease [86].
NPH and autopsy findings
The question of disease coexistence with hydrocephalus was further addressed by retrospectively studying brains with ventricular enlargement in a population-based (rather than disease selected) autopsy series (Table 2.2 and Figure 2.2). From 1996 to 2012 the author has performed detailed brain examinations on 5 patients with a clinical diagnosis of idiopathic NPH, 3 patients with a clinical diagnosis of secondary NPH, and 20 patients in whom ventriculomegaly could not readily be explained by other disease processes identified. In the first two groups, 6/8 patients had been shunted; all had resolution of their gait abnormalities and incontinence, but not their cognitive deficits. Senile plaques were present in 6/8 cases, and cerebrovascular changes in 4/8. From the neuropathology perspective, there was no difference between the idiopathic and secondary NPH cases. Among the other 20 cases, the age and magnitude of ventricular enlargement was similar to those with a clinical diagnosis of NPH. As with the NPH groups, Alzheimer’s disease type changes and cerebrovascular abnormalities were often present. The presence or absence of such changes could not explain the lack of symptoms in 9/20 individuals (although it must be noted that detailed histories were not always available). In retrospect, one individual who had ataxia and dementia might have had NPH (case C16 in Table 2.2). Considering all three groups together, 9/28 cases had prominent dorsal grooving of the corpus callosum caused by its elevation and impingement on the falx cerebri. Of interest is the identification of three patients (cases A2, C6, C8 in Table 2.2) with parkinsonism (in one developing 2 years after shunting); none had Lewy bodies or synuclein immunoreactive deposits characteristic of Parkinson’s disease, nor evidence of other neurodegenerative disease associated with parkinsonism. It is known that the clinical distinction between NPH and Parkinson’s disease can be difficult [87], and in some cases the parkinsonism can respond to shunting [88]. In this circumstance projections from the substantia nigra to the striatum might be compromised [89]. Three patients had abnormal narrowing of the cerebral aqueduct as the likely cause of hydrocephalus (cases A5, C13, C17 in Table 2.2), consistent with the current knowledge that some NPH patients might be delayed presentations of congenital anomalies [48]. In general, however, a structural cause for hydrocephalus was not always apparent despite careful inspection of the meninges and arachnoid granulations.
Figure 2.2 Photographs of coronal slices of hydrocephalic brains at the frontotemporal level highlighting the unpredictability of functional impairment. The upper slice is from a patient with clinical diagnosis of idiopathic NPH (case A5 in Table 2.2). The inset shows the cerebral aqueduct partially obstructed by granular extrusions, likely the consequence of an irritating process (possibly mild ventriculitis) many years earlier. The middle slice is from a patient with clinical diagnosis of secondary NPH (case B3 in Table 2.2). The shunt (piercing the septum pellucidum) was no longer functional and the symptoms had recurred. The lower slice is from an individual whose ventricles had enlarged considerably over the preceding 9 years but was asymptomatic (case C13 in Table 2.2). The septum pellucidum is destroyed and the corpus callosum thinned.
Table 2.2 Summary of neuropathologic autopsy findings in chronic adult hydrocephalus casesa
(A) Clinical diagnosis of idiopathic normal pressure hydrocephalus
(B) Clinical diagnosis of normal pressure hydrocephalus secondary to known cause
(C) Hydrocephalus at autopsy in adults with no neurologic complaints or atypical history (none were shunted)
a Retrospective review of unselected autopsy cases with complete brain examination in Province of Manitoba 1996–2012 (approximately 300–350 adult brains per year, hospital and medicolegal cases). Excluded were those cases with overt malformation, neurologic problems dating back to childhood, history of severe head injury or cerebrovascular event, and acute causes. None of the cases presented here were included in a previously reported series [28]. Precise measurements of the ventricle sizes are not available.
