21 Ventral Subaxial Spine Constructs
21.1 History
Historically, attempts at the clinical application of ventral spinal instrumentation met with varying degrees of success. 1 , 2 Each of these devices was useful in its day and helped move the art of spine fixation forward. One of the first attempts at ventral spinal instrumentation was made by Milgram in 1953; it was unsuccessful. 3 This was soon followed by Cloward’s report: “The Anterior Approach for Removal of Ruptured Cervical Discs.” 4 Then, Humphries and colleagues, in 1961, reported the unsuccessful use of a ventral interbody fusion clamp. 5
Dwyer was among the first to insert an instrumentation construct surgically in the ventral thoracic and lumbar spine. His technique was used to instrument multiple spinal levels 6 – 8 but often met with less than optimal success (Fig. 21.1). The Zielke technique, developed subsequently, was designed to fix fewer spinal segments effectively in a more rigid fashion than could be achieved with the Dwyer technique. 9 Harrington then reported on the combination of Knodt rods or Harrington distraction rods with acrylic augmentation for ventral spinal use. 10 This was followed by the development of the Kostuik-Harrington distraction system. 3

Kaneda and colleagues developed a technique of attaching spiked vertebral plates to vertebral bodies with screws interconnected by rigid rods. 11 , 12 The Kaneda device ushered in the era of modern ventral thoracic and lumbar fixation via its ability to fix the spine rigidly over a short segment, with relative ease of insertion. A wide variety of techniques have subsequently been employed clinically. 3 , 13 – 18
The Rezaian device was developed for interbody distraction fixation (see Chapter 23). 19 It provided ventral spinal distraction, as did the Kaneda, Kostuik, and related devices. The Rezaian device provided simple distraction only, whereas the Kaneda device offered the option of applying compression or distraction and a method of applying cantilever beam fixation forces to the spine via a ventral approach.
Only relatively recently have ventral instrumentation constructs been routinely applied to the cervical region. Caspar et al developed a semiconstrained (semirigid or dynamic) ventral cervical plate system that was preferably used with screw purchase of the dorsal vertebral body cortex (bicortical purchase). 20 Morcher subsequently developed a constrained (rigid) plate system that used a screw–plate locking mechanism. 21 The latter did not require bicortical purchase, which opened the door for a wide variety of rigid, and subsequently dynamic, devices that are in use today.
21.2 Surgical Exposures
Ventral approaches to the spine for the application of instrumentation are the same as those used routinely for ventral spinal surgery (see Chapter 10). In the cervical region, if extensive longitudinal exposure is required, a diagonal rather than a horizontal skin crease incision helps to increase the length of exposure. Ventral exposure for the cervicothoracic region may be gained through the thoracic inlet, with a manubrium-splitting or a sternum-splitting approach, or with the lateral extracavitary or transcavitary approach. In the midthoracic region, the ventral spine may be accessed via the ventral transthoracic approach, the extralateral thoracotomy approach, or the lateral extracavitary approach.
The thoracolumbar region presents significant anatomical barriers because of the confines of the diaphragm and associated structures. Nevertheless, with appropriate dissection and an appropriate consideration of anatomy, this region can be exposed ventrally. It can also be accessed with the lateral extracavitary approach. From L2 caudally, the ventral lumbar spine can be accessed through the ventrolateral or lateral extracavitary approach, or even the transperitoneal approach. The lumbosacral region can be accessed by means of the transperitoneal approach for low (sacral) lesions, the Pfannensteil extraperitoneal approach, the ventrolateral extraperitoneal approach, or the lateral extracavitary approach. The latter requires substantial iliac crest resection to gain access to the sacrum.
21.3 Implant Types
Spinal instrumentation constructs are discussed here from a biomechanical viewpoint. Although much information is provided about clinically used spinal implants, this information is most certainly not complete, nor is it intended to be so. It is designed to place the final touches on a clinically practical understanding of spinal biomechanics as applied to spinal instrumentation.
