22 Dorsal Subaxial Spine Constructs
22.1 History
Spinal implants were initially, and are still, used for the supplementation of bony fusion. However, bony fusion operations were initially performed without implants. 1 , 2 Wire and screw fixation of the unstable spine was first reported around the turn of the century. In the United States, these techniques remained quietly in vogue until the pre-World War II years. 3 – 6 During the same period in Europe, Fritz Lange described the use of steel rods for the stabilization of the spine. 7 In this ill-conceived but innovative approach to spine stabilization, the rods have no solid bony purchase. Success was therefore limited.
In the 20 years after World War II, there were two major breakthroughs in spine surgery: the interspinous wiring technique of Rogers and the introduction of the Harrington system for spine stabilization and deformity correction. Rogers described the technique of cervical interspinous wiring in the early 1940s. 8 , 9 Harrington introduced his instrumentation system in 1962. 10 , 11 For illustrative purposes, some of the dorsal spinal implants that are of historical interest, but for the most part are no longer in use today, are depicted in Fig. 22.1.

Since then, modifications of both the Rogers technique and the Harrington system have been devised to increase their security of fixation. These include a variety of interspinous wiring modifications and the use of sleeves and square-ended modifications of Harrington rods. The next significant advance in dorsal spinal stabilization was the development of multisegmental spinal instrumentation. Multisegmental instrumentation permits sharing of the load applied to the instrumentation construct with multiple vertebrae, thus substantially decreasing the chance of failure at the metal–bone interface.
The Luque segmental wiring technique, developed in the early 1970s, was the first of this class of implants to achieve wide clinical application. 12 , 13 Subsequent modifications have been used. These include closed loops instead of rods and techniques for anchoring the rods to the sacrum (see Fig. 22.1). 14 , 15 Deformity correction is achieved by sequential tightening of the wires. 16

Sublaminar wires were used to augment the efficacy of Harrington rod fixation by reducing the chance of hook dislodgement. 17 This combination allowed the surgeon to apply distraction and simultaneously enhance the correction of the spinal deformity. Further modifications were the forerunners of more complex, currently used systems of universal spinal instrumentation (USI). 3 , 14 , 15 , 17 – 19
Harrington was the first to report the use of the pedicle as a fixation site. He abandoned this concept because of problems with component–component (screw–longitudinal member) integrity. Roy-Camille et al were principally responsible for the refinement and institution of the common clinical application of pedicle fixation. 20
The Luque sublaminar wiring technique waned in popularity, primarily because of the associated risk for neurologic injury and the lack of ability to exert distractive or compressive forces on the spine. Cotrel and Dubousset developed an instrumentation system that addressed these issues and more. 21 , 22 The Cotrel-Dubousset instrumentation system consists of rods and multiple hooks (which can be affixed to the lamina, pedicle, or transverse process) and screws—a true USI. This provides a reliable segmental fixation of the spine, plus the option to use dorsal rotational forces to correct scoliotic deformities. These and other advantages allow the safe and efficacious segmental fixation of the spine and the introduction of several additional manufacturer-specific, but similar, implant systems. 19 , 23 – 27
Roy-Camille pioneered the development of lateral mass plates and screws; these were introduced in the United States in 1988. 28 They have overtaken cervical wiring techniques in popularity with surgeons.
Dorsal distraction, dorsal compression (tension-band fixation), dorsal three-point bending, and dorsal cantilever beam fixation are individually addressed in this chapter. Like ventral fixation strategies, dorsal implants respond differently to different loading conditions. This must be repetitively considered by the spine surgeon. Of general note regarding dorsal spine surgery is its tendency to destroy soft tissue. Kawaguchi and colleagues demonstrated muscle injury in all patients who underwent dorsal lumbar surgery. These injuries were related to retraction pressure and to the duration and extent of exposure. 29
22.2 Dorsal Distraction Fixation
The use of a spinal implant to apply a dorsal distraction force alone is uncommon. Usually, there is an accompanying three-point bending or cantilever beam force application. The rarity of the need for an isolated implant-derived dorsal distraction force application and the possibility of exaggerating spinal deformation tend to discourage the clinical use of such an implant-derived force application.
22.2.1 Techniques and Clinical Applications
With respect to isolated dorsal distraction force application, short-segment applications are common. The use of the now extinct Knodt rod (via sublaminar hooks) at a single motion segment is an example of such an application (Fig. 22.2a, b). Interspinous process distraction–decompression systems apply the same forces to the spine, only more dorsally (Fig. 22.2c). Significant complications can arise from the use of such a fixation strategy, 30 despite studies that demonstrate otherwise. 31 , 32 Nevertheless, the desired result remains the opening of the neuroforamina. Spacers combined with spinous process fixators have also been studied. 33 Such a strategy has been employed in the cervical spine to treat cervical spondylotic radiculopathy and myelopathy. 34
Dorsal spinal distraction for the reduction of an extension spinal deformity may be indicated, but rarely (Fig. 22.3). Distraction, combined with three-point bending force application, may be useful. 35 Such a strategy is termed ligamentotaxis or annulotaxis (see Chapter 10 and Fig. 10.5).

