23 Posterior Quadrantic Resection and Disconnection
Surgery is often contemplated when patients with medical intractability suffer from widespread areas of hemispheric epileptogenicity. When the whole hemisphere is involved with accompanying hemiplegia, hemispherectomy is the treatment of choice. In subhemispheric epilepsy (epilepsy involving two or more lobes), where there is residual motor neurological function (finger opposition and foot tapping) secondary to a condition that is static, multilobar surgery sparing the functional cortices is an option that needs to be considered. Intractable multilobar epilepsy has traditionally been treated by resective surgery, which involves the removal of large parts of the hemisphere, classically, either the frontal and temporal lobes, or the temporal, parietal, and occipital lobes (posterior quadrant resection), leaving behind a large operative cavity. Akin to the evolution of disconnective techniques in hemispherectomy, the surgical approach for posterior quadrantic epilepsy has evolved progressively toward more disconnection and less resection. These techniques, while maintaining similar seizure outcomes to resective surgery, are aimed at reducing perioperative morbidity and long-term complications.
Indications for Surgery in Subhemispheric Surgery
The surgical treatment of posterior quadrantic subhemi-spheric epilepsy has been increasingly used in the last decade, but the frequency of these surgeries accounts for less than 5% of all epilepsy surgeries.1,2 The increased use of these techniques has been due to advances in Neuroimaging, increased awareness, advances in pediatric anesthesia, and introduction of disconnective techniques in epilepsy surgery. Posterior quadrantic epilepsy surgery is indicated when the epileptogenic zone encompasses large areas of the temporal, parietal, and occipital lobes (posterior quadrant) and spares the central and frontal areas. The indication rests on a good concordance between the imaging (magnetic resonance imaging [MRI], computed tomography, nuclear) studies, electroencephalography (EEG), and clinical and neuropsychological evaluations that localize the lesion to the posterior quadrant unilaterally. The etiologies for posterior quadrantic epilepsy in our series were cortical dysplasia, Sturge-Weber syndrome, ischemic prenatal lesion, and sequela of ruptured arteriovenous malformations.
Preoperative Assessment
The examination of the neurological status generally confirms the presence of homonymous hemianopsia. Patients will demonstrate the ability to perform fine finger movements and foot tapping, although some may have parieto-cortical sensory loss. The preoperative investigations include MRI and prolonged EEG recordings with telemetry. MRI should reveal the presence of an extensive radiological abnormality of the temporo-parieto-occipital lobes, with imaging characteristics of the causative pathology ( Fig. 23.1 ). The interictal EEG and prolonged EEG telemetry confirm the origin of the epileptiform activity arising from the large radiological abnormality with propagation of activity to anterior cortices or contralaterally in ictal recordings. The concordance achieved with presurgical investigations (that aim at localizing the epileptogenic zone) generally obviates the need for chronic invasive recording. Invasive recording is reserved for difficult cases in which phase I presurgical evaluation results in discordant findings. The pathological substrate responsible for the seizure disorder should be static and should necessarily not be a progressive one like Rasmussen encephalitis. The presence of residual voluntary motor function of the contralateral distal musculature, that is, finger opposition and foot tapping, contraindicates a hemispherectomy but forms the indication for posterior quadrant resection or disconnection. The presence of a visual field defect imparts greater confidence to the surgeon in his or her decision to carry out this extensive surgery. However, the possibility of creation or aggravation of a homony-mous field deficit is not an absolute contraindication to this surgery in cases in which the risk–benefit ratio is favorable. In dominant posterior quadrantic surgery, speech is a significant concern. In our experience, all patients who underwent surgery for dominant quadrantic lesions had congenital lesions and consequently had language functions residing in the right hemisphere. This hypothesis was made on the basis of the seizure semiology, neuropsychological examination, and more so on the basis of the nature and location of the radiological abnormalities. This precluded the need for a Wada test. Our presurgical hypothesis was validated by the absence of postoperative language dysfunction.