Secondary reactive changes due to hydrocephalus
When the cerebral ventricles expand, damage to periventricular white matter stimulates prominent reactive changes among glial cell populations including activation and proliferation of astrocytes and microglia [9]. In chronic adult hydrocephalus including NPH, the rate of ventricular enlargement is slow (e.g. years) and such reactive changes are usually subtle. When varicose axons are identifiable (especially using immunohistochemical detection of neurofilament or amyloid precursor protein, which accumulates at sites of axonal compromise), one may also recognize reactive microglia using appropriate immunohistochemical labeling (e.g. HLA-DR, CD68, or Iba1). Rarely phagocytosed myelin debris can be demonstrated (e.g. with the Marchi silver precipitation method [90]). Reactive astrocytes increased their expression of the water channel protein aquaporin-4, especially in their endfeet where blood vessels are contacted. This has been demonstrated in many of the animal models and most recently in biopsies from NPH patients [91]. This is of potential relevance to compensatory water movements in the hydrocephalic brain.
Altered neurons and connections in the hydrocephalic brain
Brain function is ultimately determined by the integrity of connections between neurons. Axons in the periventricular white matter are the main target for damage due to hydrocephalus. Therefore, connections within the cerebrum, between the hemispheres, and to the brainstem and spinal cord are potentially compromised. The structure–dysfunction relationship in young hydrocephalic brains, about which there is considerable information, has been reviewed in detail recently [13]. The gait abnormality in NPH may be due to dysfunction of the supplementary motor cortex and possibly connections with the basal nuclei [92,93]. Functional MRI studies indicate that sensation related to urinary bladder filling occurs in the insular cortex, the anterior cingulate gyrus provides monitoring and control, and the prefrontal cortex makes voiding decisions [94]. Adults with hydrocephalus appear to have cognitive abnormalities attributable to changes in the septohippocampal circuit, while those with NPH seem to have dysfunction of prefrontal structures [95]. All of these dysfunctions can be explained by the periventricular white matter damage and subcortical disconnection. Long pathways extending to the brainstem and spinal cord can also be damaged [63,96,97].
Most histopathological studies of neuron changes in hydrocephalic brains have been conducted in young animals. Neuron death is infrequent unless the white matter is completely destroyed and the deep cortical layers exposed to the ventricles [98]; this seldom happens in NPH. Disconnected neurons have fewer synapses and their dendritic complexity is reduced. This too is likely subtle in NPH. In contrast to Alzheimer’s disease patients, people with NPH do not have significant atrophy of the hippocampus [99]. Adult hydrocephalic rabbits have changes in monoamine neurotransmitter concentrations including decreased dopamine in the hypothalamus and medulla, decreased serotonin in the basal nuclei, and increased norepinephrine in the thalamus [100]. Although neuronal changes in the striatum are not typically identifiable at the microscopic level, positron emission tomography studies in NPH patients indicate a correlation between postsynaptic dopamine (D2) receptor hypoactivity in the putamen and the severity of gait impairment [101,102]. Autopsy studies have demonstrated nonspecific degenerative changes in the substantia nigra of adults with chronic hydrocephalus [28].
Summary
Neuropathological changes in NPH suggest that the pathogenesis of white matter damage (and secondary neuronal disconnection) is similar to that in young hydrocephalic brains, but in general less severe. Blood flow hypoperfusion leads to hypoxic-ischemic changes in the white matter, physical stretching compromises axon integrity, and retarded turnover of the CSF alters the extracellular microenvironment. The additive effects of age-related cerebrovascular disease and neurodegenerative disease (especially Alzheimer’s) contribute to the brain dysfunction and perhaps help explain distinctive aspects of the clinical syndrome. There is potential for reversal of some symptoms, often without substantial reduction in ventricle size, likely through correction of white matter hypoperfusion and normalization of the extracellular environment. To gain a greater understanding of the brain damage in NPH, detailed analysis of autopsy material along with experimental studies in aged animals is required.
Conflict of interest disclaimer
The author has in the past received consulting fees from Codman and Medtronic, both in relation to development of shunt technology.
Funding support
Dr. Del Bigio holds the Canada Research Chair in Developmental Neuropathology.