22.2.2 Complications
The application of isolated dorsal spinal distraction forces may exaggerate a kyphotic deformity. This most often occurs when a segmental kyphotic deformity is superimposed on a normal lordotic curvature. The moment arm through which the distraction forces act, and the inability to achieve a three-point bending force application, set the stage for this phenomenon. The latter results from the lack of contact between the rod and the fulcrum (Fig. 22.4).

This type of force application may flatten the normal lordotic curvature (Fig. 22.5). This is occasionally associated with a clinical–anatomical syndrome of back pain termed flat-back syndrome. Patients with this syndrome have a loss of lumbar lordosis and a thoracic compensatory attempt at restoring sagittal balance (see Chapter 27, (Fig. 22.6, and Fig. 22.7). Because of pelvic flexion, the person so afflicted must flex the knees to “face forward.” This results in significant back, buttock, and dorsal thigh tightness and pain. In refractory cases, wedge osteotomies may be required to correct the deformity (see Chapter 27).



Not surprisingly, screws and hooks were ultimately shown to fare better than sublaminar cables in biomechanical comparisons regarding pullout and other forms of failure performed in vitro. 36 Except in the very osteoporotic spine, screws appear to fare better than simple pedicle hooks from a pullout perspective. 37 On the other hand, pedicle and laminar hook claws fared better than pedicle screws in another study. 38 This suggests that hook claws provide substantially better fixation than simple pedicle hooks. In this domain of selection of implant type in the osteoporotic patient, clinical judgment must prevail. Although not absolutely clear, hooks placed in a claw configuration appear to provide the greatest fixation potential regarding pullout resistance in osteoporotic bone.
22.3 Dorsal Compression (Tension-Band) Fixation
Short tension-band constructs understandably result in less spinal stiffness than longer constructs. Therefore, a shorter tension-band fixation construct, appropriately used, carries a lesser chance of early and late implant–bone interface failure.
Dorsal tension-band fixation forces rely for success on the principle of “closing the fish mouth” (Fig. 22.8). If the “jaw” of a “fish” is intact, then simply forcing the fish’s “mouth” into a closed position will result in a tight approximation of the teeth (and a solid construct). On the other hand, if the fish’s jaw is fractured, closing the fish’s mouth will not ensure approximation of the upper and lower teeth (Fig. 22.9a); in fact, it may further disrupt their alignment (Fig. 22.9b). The clinical correlate of the “fractured jaw” is disruption of the lamina or spinous process (see Fig. 22.9). Closing the fish’s mouth with a tension-band fixation construct may exaggerate the deformity and do little to augment spinal stability.


Tension-band fixation constructs function by closing the fish’s mouth. To provide spinal stability, the force application requires an intact hinge serving as the instantaneous axis of rotation (IAR). Without this intact hinge, success cannot be expected.
Another relative requirement of the anatomical or pathoanatomical arrangement of the spine, before the application of dorsal tension-band fixation forces, is the intrinsic or surgically created ability of the spine to resist overcompression. If, for example, the fish had no teeth, but the hinge of the jaw was intact, forceful closing of the mouth would result in an “overclosure” of the jaw. In the case of the spine, a similar mechanism could result in nerve root impingement at the level of the neuroforamina or in buckling of the ligamentum flavum into the spinal canal (Fig. 22.10).