Evolution of Surgery for Subhemispheric Epilepsy
We have recently described the evolution of procedures for subhemispheric epilepsy.3 Over the past 15 years, the surgical procedure has evolved from anatomical multilobar resection to the introduction of disconnective techniques similar to the development of functional hemispherectomy techniques in hemispheric epilepsy. In the early years, the surgical procedure for this disease was anatomical posterior quadrantectomy, which entails the removal of the temporal, parietal, and occipital lobes. The next step in the evolution was the description of functional posterior quadrantectomy. In this procedure, an extended temporal lobectomy is performed, then the parietooccipital lobe is disconnected. The latest progress in the surgical treatment of subhemi-spheric epilepsy has been the introduction of peri-insular posterior quadrantectomy (PIPQ). In this surgery, no lobe is resected, and the entire posterior quadrant (temporo-parieto-occipital cortex) is disconnected but remains viable because of the preservation of vessels supplying or draining the disconnected lobes.3
Anesthetic Considerations
Most patients with intractable subhemispheric epilepsy are children; some are infants.4 Anesthesia in these situations poses a formidable challenge. Some important considerations are therefore mandatory. Many of these children are often developmentally delayed and uncooperative. They may have carious or loose teeth, gum hypertrophy, enlarged adenoids or tonsils, or cardiac involvement, and all of them will be on one or more seizure medications. Chronic carba-mazepine and phenytoin usage is associated with resistance to muscle relaxants and narcotics. Posterior quadrantic surgery is a major, complex, long-duration procedure that can have massive blood loss and fluid shifts. Because the skull of a child is larger in proportion to the body than that of the adult. The scalp and skull are very vascular; massive bleeding may be expected. Therefore, venous access with at least two large bore cannulae should be assured, and meticulous monitoring of oximetry, invasive arterial pressure, central venous pressure, temperature, expired carbon dioxide and anesthetic gases, urine output (indwelling catheter), serial hematocrit, arterial blood gases, electrolytes, and coagulation parameters is required. Continuous intra-arterial blood pressure and central venous pressure monitoring is mandatory because these monitors help in optimizing volume replacement. A depth-of-anesthesia monitor is helpful to measure the level of anesthesia, prevent awareness, and prevent overdose of inhalational or narcotic agents. An ideal anesthetic regime would rapidly induce sleep without interfering with the EEG, provide analgesia when required, provide cerebral protection with a stable ICP, provide hemo-dynamic stability, and end with a rapid awakening to a safe state when the procedure is finished. The smaller the patient is and the more complex the surgery is, the more difficult it is to manage the blood loss, hemodynamics, and temperature. Isoflurane and sevoflurane with mild hyperventilation may be used. Propofol will produce a consistent reduction in cerebral blood volume and intracronial pressure (ICP). Neuroprotection is best done by ensuring adequate cerebral perfusion. The target for fluid therapy is normovolemia. Normal saline is the commonly used crystalloid, although large volumes of normal saline will cause acidosis. Colloids such as Voluven are an option. The child may tolerate low hematocrit, but, if necessary, transfusion may be started to maintain normal volume. There is also a need to check coagulation profile and get specific blood products like fresh frozen plasma (FFP) and cryoprecipitate, if required, as in cases of massive transfusion. The ambient temperature needs to be kept up with a body warmer, warm intravenous fluids, and airway heat-moisture exchangers.
Intraoperative Functional Mapping
Intraoperative electrophysiological monitoring assumes major importance in posterior quadrantic epilepsy surgery because the motor areas need to be identified and preserved, and the area of the brain that is epileptogenic needs to be defined for disconnection or resection.
Electrocorticogram (ECoG) is used mainly to delineate the extent of the epileptogenic foci or to confirm the success of the predetermined resection or disconnection. This is performed using electrodes placed on the surface of the cortex, usually in strip or grid format. The choice of electrodes will depend on the size of the craniotomy (exposed cortex). Localization of the epileptogenic foci will need grid electrodes that are either rectangle or square format; their number can vary between 4 and 64 electrodes. Dedicated intraoperative monitoring machines enable one to do either monopolar or bipolar recording through software control. In posterior quadrantic surgery, ECoG helps in identifying the limits of the epileptogenic zone. However, the practitioner must be cautious when choosing the extent of excision. Primary consideration for excision should be based on clinical factors, MRI, and EEG recordings done in the laboratory preoperatively. The ECoG would only reinforce one’s preoperative hypothesis as far as localization of the epileptogenic zone is concerned.