Specifically, ventral distraction, ventral compression (tension-band), and ventral cantilever beam fixation strategies are discussed in this chapter. The nuances of each are emphasized. However, it is essential to understand that implants respond differently under different loading conditions. For example, a ventral cantilever beam fixation device is usually thought of as a cantilever (Fig. 21.2a). Under axial loading conditions, it resists compression and therefore functions as a distraction device (Fig. 21.2b). However, if an extension moment is applied to the spine, it resists segmental extension, thus functioning as a compression (tension-band fixation) device (Fig. 21.2c). Three-point bending forces can be applied or resisted by a multisegmental implant (Fig. 21.2d). This mode of load resistance very effectively resists translation () (see Chapter 29 and Fig. 21.2e).

This part of the chapter comprises three sections. Each focuses on the mechanisms of force application or resistance via the implant. Admittedly, the organization is somewhat arbitrary and artificial, in part because ventral distraction fixation, compression (tension-band) fixation, and cantilever beam fixation in many regards have overlapping physical and mechanical characteristics. Ventral distraction and compression devices are often cantilever beam constructs, as well. In this chapter, the biomechanical and clinical discussion is found principally in the cantilever beam section. Ventral distraction and compression fixation are discussed only when a consideration of the application of ventral distraction or compression forces is relevant—from either a clinical or a biomechanical perspective.
21.3.1 Ventral Distraction Fixation
Biomechanics
Ventral spinal distraction, for the purposes of the discussion here, is achieved with the placement of either distraction forces or a neutral construct at the time of surgery. With the placement of an implant in a neutral mode, the construct subsequently bears an axial load when the patient assumes upright posture. This essentially results in the implant resisting compression and, hence, effectively distracting the spine by applying a resistance to compression (see Chapter 17). Therefore, for the purposes of most discussions (including the discussion presented herein), the term ventral distraction fixation applies to distraction and neutral fixation unless otherwise specified.
Ventral spinal distraction is similar in many respects to the application of dorsal force by tension-band fixation (compression), but opposite in orientation. The application of distraction forces to the spine does not always result in the same type of force application. The characterization of the force application is determined by the location of the instantaneous axis of rotation (IAR) in relation to the points of instrumentation-related force application. If the point of application of a distraction force by an implant is ventral to the IAR, forces opposite in orientation, but similar in nature, to dorsal tension-band fixation are applied (Fig. 21.3a). These are usually applied via cantilever beam constructs (see Chapter 17).

If the point of application of the force by the construct is in line with the IAR, the aforementioned distraction and tension-band like forces (bending moments) are not applied to the spine (Fig. 21.3b). Such constructs are simple distraction (buttress) constructs (see Chapter 17). Tension-band forces necessitate the use of an applied moment arm to achieve their desired result. The length of this moment arm is the perpendicular distance from the point of application of the forces (by the construct) to the IAR. If this type of force is applied in the opposite direction (i.e., in a distraction mode), either distraction with extension (Fig. 21.3c) or a three-point bending type of fixation is achieved (Fig. 21.3d). The former can be thought of as tension-band distraction fixation, or rather distraction fixation applied at a perpendicular distance from the IAR (see Chapter 17).
For ventral interbody distraction (or neutral) fixation to be effective as a technique, intrinsic or surgically created resistance to distraction and/or spinal bracing is required. For example, ligamentous resistance to distraction (tensioning) causes an intrinsic compression of the bone graft into both ends of the mortise, thus providing greater security to the strut graft–mortise relationship. This can be applied by cantilever beam implants, as well.
Interbody implants that exert mechanical influence in line with the IAR cause, as mentioned, pure distraction. A variety of constructs have been employed. Expandable cages are modern-day versions of such interbody constructs. Expandable cages have been compared mechanically with nonexpandable cages and bone graft struts. None showed superiority over any of the others from a mechanical perspective, 22 likely because of the limited extension and rotation stiffness related to all implants studied. Expandable cages, however, are expensive, have less capacity for bone graft volume, provide suboptimal end plate contact for bone fusion purposes, and are associated with greater technical challenges than their nonexpandable counterparts. Titanium mesh cages for cervical spine stabilization after corpectomy have been shown to be associated with significant clinical utility. 23 Greene et al demonstrated that interbody cages mechanically perform equivalently to structural bone grafts. 24 Finally, expense of operation, as well as follow-up costs, should play at least some role in the pre- and postoperative decision-making process. Some strategies and technologies are much more expensive than reasonable alternatives. 25 , 26
Distraction, as applied by a semiconstrained (semirigid or dynamic) screw–plate device, requires that adequate dorsal spinal stability be present. A screw–plate construct, regardless of the mode of application, is effective in resisting axial loads and spinal extension, but it is not as effective in resisting flexion. In this situation, plate bending or fracture or screw cutout may occur following flexion. If dorsal spinal stability is present or created, these complications are less likely (Fig. 21.4).

