25 Systemic Complications and Disease-Specific Phenomena Leading to Ischemic Injury
Abstract
The neurointensivist is first an intensivist, trained to treat all systems of the body in the acute care setting. In addition this clinician must understand how each system affects and is affected by the brain and spinal cord. All treatment in the neurosurgical intensive care unit should be directed at preventing ischemic central nervous system injury by optimizing the other body systems to deliver the proper amount of substrate to the brain and spinal cord. The delivery of substrate must remain consistent and within parameters necessary to overcome the current injury and to prevent further injury. Thus normal parameters of perfusion, oxygenation, metabolite delivery, and physiological function should not be kept normal but must be altered to meet the altered demands of ischemic nervous system tissue and nervous tissue that is in the penumbra of the injury. Without a thorough knowledge of the nervous system just maintaining the normal physiological conditions of all other body systems may cause irreversible neurologic damage and a poor outcome.
Case Presentation
A 69-year-old Hispanic woman undergoes craniotomy for evacuation of a traumatic subdural hematoma at a community hospital and is transferred on postoperative day 2 to a tertiary care trauma center for a higher level of care in the neurosurgical intensive care unit. At the original hospital, her abnormally high glucose level was not corrected because it was considered a normal reaction to the steroids she was being treated with “for brain swelling.” She arrived at the trauma center with a Glasgow Coma Scale score of 5 and a blood pressure of 85/50. She was loaded with Dilantin at the original hospital, and started on 100 mg doses every 8 hours. No neuroimaging was sent with the patient.
See end of chapter for Case Management.
25.1 Introduction
The neurological intensive care unit (NICU) is not specific to just neurologic disease; aberrances in the normal neurologic state can lead to several systemic malfunctions. Obeying the basic principle in neurosurgery of the Monro–Kellie Doctrine, dealing with any factors that obscure the circuitry of the human body can result in altering single terminal synaptic neurotransmitter output to systemic serum hormonal fluctuations; this leads to organ dysfunction and failures and manifest as diseases commonly seen in the medical intensive care unit, along with not-so-common disease states. The most common diagnoses encountered in the NICU include strokes, intracranial hemorrhages, traumatic brain and spinal injuries, cerebral and spinal neoplasms, seizures, and postoperative neurosurgical patients. Many of these patients appear stable on admission but can quickly become the sickest patients in the hospital with a cubic millimeter displacement in neural tissue.
This chapter reviews the chief systemic complications and disease-specific phenomena most often encountered in the NICU patient along with the common treatment recommendations, and it offers recommendations to prevent their occurrence.
25.2 Ischemic Brain Injury
The human brain makes up less than 3% of the total body weight but requires around 25% of the total energy demand of the body; this metabolic rate is 3.5 times greater than that of other primates. The resting brain consumes 25–30 μmol/100 g/min of glucose and 130–180 μmol/100 g/min of oxygen. Normal cerebral blood flow (50–60 mL/100 g/min) is needed to maintain function. 1 ► Table 25.1 demonstrates neurologic change with reduced blood flow. Within seconds of ischemia, slowing of cortical activity is evident on electroencephalogram. This protective mechanism slightly decreases metabolic requirements, but less than 4 minutes of deprived blood flow starts to cause irreversible changes. 2 Efflux of potassium and influx of sodium and calcium due to the energy-dependent ion pump dysfunction lead to cell death. Release of glutamate, a primarily excitatory neurotransmitter, from the cell body exacerbates this process, exciting neighboring neurons in an energy-deprived environment and facilitating calcium entry into the cell, leading to further irreversible injury—known as excitotoxicity.