If, on the other hand, the fish’s tongue (our hypothetical fish has a tongue) were swollen and protruded into the mouth, the application of a tension-band fixation force would result in further protrusion of the tongue, and reduction of its volume before jaw closure (implant placement) would be optimal (Fig. 22.11).
It has been emphasized in Chapter 12 that an adequate ventral neural element decompression must be performed before the application of a tension-band fixation implant to the spine. Obviously, closing the fish’s mouth will wedge any mass that is dorsal to the hinge (IAR) toward the dural sac (see Fig. 22.10 and Fig. 22.11). This phenomenon is a result of the redirection of the transmitted forces. Remember, a tension-band fixation technique only closes the fish’s mouth. It does not stabilize the hinge of the jaw (Fig. 22.12). This stability must already be present.


The application of dorsal compression forces (i.e., by a tension-band construct) with an accompanying interbody fusion increases the bone healing–enhancing forces if the interbody fusion is positioned dorsal to the IAR. The underlying principle is that of “load sharing.” Placement of the construct in a compression mode in this manner allows the axial load-supporting capacity of the ventral interbody fusion to be maximally exploited by compression of the interbody strut into the mortise of the vertebral body (Fig. 22.13). This requires that the anterior longitudinal ligament be intact.

The forces applied to the spine by tension-band fixation constructs were discussed in Chapters 16 and 17. The direction of the force applied to the spine by tension-band fixation constructs differs by 90 degrees from that of the force applied by three-point bending constructs. The former is parallel to the long axis of the spine; the latter is perpendicular to this axis (Fig. 22.14). Because of the parallel orientation of the force application and the fact that the moment arm is perpendicular to this orientation, the length of the construct does not affect the bending moment applied at the termini of the construct. Therefore, the length of the construct does not affect the efficacy of deformity correction. The only factor affecting the length of the moment arm is the distance from the IAR to the point of attachment of the construct (Fig. 22.15).


22.3.1 Techniques
The application techniques for tension-band fixation vary widely. They range from cerclage wiring in the cervical spine (true tension-band fixation) to the use of cantilever beam fixation constructs in a compression mode. 39 They all have one attribute in common: the application of a compression force complex at a point that is dorsal to the IAR and the neutral axis. From a mechanical perspective, pedicle screw anchors have been shown to be superior to hook anchors in resisting traction (i.e., by a tension-band fixation strategy). 40 In this regard, the pedicle screw is resisting loads applied perpendicular to the long axis of the screw, as opposed to the discussion regarding pullout in the prior section (“Dorsal Distraction Fixation”).
22.3.2 Clinical Applications
Cervical Spine
Dorsal tension-band fixation techniques for application in the cervical region have one significant advantage that similar applications in the thoracic and lumbar region do not: the orientation of the facet joints in the coronal plane (Fig. 22.16). If the integrity of the facet joints is left intact by the pathologic process, the dorsal application of tension between two vertebral segments at the lamina or spinous process level (dorsal to the IAR) positions the involved facets so that flexion and, more importantly, ventral translation cannot occur. The juxtaposed nature of the cervical facets results in their contribution to translation resistance by virtue of their engagement (see Fig. 22.16). These factors simplify the decision-making process regarding the application of dorsal tension-band fixation spinal implants in the cervical region.

