21 Extratemporal Resection
The safety and efficacy of surgery for temporal lobe epilepsy has been well established and represents the paradigm for resective epilepsy surgery.1–5 By contrast, surgery for epilepsy of extratemporal origin poses several unique challenges, which require the team of treating physicians to engage in a rigorous preoperative workup often culminating in a technically demanding operative procedure, with traditionally less successful outcomes regarding seizure freedom. Surgery for medically refractory partial extratemporal epilepsy involves cortical resection of the areas of ictal onset and seizure propagation anywhere outside of the temporal lobe. It is the more common type of epilepsy surgery performed in children. Although anteromesial temporal lobectomy for temporal lobe epilepsy is known to carry a reasonably low morbidity, the resections necessary for extratemporal seizure foci typically involve larger areas of cortex, which often may either encompass or abut functionally significant regions of the brain.
Although much of the literature pertaining to extratemporal epilepsy surgery describes treatment of frontal lobe epilepsy, all cortical areas outside of the temporal lobe have the potential to be involved. The ultimate surgical plan, which should ideally be devised by a multidisciplinary team in the setting of a comprehensive epilepsy center, is based on a multitude of factors and includes a consideration of pathological substrate, neuroimaging data, electroencepha-lography (EEG)/neurophysiological information, functional mapping data, and the specific risk–benefit profile of the individual patient. Published rates of seizure freedom after surgery for extratemporal epilepsy vary between 30 and 80%, compared with more than 80% for temporal lobe epilepsy.6–13 However, the treatment goals are the same: the reduction or elimination of seizures with minimal morbidity, as well as the preservation or improvement neurocognitive function. Several published studies have demonstrated both the safety and efficacy of epilepsy surgery in children.6–9
Unique Considerations in Pediatric Extratemporal Epilepsy Surgery
There is a growing body of literature on extratemporal epilepsy surgery in children. This patient population warrants special consideration for several reasons. First, the pathological substrate differs in the adult and pediatric populations. The most common cause of intractable partial epilepsy in adults is hippocampal sclerosis, classically treated surgically by anterior temporal lobectomy with amygdalohippocampectomy. In children, however, the predominance of epilepsy of extratemporal origin is related to developmental brain abnormalities (e.g., cortical dysplasia, tuberous sclerosis complex, Sturge-Weber syndrome) and low-grade cortical tumors (e.g., gangliogliomas, desmoplastic infantile ganglio-gliomas, oligodendrogliomas, astrocytomas).14,15 Cortical dysplasia is especially prominent in the pathological specimens of children who undergo resections for extratemporal epilepsy.15 By contrast, adults who undergo extratemporal resections are typically found to harbor a different pathological profile than children, often including such variable entities as gliosis and focal cell loss. Although good outcomes have been reported for adults after extratemporal epilepsy surgery, they appear not to fare as well after surgical intervention as children do.16–22
Second, the treating physician must take into account the developmental implications of intervention on an affected child with a still-developing nervous system. Although the developing brain is very sensitive to the detrimental effects of recurrent seizures, with potentially permanent neuropsychological and cognitive sequelae, the plasticity of the developing brain also lends itself to better functional recovery after cortical resections that may involve eloquent cortex.23–25 Nevertheless, it is now increasingly appreciated that uncontrolled epilepsy in childhood can have a detrimental effect on a child’s intelligence and cognitive abilities and, moreover, that epilepsy surgery performed in childhood may play a critical role in enhancing development and overall quality of life.26 Finally, because medically refractory partial seizures are unlikely to remit when an adequate response is not achieved with the first two major antiepileptic medications administered, early surgery is now often advocated for both adult and pediatric populations at many centers.1,14,16,25,27
Evaluation
Because of the pathological complexity of extratemporal epilepsy, patients who are being considered for surgery are best evaluated in a comprehensive epilepsy center by a multidisciplinary team of epilepsy neurologists, epilepsy surgeons, neuropsychologists, psychiatrists, and social workers. Typically, the preoperative evaluation consists of a comprehensive battery of tests all designed to localize the epileptogenic zone. A thorough understanding of the potential strengths and limitations of each of these techniques is critical for appropriate patient selection and satisfactory surgical outcomes.
Noninvasive Modalities
Routine structural imaging with magnetic resonance imaging (MRI) together with scalp video-EEG (VEEG) comprise the most basic requirements of any preoperative epilepsy evaluation. However, other noninvasive techniques are now commonly used to localize the ictal onset zone and map functionally eloquent cortex. These include magnetoencephalography (MEG), single photon emission computed tomography (SPECT), and positron emission tomography (PET).