Functional mapping of the cortex assumes great importance in posterior quadrantic epilepsy surgery because of the risk of damaging functional cortices. Although the relationship of the epileptogenic lesion to the central sulcus (CS) can be determined with MRI, the location of the CS during surgery is difficult because of its wide variability and brain shifts. Lesions (atrophic or mass) may shift the CS thus rendering standard surface markings of the CS erroneous. The relationship of the sensorimotor cortex to the superficial venous system is, at best, inconsistent. Based on our earlier study localizing the CS in patients with perirolandic tumors, we have noted that in 13% of cases, large veins were absent in relation to the motor strip and a predominant vein was present over the CS in only 68% of the cases.5 Despite numerous technological advances in localization of functional cortex such as functional MRI, magnetic encephalography, Wada testing, surgical navigation systems, and intraoperative MRI, electrophysiological methods are still very much in use in the operation theater and in most cases considered the gold standard.
SSEP Localization of CS
The recording of somatosensory evoked potentials (SSEPs) in the operation room is relatively easy. SSEPs are obtained almost independent of anesthetic agents and are not influenced by muscle relaxants.6 The median nerve contralateral to the lesion is stimulated percutaneously at the wrist, each stimulus being a constant electric square pulse of 200 microseconds at a rate of 4.7/second. Stimulus strength is set above a moderate thumb twitch. Filters are set to 10 Hz and 1000 Hz with notch filter (50 Hz) turned off. Each recording is an average of 100 to 200 responses. Simultaneous recordings are obtained from an array of silver/silver chloride plate electrodes (SLE Ltd., South Croydon, UK). The reference electrode is placed at Fpz (International 10–20 system) and the ground electrode at Erb’s point. The electrode array is placed over the exposed cortex, and contact of the electrodes is ensured. The electrode plate is moved in different directions over the probable hand area to get the maximal response at approximately 20-millisecond latency. Studies have suggested that these 20-millisecond potentials originate from area 3b of Brodmann, which is located in the posterior wall of the CS.7 Of the 20 channel recordings, the 2 channels that show a maximum negative (N20) and a positive (P20) response are chosen. To further confirm the location of the maximum response, the grid electrodes are replaced with two individual disc electrodes. The amplitudes are measured from peak to peak N20/P25 and P20/N25. The gyrus from which the maximum N20 response is recorded is identified as the post central gyrus (primary sensory cortex), and the gyrus just anterior to it is identified as the precentral gyrus (primary motor cortex) from where the P20 response is usually obtained. We have earlier shown that even when patients have mild senso-rimotor deficits preoperatively, it is still possible to record a phase reversal, but it is usually of a smaller amplitude.8 Conversely, if patients have gross sensorimotor deficits, they failed to record a phase reversal. However, in posterior quadrantic epilepsy, this is not of much concern, because no gross motor or sensory deficits exist.