We have focused on dorsal tension-band fixators. One must remember, though, that ventral cantilever beam constructs (Fig. 22.16i) function as distraction devices (resist compression in the upright position), cantilever beam devices (because they are, indeed, cantilever beams), and tension-band fixators (compression devices)—depending on the conditions of loading. Regarding compression force application, the ventral cantilever beam construct resists extension and, as such, functions as a resistor of extension and so a tension-band fixator (Fig. 22.16j). This was presented in Chapter 21.
Thoracic and Lumbar Spine
In the thoracic and lumbar regions, the facet joints are oriented in noncoronal planes and much greater loads are applied to the spine, under both normal and extreme loading conditions. Hence, the expectations of thoracic and lumbar spinal implants and the forces applied to the spine by the implants and by normal and excessive spinal movements are much greater than in the cervical region. Nevertheless, short-segment compression (tension-band) fixation has been shown to be beneficial, particularly in regions with a natural kyphosis (e.g., the thoracic spine). 35 The evolution of devices and techniques for thoracic and lumbar instability has culminated in the use of USI for the treatment of posttraumatic spinal instability. These techniques provide the immediate acquisition of significant spinal stability. The degree of rigidity attained is substantial. Long-rod techniques frequently provide a more-than-adequate degree of spinal stability. However, excessive stress shielding can result. This may limit healing and bony fusion. Terminal hook–bone, or even screw–bone, interface failure may be encouraged by the use of long-rod and short-fusion techniques (either following the acquisition of adequate bony fusion or with pseudarthrosis), necessitating removal of the implant. This is so because the points of contact of the implant with the spine in the unfused regions are continuously exposed to loading–unloading stresses. Repetitive motion will result in degradation of the hook–bone and screw–bone interface and failure.
22.3.3 Multisegmental Fixation
Tension-band fixation can be applied in a multisegmental manner. This can provide some advantages by distributing forces over multiple implant–bone interfaces (see Chapter 18). Terminal bending moments may result when an insufficient number of intermediate fixation points have been used (Fig. 22.17).

When interspinous wiring techniques are used for multisegmental fixation, multiple overlapping one-motion-segment cerclage wires are biomechanically optimal, particularly compared with a single cerclage wire, as might have been used in Fig. 22.18. When one long cerclage wire is used, there is a tendency toward the formation of terminal bending moments (see Fig. 22.18). This tendency is minimized by using the multiple overlapping cerclage wiring technique (see Fig. 22.18). Fortunately, far superior techniques for attaining the same result are available today.

22.3.4 Complications
Marked spinal deformities cannot be consistently reduced by dorsal tension-band fixation techniques alone. Similarly, patients who have incurred a substantial loss of lateral translational stability or scoliotic curvature are poorly served by these techniques. Intervertebral ligamentous support, which is diminished after significant translational injuries, is often necessary for the success of tension-band fixation techniques.
Similarly, dorsal tension-band fixation techniques have a limited ability to apply an effective moment arm for either the reduction of a scoliotic or kyphotic spinal deformity or the prevention of the development of a translational deformity when significant translational instability is suspected. A scoliotic deformity may be exaggerated following the use of a dorsal short-segment fixation compression technique (Fig. 22.19). Wire or cable cut-through may occur with any cerclage technique. A variety of interspinous tension-band fixation devices have been proposed and recommended. 41 Therefore, an understanding of the capacity of the spinous process to withstand compression fixation becomes mandatory. This, in fact, is limited. 42 For these reasons, dorsal spinous process plates have been of intermittent utility over the years. 43

Finally, dorsal plates and tension-band fixation devices alter interbody loads. 44 This can have a positive or a negative effect on healing, depending on factors such as ventral stability and axial load-bearing capacity (see Chapters 27 and 29).
22.3.5 Clinical Examples
Tension-band fixation constructs are commonly applied clinically. The prototype of this construct type is the interspinous wiring technique, which, if applied to excess, can result in spine deformation, usually hyperextension (Fig. 22.20). A variety of laminar clamps can be used to apply tension-band fixation forces to the spine (Fig. 22.21). Such techniques are uncommonly used today. They have been supplanted by pedicle screw–rod fixation techniques of the fixed and nonfixed cantilever beam types.


22.4 Dorsal Three-Point Bending Fixation
Three-point bending instrumentation is usually, but not always, applied in a complex manner with accompanying distraction or compression—for example, by Harrington distraction rods or by USI techniques applied in a distraction or compression mode. Three-point bending implants may also be applied in a neutral mode by USI or segmental sublaminar wiring constructs (see Chapter 17). It is usually the preferred mode of implant-derived force application for deformity prevention or treatment by most dorsal distraction techniques and many neutral techniques. Three-point bending constructs involve instrumentation application over multiple spinal segments, usually five or more, with accompanying dorsally directed forces applied at the upper and lower implant–bone interfaces and a ventrally directed force applied at the fulcrum (Fig. 22.22). This technique is often used following trauma to achieve a ventral decompression of the dural sac by distracting the posterior longitudinal ligament. The desired resultant force application may “push” the offending retropulsed bone and/or disc fragments ventrally and away from the dural sac. This is termed ligamentotaxis or annulotaxis. Because of the relative weakness of the posterior longitudinal ligament and/or the fixed nature of the retropulsed fragments, this technique may not always succeed (see Chapter 8). Sleeves around the rods that function as spacers may be used to increase spinal extension by moving the contact of the implant with the native spine to a more ventral position, thus enhancing the desired force application (Fig. 22.23).