Although invasive video-EEG (iVEEG) with subdural electrodes is felt to be superior in localizing extratemporal ictal onset zones in children undergoing resective surgery for refractory epilepsy, other studies have shown the promise of MEG as a technique for localizing the epileptogenic zone.28–33 This may partially be explained by a differential sensitivity to radial compared with tangential pacemakers, making these techniques complementary.32–34 The resection of remote ictal zones identified by MEG and electrocorticography (ECoG) does not contribute to epilepsy outcome in patients with tumors. However, in patients with cortical dysplasia, the resection of remote ictal regions may be critical to seizure outcome.29
SPECT has become a common tool in the evaluation of refractory epilepsy in children.35 subtractionictal SPECT scanning coregistered to MRI imaging (SISCOM) improves the sensitivity of ictal SPECT for localization of the ictal onset zone and has demonstrated clinical utility in guiding placement of intracranial electrodes.36–38 Ictal SPECT and SISCOM may be especially useful in providing preoperative guidance for intracranial electrode placement in children with frontal lobe epilepsy, because rapid seizure spread often renders false-localizing clinical and electrophysiological data.39,40
PET uses radiolabeled tracers to image cerebral perfusion, glucose and protein metabolism, and γ-aminobutyric acid (GABA) and serotonin receptor density.41 Interictal 2-deoxy-2[18F]fluoro-D-glucose (FDG)-PET has a reported sensitivity of 60–80% for identification of foci of hypometabolism among patients with chronic, refractory extratemporal epilepsy and a normal MRI; this sensitivity is similar to that reported for similar patients with ictal SPECT.42,43 However, the clinical utility of FDG-PET in presurgical seizure focus localization is limited by low specificity relative to iVEEG. Studies in children with tuberous sclerosis complex suffering from chronic, refractory epilepsy have shown that FDG-PET may complement MRI with diffusion-weighted imaging in differentiating epileptogenic from clinically silent tubers.44 In addition, α-[11C]-methyl-L-tryptophan (AMT), which images tryptophan metabolism, has shown promising results in preliminary clinical studies aimed at distinguishing between epileptogenic and electrically silent lesions in children with multifocal pathology.45
Functional Mapping
Precisely identifying eloquent cortex essential for sensorimotor, language, and memory function, as well as defining the anatomical relationship between ictal foci and functional centers, is critical for surgical risk assessment and decision making in extratemporal epilepsy. Several noninvasive imaging techniques, including functional MRI, MEG, and FDG-PET, provide accurate maps of primary sensorimotor cortex in children. Functional mapping may also be performed intraoperatively via direct cortical stimulation or extra-operatively via implanted subdural electrodes. Wada testing (intracarotid amobarbital test) is useful for establishing the laterality of language and memory function in cooperative children. In addition, a thorough neuropsychological evaluation is essential not only for providing a preoperative baseline but also for potentially providing corroborative data to a suspected ictal focus. Comparison of preoperative and postoperative neuropsychiatric tests may help determine whether ictal behavior has been altered.46,47
Surgery
The major factors that potentially complicate the surgical intervention in extratemporal epilepsy include the multifocality of seizure foci, the higher incidence of nonlesional MRI-negative epilepsy, and the frequent proximity of the epileptogenic zone to eloquent cortex. As a result, surgical strategies should consider a wide array of therapeutic options, which must be tailored to the individual patient’s risk–benefit profile.
When the preoperative comprehensive evaluation does not reveal an apparent localized seizure focus that can be approached surgically, the treating team of specialists is left with a dilemma regarding how to proceed. The options include either no surgery or the use of a palliative procedure, such as vagal nerve stimulation or corpus callosotomy, in the appropriate clinical setting. At our institution, we have offered selected patients, in whom we highly suspect partial seizures, but in whom we have nonetheless found non-localizing data, bilateral strip electrode survey studies to lateralize and localize their seizures. This is accomplished in a two-stage fashion, in which either a vertex craniotomy of bilateral craniotomies are performed to insert subdural strip electrodes in a survey fashion over the cortical surface ( Fig. 21.1 ). This technique has been useful in both lesional and nonlesional cases, in our experience, and has led several patients to successful resections once the ictal onset zone can be localized. For example, in our extratemporal tuberous sclerosis complex patients, we have performed this technique successfully in patients not considered to be classic epilepsy surgery patients based on their nonlocalizing preoperative evaluations. If the bilateral strip study reveals a unilateral focus, this is then approached at a later date, with a classic two-stage procedure consisting of initial grid placement, followed by resection of the ictal focus.

Traditionally, the goal of surgical management of patients suffering from medically refractory partial epilepsy has been cortical resection of the defined epileptogenic zone. This may be accomplished in one of several ways, from a surgical standpoint: single-, two-, or multistage approach. Patients harboring a radiographic lesion, which is identified as the clear source of seizures by the comprehensive preoperative evaluation, can be treated with a single-stage lesionectomy.