Responses to ischemic injuries vary in each tissue The vasculature in the brain lacks innervation from sympathetic and parasympathetic systems; rather it is regulated by the parenchymal neurons, primarily astrocytes. The initial cellular response due to insufficient oxygen is production of lactic acid from the anaerobic metabolism of glucose; this leads to a decrease in pH. The acidic environment plus increasing carbon dioxide, from blood outflow, results in a right shift in the hemoglobin–oxygen dissociation curve—lessening the affinity of oxygen for hemoglobin and thus causing its release from the blood cell to the surrounding tissue. The increased carbon dioxide also causes vasodilation in an attempt to increase cerebral perfusion. Additionally, nitric oxide (NO) is produced as a vasodilator, generated by NO synthase in the endothelial cells; however, increased amounts of it can damage the blood–brain barrier, leading to further cerebral edema. 2 The end goal of uncorrected energy requirements is cell necrosis, increased cytotoxic edema, increased intracranial pressure, further injury to surrounding brain parenchyma, and eventual herniation of brain tissue.
Recovery of necrotic tissue after initial insult (primary brain injury) is futile in the central nervous system; medical and surgical efforts are aimed at the penumbra, or perilesional zone, and reducing harmful effects on the remainder of the unaffected tissue. Much of the deterioration that takes place clinically is due to secondary injury, which progresses hours to days after the initial damage. Optimizing blood pressure, brain oxygenation, cerebral perfusion pressure, and intracranial pressures is the cornerstone of successful neurologic intensive care.
25.3 Systemic Complications
25.3.1 Oxygenation
Hypoxia
The oxygen concentration in serum is the combination of oxygen bound to hemoglobin and the oxygen dissolved in plasma. It can be expressed by the following equation:

The neurosurgeon does not need to memorize this equation, but a fundamental understanding of it is important for critical care. In this equation, the first bracket (A) is the oxygen content contributed by hemoglobin, and the second bracket (B) is oxygen dissolved in the serum.
The oxygen-binding capacity of hemoglobin (expressed in mL/g; 1 gram of hemoglobin will bind 1.34 mL of oxygen) is 1.34. One gram of hemoglobin normally binds 1.39 mL of oxygen; however, a small percentage is carboxyhemoglobin and methemoglobin so a value of 1.34 was found to be more representative. This value is multiplied by the concentration of hemoglobin and the percent of hemoglobin that is saturated (obtained from the pulse oxygenation).
The solubility of oxygen relies on the partial pressure of oxygen (PPOxy) and the solubility coefficient (0.003 mL/dL/mm Hg) of oxygen, which is *expressed at 37°C.
The following calculation assumes hemoglobin concentration of 15 g/dL, pulse oxygenation at 99%, partial pressure of oxygen in serum at 95 mm Hg, and body temperature of 37°C:

► Table 25.2 is tabulated using the above equation. Given the above calculations, there is a total of about 785 mL of oxygen available in the serum. The average resting human body consumes about 250 mL/min. This means a little over 3 minutes of oxygen is available in serum, if 100% is released to tissue.
Hypoxemia is defined by decreased oxygen in the serum, measured indirectly by the partial pressure of oxygen in serum. Hypoxemia can lead to tissue hypoxia. Cerebral hypoxia can be detrimental; loss of blood flow for 5 minutes is sufficient to cause irreversible changes. On the contrary, a similar change to cardiac myocytes requires 30 minutes. 2 Moreover, hypoxia leads to a disruption of the normal blood–brain barrier. The process of breakdown is multifactorial but is thought to be related to enhanced production of vascular endothelial growth factor, nitric oxide, and inflammatory cytokines. Experimental studies have demonstrated benefits of using simvastatin, minocycline, and melatonin in aiding with this hypoxic breakdown. 3 Avoiding a breakdown of the blood–brain barrier can reduce vasogenic edema and further aid in decreasing intracranial pressures.
After sufficient oxygenation of the blood has been achieved, the next step is oxygen delivery. The amount of oxygen delivered to tissue is dependent on the oxygen content on the serum (mL/dL) as defined earlier, multiplied by the cardiac output (CO)(L/min). Simplified, the equation is as follows:

A 10 multiplier is added to convert mL/dL to mL/L for the oxygen content. The average oxygen delivered is around 1,000 mL/min in the average human adult. Not all the oxygen delivered to the capillary bed is extracted; this can be calculated by comparing the oxygen content in the inflowing artery to the outflowing vein.