The application of a dorsal distraction force that spans more than one motion segment nearly always applies a three-point bending force complex to the spine. Even if a three-point bending construct is not initially planned, the application of sufficient distraction will eventually result in enough spinal flexion that the construct makes contact with the spine at the level of the spinal deformity (i.e., at an intermediate point along the construct termed the fulcrum) with hook–rod fixation systems. With screw–rod fixation systems and intermediate screws, the resistance to three-point bending loads and the application of frank three-point bending forces to the spine are assumed. The addition of intermediate screws significantly increases the stiffness of a short-segment pedicle fixation system. 45 This is so because of the simple addition of another point of fixation, the elimination of the ability of the intermediate vertebra to “snake,” and the ability to apply and resist three-point bending forces. Before engagement of the fulcrum, flexion occurs because of the application of the distraction force at points dorsal to the IAR. This is most common in the lumbar region, where a “natural” lordotic posture is present (Fig. 22.24).

22.4.1 Dorsal Facet Fixation
Although facet fixation is not strictly speaking a three-point bending construct, shear forces are indeed applied to the screws, and therefore three-point bending forces are resisted/absorbed by the screw. As such, dorsal single-level three-point bending forces can be absorbed by screws that pass through two bony components. This is illustrated by the translaminar transfacet technique of Magerl (Fig. 22.25a–c). In the thoracic spine, transfacet fixation results in a slightly different force application—with shear forces applied. With this technique, three-point bending forces are resisted by the screw during loading (flexion or extension). 46 Via closed techniques, the accurate placement of such screws is precarious. Adjuncts to insertion, such as guide devices, have been developed to facilitate safe insertion. 47