Techniques
The two fundamental types of ventral distraction implants are (1) interbody struts that buttress the spine(see Chapter 23 and Fig. 21.5), and (2) cantilever beam constructs that use screws in either a fixed moment arm or a nonfixed moment arm mode (Fig. 21.6). Bone, acrylic 27 (see Chapter 23), or metal and nonmetal equivalent implants can be used for the first type. They can be inserted through a true ventral or lateral approach, as well as via the transpedicular approach dorsally. 28 Cantilever beam constructs can be applied by a variety of screw–rod or screw–plate systems.


One must appreciate the often unexpected, but usually predictable, consequences of force applications to the spine. For example, distraction with a nonfixed moment arm cantilever beam implant, without an intervening point of fixation, can result in untoward bending moment application (see Fig. 21.6).
Clinical Applications
The decision to use an interbody buttress or a cantilever beam technique with a fixed moment arm, a nonfixed moment arm, or an applied moment arm, or as a dynamic implant, is of critical importance. All too often, instrumentation techniques are chosen and applied in a somewhat cavalier manner, with too little attention paid to biomechanical principles. Questions that should be asked before the insertion of an implant include, but are not limited to, the following:
Is a spinal implant indicated, and if so, what is the specific indication for surgery?
Is a rigid or dynamic implant desired or required?
Is deformity reduction, or simply deformity prevention, required?
Is subsidence a major issue?
What are the loading conditions that will be applied to the implant and construct during routine patient activities?
These questions are not always easily answered, nor are they readily addressed. Their routine preoperative consideration ensures that the surgeon is at least placing physical and biomechanical principles in a position of high priority—that is, they are high on the list of preoperative considerations. The aforementioned questions can perhaps be better addressed by methodically considering the involved mechanics portrayed in this and subsequent chapters. In this regard, each region of the spine is associated with unique anatomical and biomechanical challenges. Hence, each region is considered separately in the sections that follow.
Cervical Spine
In the cervical spine, interbody strut buttressing and fixed or nonfixed moment arm cantilever beam fixation techniques are frequently used together (e.g., the simultaneous use of a cantilever beam construct with an interbody bone graft or cage). 29 Preoperatively, the surgeon must consider the technique that he or she deems the most appropriate for the situation at hand, using intuition, experience, and the best available evidence (i.e., existing clinical and biomechanical information). 30
Several questions related to the overall decision-making process must be specifically answered during implant selection:
The actual indication for surgery itself must be addressed. An assessment of the available evidence regarding the results of surgery versus those of nonoperative management is critical. Such evidence may be sparse in many instances but is most robust in the trauma arena. 31
Is posterior column integrity adequate? If not, it should be rectified.
Is a buttress strategy alone adequate? Anterior cervical discectomy and fusion, for example, can be performed with or without instrumentation. The insertion of an implant may or may not be associated with a significant increase in the fusion rate, but it is most certainly is associated with an improved maintenance of segmental lordotic posture and disc interspace height. 32
If greater stability is required from the implant, is a rigid construct, rather than a more dynamic construct, more appropriate (see Chapter 29, Fig. 21.6, and Fig. 21.7a)?