References
























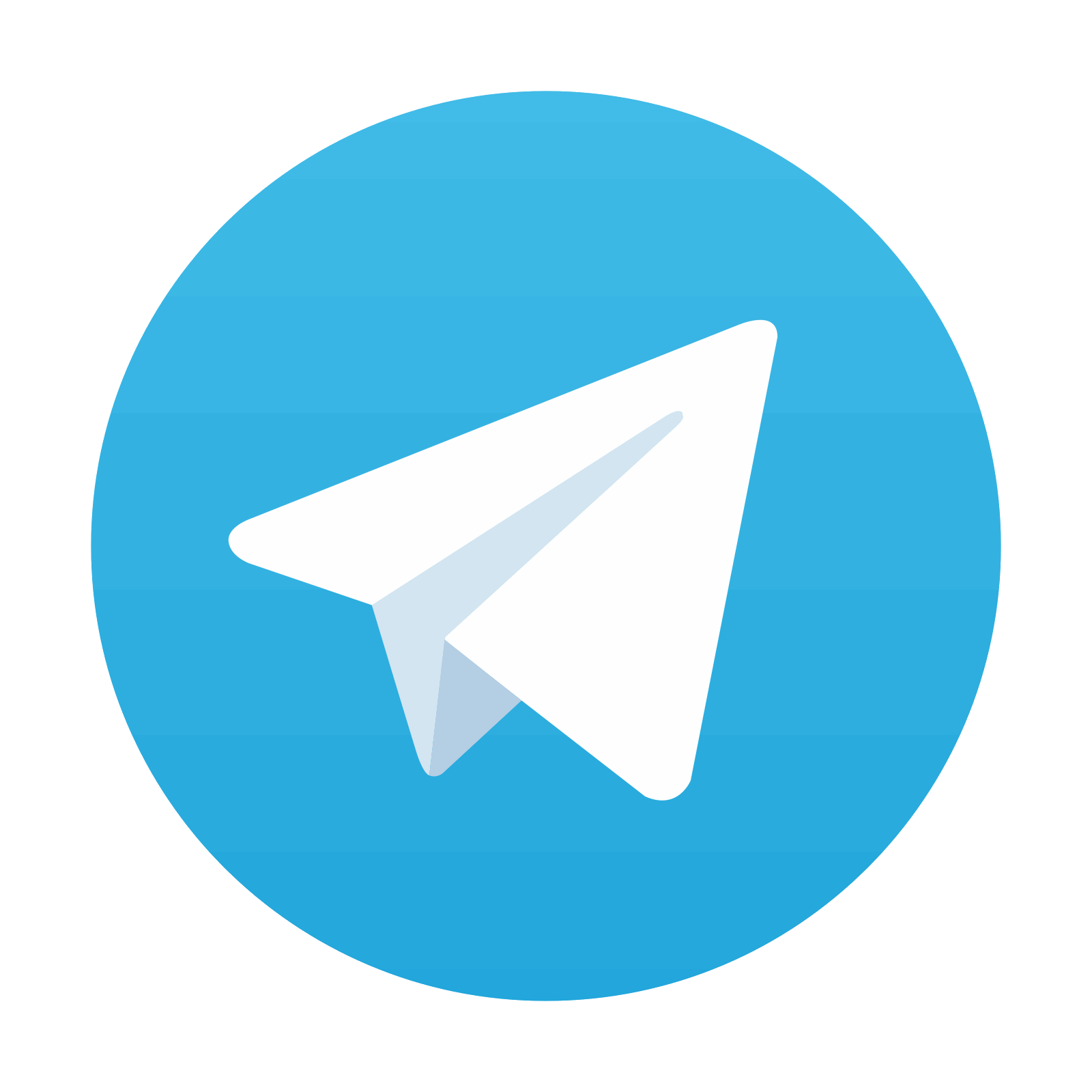
Stay updated, free articles. Join our Telegram channel

Full access? Get Clinical Tree
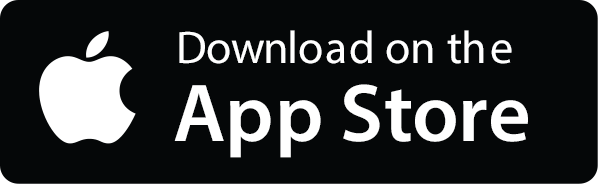
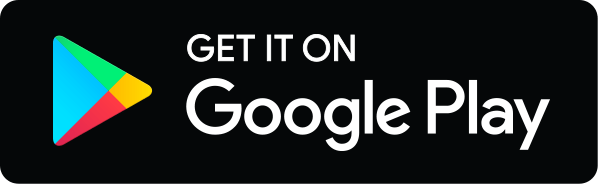