A similar technique in the cervical spine has been found to be suboptimally effective from a biomechanical perspective. 48 , 49 This technique, the transfacet screw fixation technique, differs from its thoracic counterpart in that it does not involve a pedicle purchase component (Fig. 22.25d). This is akin to the difference between the Magerl and Boucher techniques applied in the lumbar spine. 50 , 51 The Magerl translaminar fixation technique involves passage of the screw through the lamina, through the facet joint, and into the pedicle (see Fig. 22.25 a–c). Three-point bending (shear) forces are applied to the screw during loading, as in the thoracic transfacet pedicle screw fixation technique. The Boucher transfacet fixation technique involves passage of the screw in a transfacet manner, with the screw terminating in the pedicle (Fig. 22.25e, f). In this way (i.e., screw termination in the pedicle), the lumbar transfacet technique provides substantially greater mechanical strength than does the cervical true transfacet counterpart. Both lumbar techniques provide substantial stability. The stability provided is, from a biomechanical perspective, equivalent to or comparable with that provided by pedicle screw fixation in many of the parameters studied. 52 – 55 Regarding the transfacet fixation technique of Boucher, which indeed is arguably more biomechanically sound than the translaminar transfacet technique of Magerl, the forced engagement of the facet joints by the screws facilitates the security of fixation by taking advantage of the substantial surface area of close contact between the facet joint surfaces (Fig. 22.25g). This most likely explains its biomechanical superiority. 50 – 55 Of note, Tuli et al demonstrated an increased risk for reoperation in a clinical comparison of translaminar facet screw fixation and pedicle screw fixation. 56 This has not been studied with the transfacet technique of Boucher.
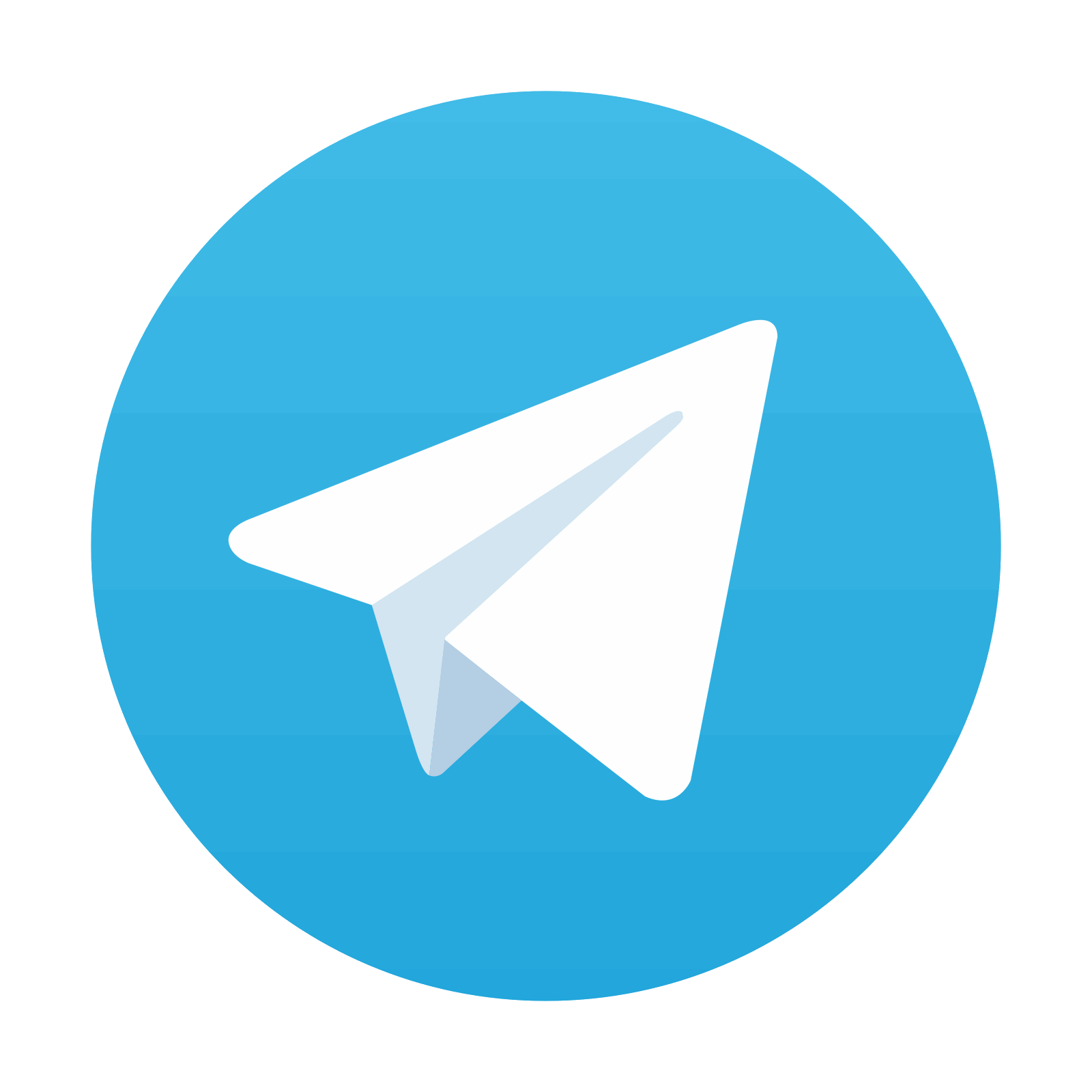
Stay updated, free articles. Join our Telegram channel

Full access? Get Clinical Tree
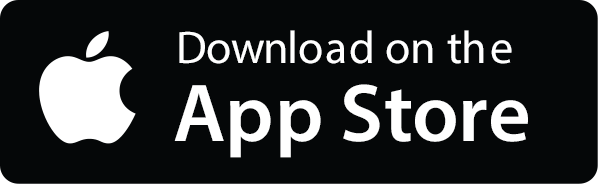
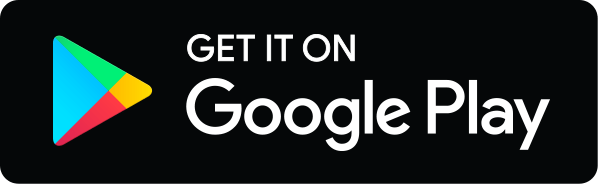