If concerns exist that the epileptogenic zone extends beyond the boundaries of the radiographic lesion, then one can identify the extent of ictal zone by either using intraoperative ECoG or by placing a subdural grid over the lesion to define this extraoperatively. Intraoperatively, ECoG may assist in the identification of the epileptogenic zone. Limitations of this technique include analysis of interictal data only and significantly diminished interictal spike frequency under general anesthesia. Technically, this procedure is similar in adults and children: electrodes are placed over the putative epileptogenic zone and interictal electrical spike activity is characterized. It is generally reliable if an interictal spike frequency reaches at least one spike per minute. This technique is widely applied in children with brain tumors and refractory epilepsy, where the ictal onset zone is frequently localized to cortex adjacent to the tumor and the resection of perilesional cortex with abnormal interictal spike activity correlates with long-term seizure freedom.48,49
Although there are clearly limitations to performing intraoperative ECoG in awake pediatric patients, chronic implanted subdural electrodes not only appear to be well tolerated in this population but may also yield more useful data with the longer sampling times.25,50 With the two-stage approach, the epilepsy team is able to develop a map of the ictal zone and the associated epileptogenic network that would need to be addressed surgically ( Fig. 21.2 ). Extraoperative ictal focus mapping via stimulation across subdural electrodes (arrays of 2.5-mm platinum electrodes either 5 mm or 10 mm apart, mounted on Silastic strips, implanted under general anesthesia) is a well-established technique.51,52 Reports in children with extratemporal epilepsy indicate a sensitivity of approximately 90%.53 A significant advantage is the ability to capture ictal and interictal events over several days of monitoring. Conversely, a disadvantage is the need for an additional operation for electrode implantation.51 Most centers report an overall complication rate of 10 to 15% among patients with chronic intracranial electrodes.54–59
The reported complications include cerebrospinal fluid (CSF) leak or positive CSF cultures, usually in the absence of clinically evident meningitis.50,55,56,58 Other reported complications include transient neurological deficit, epidural or subdural hematoma, and stroke. A reduction in complication rate with increasing surgical experience and lower complication rates in children have been reported.54,57 Class 2 data indicate that dexamethasone may reduce cerebral swelling in children with implanted subdural grid electrode arrays. However, it also decreases the seizure frequency, which may lead to longer extraoperative monitoring periods to capture sufficient data to localize the ictal focus.60 Studies of the pathological changes seen in cortex underlying subdural arrays have revealed focal, transient aseptic meningitis in all patients. However, the severity of this reaction does not correlate with the incidence of infection or long-term surgical outcome.61

In addition to the seizure information, functional data can be obtained using the same grid with extraoperative functional mapping. In many of these patients, the extent of resection of the seizure focus may be limited by its extent of involvement of eloquent cortex. Because awake cortical mapping may not be possible in younger pediatric patients, other modalities such as extraoperative grid mapping, MEG, or functional MRI may be necessary to define eloquent cortex. Once these regions have been satisfactorily mapped, the surgeon can discuss the various treatment options with the patient’s family in light of the specific risk–benefit considerations: resection, multiple subpial transections, or no resection. The refinement of neuronavigational techniques over the last several years has also aided the epilepsy surgeon. Both frameless and frame-based systems have been used in placing monitoring electrodes, particularly depth electrodes, and to aid in resection. Three-dimensional MRI reconstructions, functional imaging data, and MR angiography have all been used effectively to help delineating the extent of resection.62
For a select group of patients with the most complex manifestations of extratemporal epilepsy, we have advocated the use of a multistage surgical approach.50 This typically consists of electrode implantation, an extraoperative monitoring period, resection and electrode reimplantation, a second monitoring period, and a third operation for electrode removal and further resection if necessary ( Fig. 21.3 ). The additional monitoring period often reveals secondary ictal foci that only become apparent after removal of the primary seizure focus. Criticisms of this philosophy include the additional risk of further invasive monitoring and an additional surgery. In our experience, a second intraoperative monitoring period followed by a third surgical stage is simpler than a return to the operating room several months later. In this select group of pediatric patients with poor surgical prognostic factors (i.e., multifocal ictal onset, ictal overlap with eloquent cortex, or previous surgical failure), we believe that this additional stage has the potential to improve surgical outcomes. We have postulated that it is difficult to assess adequately the potential of secondary seizure foci to become independent ictal generators once the primary focus has been resected. Multifocal epilepsy involving purely extratemporal or temporal and extratemporal regions of seizure onset is common in the pediatric population. In addition, acute seizures after ictal focus resection reflect a poor prognosis.63–65 This supports the strategy of multistage procedures, in which secondary ictal foci not evident during the initial invasive monitoring period may be identified in a second monitoring session and treated surgically with a second resection.

An alternative method for localizing secondary foci is intraoperative ECoG after resection. However, this method relies on interictal data under general anesthesia as opposed to capturing ictal and interictal events over a longer period of time. Nonetheless, the justification for a multistaged surgical procedure remains controversial and necessitates additional study before any conclusions regarding treatment protocols in this difficult subset of patients can be drawn.