Fig. 21.7 As an isolated spinal stabilizer, a rigid construct (e.g., a fixed moment arm cantilever beam construct) provides greater stability than a semirigid one (e.g., a nonfixed moment arm cantilever beam construct). The nonfixed moment arm construct allows movement that a rigid construct does not (see Fig. 21.6). However, the nonfixed moment arm construct, if applied appropriately with an accompanying interbody buttress (e.g., an interbody bone graft, strut, or cage) may be effective. This is especially so if the spine is distracted, the buttress is inserted securely into its interbody position, and the construct is then compressed (see Chapter 18). (A) If the spine with such a construct is extended, (B) the bone graft is loaded in compression (arrows). (C) If it is flexed, the bone graft is unloaded (arrows). This is related to the fact that the instantaneous axis of rotation (IAR; dot) is located in or about the implant in such constructs. Therefore, angular motion rotates about the IAR.
Several nuances must additionally be considered. For example, nonfixed moment arm cantilever beam implants transfer a greater percentage of an axially applied load through the spine than do their fixed moment arm counterparts. 33 This is related to their relatively diminished stiffness 34 – 36 and to implant design characteristics, as well. 37 This must be considered in the selection of a rigid (fixed moment arm cantilever beam) versus a more dynamic (nonfixed moment arm cantilever beam) implant. Additionally, ventral plates cause unloading of an interbody strut in flexion and loading in extension. 38 – 40 This is related to the fact that the IAR moves toward the implant, thus resulting in the aforementioned phenomenon (Fig. 21.7b, c), and may be reduced by using dynamic implants or buttress plates (see Chapter 29).
Clearly, regardless of the complications associated with instrumentation, its advantages usually outweigh its disadvantages for ventral decompressive operations. 24 Interbody constructs also provide unique advantages. That said, the type of construct may have an effect on outcomes. For example, outcomes of strut grafting following corpectomy have been shown to be superior to outcomes of multiple-level discectomy and fusion procedures. 41 , 42 It is emphasized, though, that such observations were made in uninstrumented cases (no plate). However, it has also been observed that the performance of interbody strut procedures without instrumentation results in significant deformation. 43 In this regard, Bolesta et al demonstrated that ventral plate fixation improved outcome in two-level procedures. 44 Wang et al, on the other hand, did not. 45 To further confuse the issue, Park et al demonstrated that multilevel discectomy and corpectomy are relatively equivalent, although corpectomy is associated with greater subsidence. 46 Finally, disrupting the integrity of the vertebral body adjacent to an interbody cage does not appear to affect stability. Taking local autograft from the cervical vertebral body (to pack interbody cages) does not seem to be associated with significant adverse biomechanical consequences. 47 These findings are indeed confusing and difficult to interpret. Hence, the literature does not provide the guidance we might want. We therefore must rely on intuition, experience, and a solid foundation in biomechanics during the strategy-planning process.
Because the implants discussed in this chapter are often used to apply distraction at the time of surgery via screws attached to longitudinal members, or are subsequently expected to withstand additional axial loads during assumption of the upright posture, they are considered distraction implants. These are cantilever beam implants (screws affixed to a plate or rod, as a beam is affixed to an immobile object at one end only). Because cantilever beam implants resist more forces or loads than simple compression (they resist rotation, angulation, and extension forces, as well), they provide much more utility and complexity than a simple interbody strut distraction device does. An interbody device is not a cantilever beam implant. It resists rotation and translation poorly. The addition of a plate to an interbody construct adds multiplanar control of complex loads and forces. Hence, it makes significant clinical and biomechanical sense, 24 , 48 – 50 particularly in smokers. 51
Hybrid interbody constructs (both corpectomy and discectomy with interbody fusions in the same patient), combined with a cantilever beam construct (i.e., a ventral multilevel fixation plate), have shown significant efficacy for multilevel cervical myelopathy. 49 Other modifications have, as well. 52 The intermediate point of screw fixation facilitates the application and/or resistance of three-point bending forces. This then creates a construct that is even better at resisting multiplanar forces and loads. The bottom line? The more modes of force application employed in a construct (e.g., distraction, tension-band fixation, cantilever beam fixation, and three-point bending fixation), the more efficacious the construct from a mechanical perspective (Fig. 21.8). A relative exception may exist with the application of titanium mesh cages. They have been shown to be very efficacious following corpectomy. The irregular nature of the surface of the titanium cage, in contact with the end plate, provides very secure fixation. Fibula graft, however, provides a cost-effective and efficacious alternative. 53

Ventral cervical plate fixation for degenerative disease is now commonplace. Its addition to one- and two-level discectomies has been shown to decrease reoperation rates and increase fusion rates. 54 – 64 Plating may be used for revision surgery, as well. 29 , 65 It may even be cost-effective with first-time surgery. 66 Plating has been shown to reduce the incidence and extent of postoperative deformity, 62 , 67 although graft subsidence and deformity rates are higher with bioabsorbable implants. 68 Of final note, the efficacy of fixed moment arm plate cantilever beam fixation is reduced by repetitive loading (fatigue testing). This may lead to failure, particularly in junctional regions. 69 These laboratory and clinical observations underscore both the importance and the potentially misleading nature of biomechanical studies. Static testing may show substantial stability, whereas fatigue testing demonstrates construct design flaws that become evident after the application of multiple loading cycles and are also more relevant to the clinical situation. This point cannot be overemphasized.