By extracting common variables, this equation is often simplified as follows:

Oxygen-deprived arterial blood will generally result in decreased venous oxygen content as it traverses the capillary bed. This is the principle behind measuring the venous oxygen content of the jugular vein (SjVO2) in neurointensive care patients. This practice has recently been replaced by brain tissue oxygen tension (PBtiO2) monitoring with intraparenchymal devices (e.g., Licox Brain Oxygen Monitoring System, Integra Neurosciences).
Hyperoxic Therapies
Hyperoxic normobaric therapy has been shown to be beneficial in the acute phase of brain injury and stroke. The most popular study was performed on patients with severe traumatic brain injury treated with 100% FIO2, started within 6 hours, and continued for 24 hours. Intracerebral microdialysis demonstrated increased glucose and decreased glutamate and lactate levels. 4 Others have demonstrated reduction in lesion volume up to 100 mL and improved perilesional tissue oxygenation as measured by oxygen-15 positron emission tomographic scan. This was further verified by other researchers with hydrogen-magnetic resonance spectroscopy detection of N-acetylaspartate (a neuron-specific marker of mitochondrial dysfunction), which was found to be lower in the perilesional tissue with hyperoxic therapy. 5 Similarly, in patients with middle cerebral artery stroke, 40% FIO2 as demonstrated decreased mortality and fewer complications. 6
Hyperbaric oxygen therapy has also been tested in traumatic brain injury. 7 , 8 , 9 , 10 Normal atmospheric pressure at sea level is 1 ATM or 760 mm Hg. With hyperbaric treatment, up to 3 ATM of pressure is applied; this causes the oxygen content of plasma to rise from 0.3 to 6.6 mL per 100 mL of blood. Hemoglobin oxygen content is affected at a very minuscule level, not enough to be clinically significant. This would cause the above serum oxygen of 20.2 to increase to 26.5 mL/dL. The proposed benefit of this therapy is vasoconstriction; the increased oxygen content requires less blood volume to meet oxygen demands. 7 This is the same mechanism proposed for crush injuries and burns for other tissue; going back the Monro–Kellie doctrine, less blood content means decreased intracranial pressure. An average improvement of 2.68 GCS points has been found in the literature for traumatic brain injury patients treated with hyperbaric therapy. 11 It has also been shown to reduce brain inflammation in animal models. 12 Further trials are needed in human patients before this becomes standard therapy.
Ventilated Patients
In ventilated patients, common etiologies of hypoxia must be aggressively determined. To maintain adequate cerebral and systemic oxygenation, oxygen-carrying capacity, acid–base balance, and pulmonary pathologies should be determined. Some key markers that signal poor oxygenation are the alveolar–arterial gradient, lactate level, base deficit, and serum bicarbonate.
The base deficit is defined by the amount of base that must be added to 1 L of blood to raise the pH to normal (7.40). It has been used as a marker for injury severity in trauma and its normalization as a goal to reach an end point in resuscitation after acute injury. 13 Serum bicarbonate can be used in a similar manner but is less specific than base deficit, albeit easier to obtain through venous sample. 14
In the setting of metabolic acidosis, Winter’s formula is helpful in determining whether respiratory compensation in present. It is given as follows:

The patient’s actual PCO2 is compared to the range formulated from the above equation. If the patient’s PCO2 is higher than the range predicted by Winter’s formula, then primary respiratory acidosis is also present. If the PCO2 is lower than the range, then primary respiratory alkalosis is present. 15 For example, if the patient presents with metabolic acidosis and is found to have a pH of 7.42 and bicarbonate of 18,

The patient’s expected PCO2 should be between 33 and 37 mm Hg if no instance of respiratory acidosis or alkalosis is present.
25.3.2 Anemia
Anemia is conventionally defined as a decrease in red blood cells or hemoglobin in the serum. A more valid and functional definition is a decrease in the oxygen-carrying capacity of the blood. 16 The optimal goals for hemoglobin and hematocrit values are not well established. However, observing the equation for oxygen content above, it is evident that hemoglobin plays the largest role in maintaining an adequate level of oxygenation in the blood. Patients with multisystem trauma or prolonged stays in the NICU are at greater risk of developing anemia of various etiologies. All primary sources of bleeding should be aggressively sought. Patients with blunt chest or abdominal injury should be evaluated for hemothorax and retroperitoneal bleeding, respectively. Other rare causes include medication reaction but are usually accompanied by hemolysis.