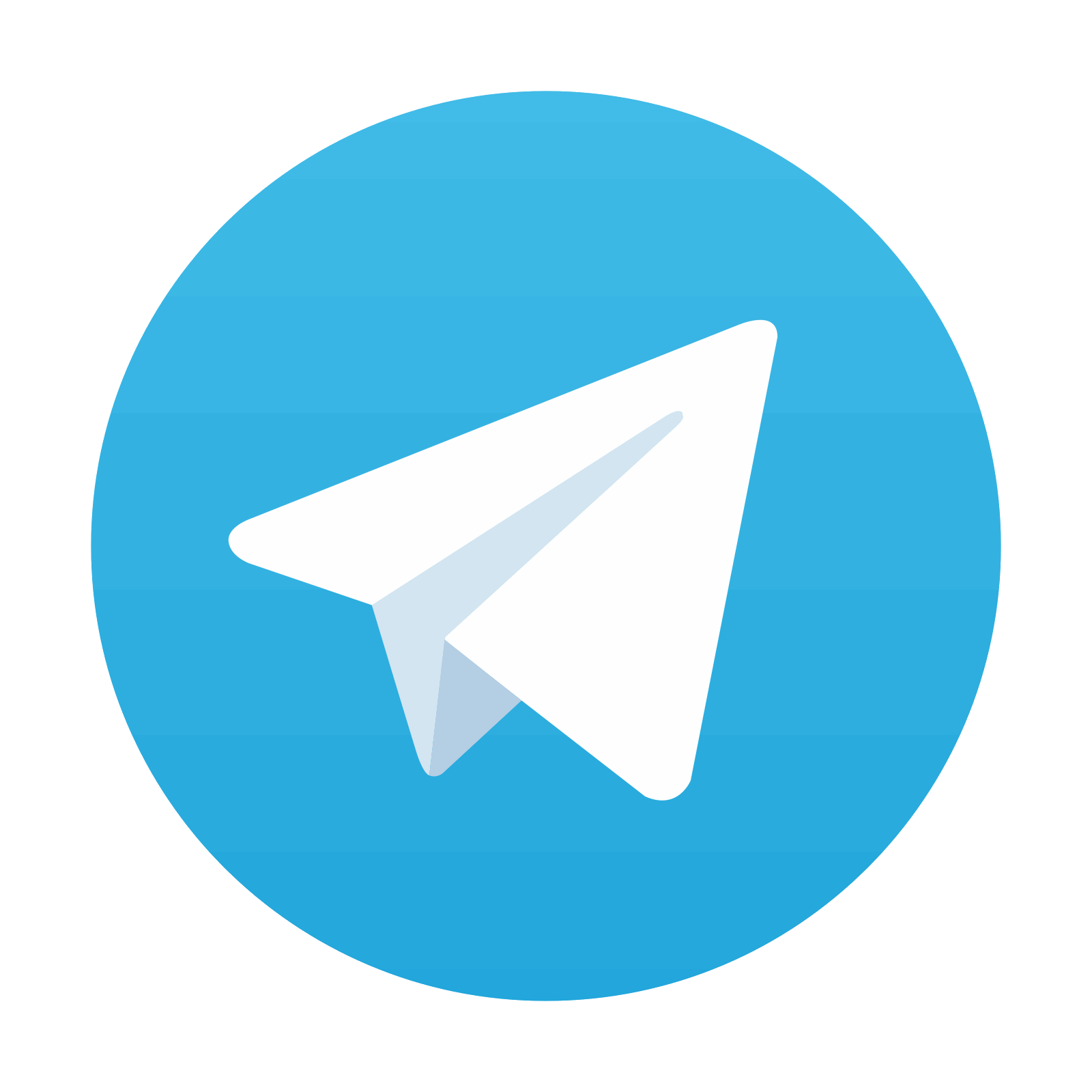
Stay updated, free articles. Join our Telegram channel

Full access? Get Clinical Tree
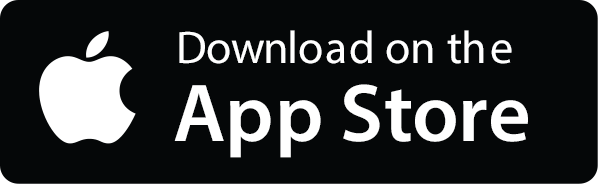
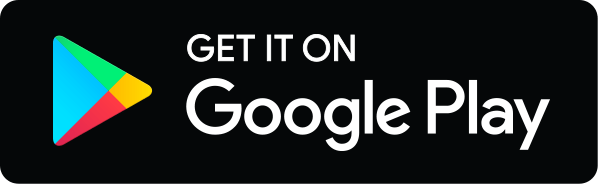