One might think that precise ventral plate positioning during surgery is key to good results. Interestingly, from a clinical perspective, variations in plate position after ventral cervical spine surgery had little to do with clinical outcome. 70
All this having been said, complex three-column traumatic injuries can be treated ventrally alone with rigid fixed moment arm cantilevers. Careful patient selection is emphasized. 71
Thoracic and Lumbar Spine
Thoracic and lumbar ventral spinal distraction fixation techniques have biomechanical attributes similar to those of their cervical counterparts. However, slight differences do exist, such as the more common use of ventral rod–screw systems (rather than plates) and the need for more substantial constructs, which is related to the greater stresses applied to them (compared with those in the cervical spine).
The prototype of thoracolumbar devices was the Dunn device. 16 Others soon followed. The most notable and popular recently employed strategies involve the placement of interbody cages. Evidence of their efficacy as distraction spacers to maintain disc height and augment fusion is mounting. 72 Hence, their popularity is increasing. Transforaminal lumbar interbody fusion (TLIF) and other minimally invasive techniques are the prototypical strategies that employ interbody spacers. These usually incorporate interbody struts or cages with cantilever beam fixation. Such techniques have been shown to restore disc interspace height and reduce preoperative subluxations. 73 Threaded interbody fusion cages, which present their round face to the end plate, have been shown to provide suboptimal resistance to subsidence (see Chapter 23). Tapered cages may provide some advantage because of the uniformity of the contact surfaces and the preservation of lordosis. 74 Implant characteristics, such as stiffness, strength, and torsional resistance, can also affect clinical utility. 75 – 79 In addition, these implants generally stress neighboring nonfused segments to the extremes of their functional ranges. 80
As with cervical constructs, the augmentation of anterior interbody fusions in the lumbar spine with a ventral plate is associated with improved mechanics. In fact, doing so may obviate the need for dorsal pedicle fixation procedures. 81
Vertebral reconstruction via vertebroplasty may provide ventral axial load-bearing support for osteoporotic vertebral compression fracture. This has been shown to be effective with alternative materials, including biodegradable calcium phosphate cement with instrumentation. 82 The beneficial effects of vertebroplasty have been studied in the biomechanics laboratory. 83 This affects its ability to provide axial load bearing.