Hypoxemia is conventionally measured through partial pressure of oxygen in serum. However, as already described, hemoglobin plays a significant role in serum oxygen concentration. As shown in ► Table 25.3, a 50% reduction in hemoglobin (15‒7.5 g/dL) results in almost equal reduction in oxygen content, whereas a 50% reduction in partial pressure (95‒47.5 mm Hg) results in less than 1% change.
Serum oxygenation | Normal | Anemia | Hypoxemia |
Partial pressure (mm Hg) | 95 | 95 | 47.5 |
Oxygen saturation (%) | 99 | 99 | 99 |
Hgb concentration (g/dL) | 15 | 7.5 | 15 |
Hgb-bound oxygen (mL/dL) | 19.9 | 9.9 | 19.9 |
Dissolved oxygen (mL/dL) | 0.29 | 0.29 | 0.14 |
Serum oxygen content (mL/dL) | 20.2 | 10.2 | 20.04 |
Volume (L) | 1.25 | 1.25 | 1.25 |
Total oxygen (mL) | 252 | 128 | 250 |
Serum oxygen reduction | 49% | 0.01% |
A normal hematocrit ranges from 40 to 54% for males and 38 to 47% for females. A hematocrit greater than 30% is generally used to optimize cerebral blood flow. 17 , 18 Newer studies for nonneurosurgical patients have shown evidence for adoption of conservative transfusion thresholds. One study claims to provide evidence for a transfusion threshold of 7 g/dL for head-injury patients; however, the lowest mean for the experimental arm was 9.6 g/dL and thus failed to provide significant evidence. 19 With a lack of transfusion studies specific to neurosurgery patients, when caring for the organ with the highest oxygen requirements, we recommend a more liberal approach by keeping hemoglobin concentrations greater than 10 g/dL hematocrit greater than 30% for patients with a central nervous system disease or disorder.
25.3.3 Fever versus Hyperthermia
Fever, or pyrexia, is defined as a body temperature of greater than 101°F or 38.3°C with a normal thermoregulatory system. Any condition capable of triggering an inflammatory response can cause a fever through release of cytokines, which act on the hypothalamus to elevate body temperature. Hyperthermia is the result of a defect in temperature regulation. Both result in elevated body temperatures; however, the former responds to antipyretics (like acetaminophen), whereas the latter does not.
Fever is known to develop in 30% of stroke patients within 48 hours. 20 Moreover, pyrexia in head injuries has been associated with worse outcomes. 21 In the case of head injury, fevers can originate from cerebral tissue necrosis, intracerebral hemorrhage, or even disturbance in central thermoregulation from mass effect. No matter the source, infectious causes should be aggressively sought and treated in addition to antipyretic therapy and cooling measures. One specific cause of fever seen with head injury is sympathetic storming; this phenomenon is discussed later in this chapter but should always be kept as a possible differential for fever when other workup is negative. Other possible causes to keep in mind are drug-induced fevers, transfusion reactions, and postoperative fevers.
Pyrexia is a potent vasodilator and can raise intracranial pressure and cerebral metabolic requirement for oxygen (CMRO2). The increase in CMRO2 is one of the primary reasons that fever should be avoided in the NICU. Moreover, increased temperature causes a decrease in hemoglobin’s affinity for oxygen. As discussed earlier, the goal should be to keep cerebral tissue oxygenation at the ideal levels. Untreated pyrexia will lead to hypoxia and may lead to strokelike symptoms, which manifest as an acute change in neurologic exam. An additional consideration is to avoid shivering when getting the patient to a euthermic state; excessive shivering can lead to rhabdomyolysis.