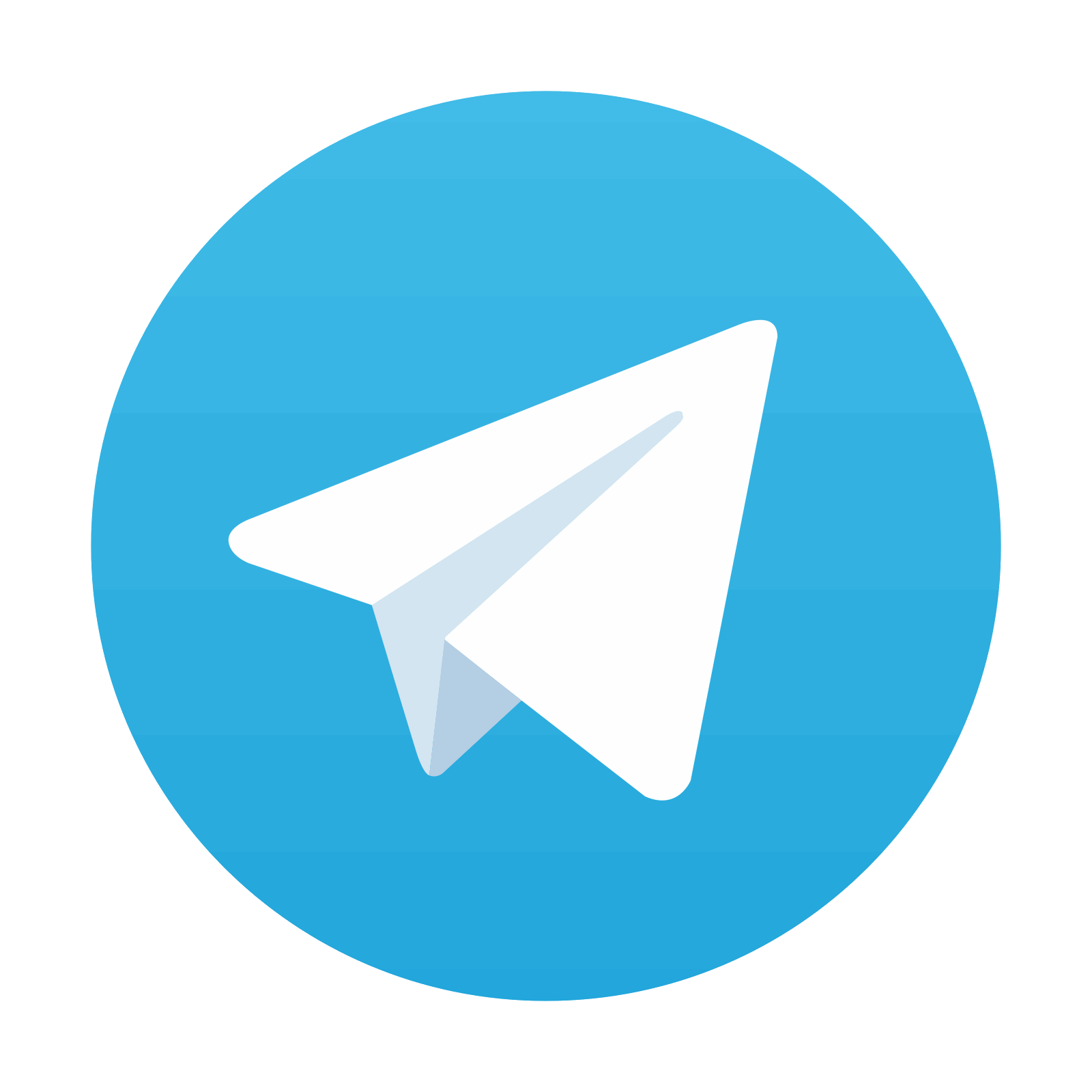
Stay updated, free articles. Join our Telegram channel

Full access? Get Clinical Tree
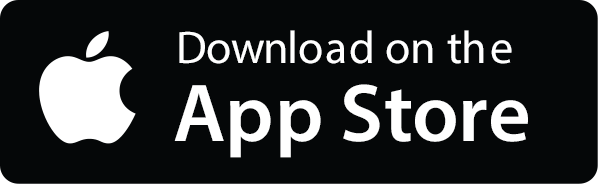
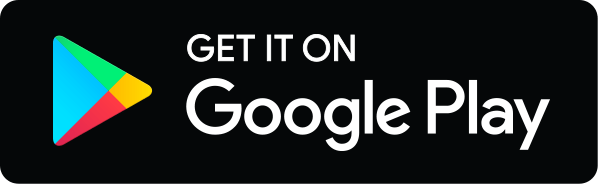