Finally, indirect foraminal decompression is achievable via ventral interbody approaches. This can be accomplished with a variety of techniques, including the lateral trans-psoas interbody fusion. 84
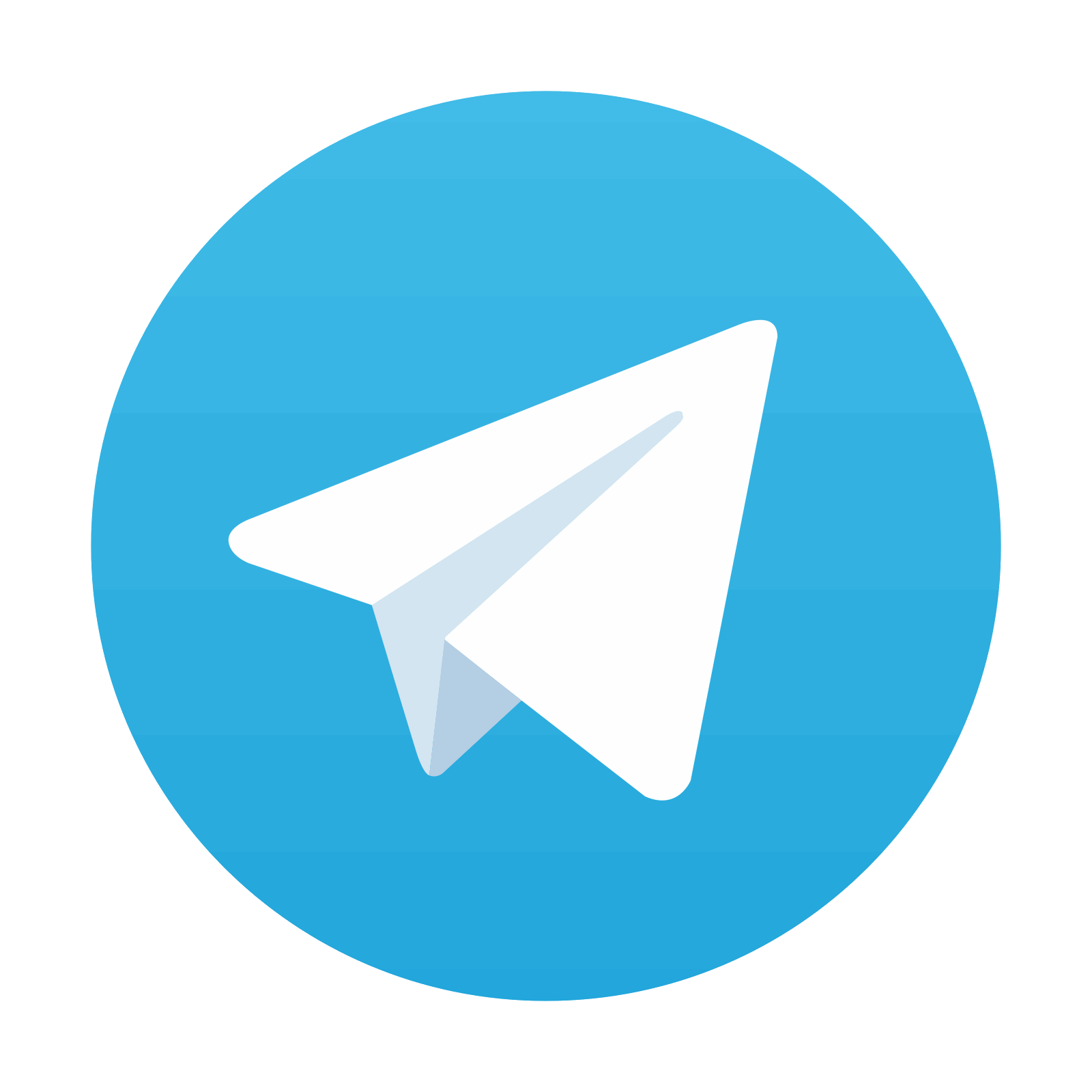
Stay updated, free articles. Join our Telegram channel

Full access? Get Clinical Tree
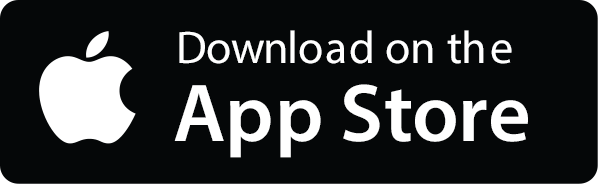
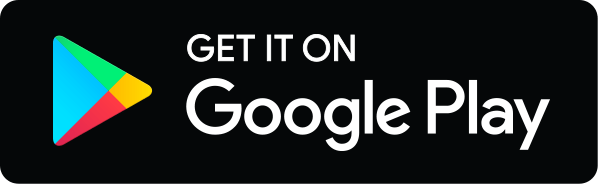