25.3.4 Electrolyte Disturbances
Aberrant electrolytes are the most common complication seen in the NICU, occurring in 59.3% of the Traumatic Coma Data Bank register. 22 They are primarily in the category of early complications, seen from the time of admission through the first 5 days. It is therefore imperative to monitor fluid status, including total intake and output, daily body weight, and color and specific gravity of urine, to avoid unnecessary cerebral edema or volume contraction. Consideration must be made for patients on chronic diuretics, nasogastric or orogastric suction tubes, or with fever or diarrhea. Serum sodium and glucose are principally addressed due to their effects on serum osmolality (see equation below). Sodium is present intra- and extracellularly and cannot easily diffuse passively between the two compartments; observing the osmolality calculation, one can easily surmise that sodium is the major determinant of tonicity.

Additionally, viscosity becomes an issue with increasing serum osmolality. When blood is flowing through capillaries that are less than 6 μm in diameter, some smaller than an erythrocyte, hyperosmolar serum can cause a decrease in the normal cerebral blood flow. This retardation in blood flow can deprive neural tissue of essential nutrients and lead to ischemic changes if minimal metabolic requirements are not maintained.
Abnormalities in serum potassium are frequently encountered due to gastric suctioning, diarrhea, and medications. Along with correcting the underlying pathology, normal serum concentrations should be maintained. As already described, serum bicarbonate can be used as a marker for hypovolemia, especially in trauma patients. If other signs of volume depletion are present, efforts should be made to promptly correct the deficit.
25.3.5 Hyperglycemia
Glucose is vital for almost all cellular processes and is the primary source of energy for cerebral tissue. The passage of glucose is facilitated by a family of membrane transport proteins known as GLUT (glucose transporter). Many variations of these transporters exist, but GLUT1 and GLUT3 are the primary ones employed by cerebral and neuronal tissue. GLUT1 was found to be the primary transporter at the blood–brain barrier but is also found in other regions of the body. The transporter of interest is GLUT3, which is found almost exclusively in the neuronal population and has a fivefold affinity for glucose. This is thought to be the evolutionary advantage because neural tissue is the last organ you would want to deprive of energy during times of prolonged starvation. But this advantage becomes detrimental during brain injury.
Many studies have shown worse outcomes in NICU patients with poor glucose control. Hyperglycemia usually reflects a stress reaction, mediated by the sympathetic response that prevents glucose entry into nonessential fight-or-flight tissue, and can worsen outcome after traumatic brain injury, likely due to increased cerebral edema. 23 , 24 At the writing of this book, the GAMES trial is under way from which preliminary data have shown a continuous glyburide infusion to be associated with decreased cerebral edema. 25 Conversely, serum glucose should not be kept under tight regulation; strict regulations resulted in increased metabolic load, and cerebral microdialysis demonstrated more frequent critical reductions in glucose and elevations of lactate–pyruvate ratio. 26 We recommend blood glucose < 150 mg/dL.
A secondary effect of hyperglycemia is the transitional shift of water from the intracellular compartment into the serum. This dilutes the serum and can present as pseudohyponatremia. For every 100 mg/dL increase in glucose, there is a 1.6 mEq/L reduction in sodium. As the hyperglycemia is corrected, by the glucose shifting into cells, the water follows and autocorrects the osmolality. An inverse effect of hyperglycemia is hypercoagulability and increased serum viscosity; increased serum glucose concentrations have been shown to increase serum fibrinogen. 27 Increased serum viscosity and increased risk of thrombus formation are both detrimental complications if occurring in the cerebral vasculature; ultimately leading to ischemic injury.
25.3.6 Blood Pressure
Multiple methods to measure blood pressure exist in the NICU. Indirect methods with the pressure cuff are quick and easy. Many hospitals have transitioned from the auscultatory method to the oscillometric method, which has been shown to give more accurate measurements. Direct measurements are invasive but can give a real-time measurement. Normotension, and in some cases, hypertension, is an essential state to maintain in the NICU.
Hypotension
Patients in the NICU frequently have episodes of hypotension. In multitrauma patients, other etiologies must be entertained, necessitating examination of pulse pressure, oxygen saturation, arterial blood gases, and cardiopulmonary and abdominal compartments. In patients with severe traumatic brain injury (Glasgow Coma Scale score ≤ 8), it has been shown that a single episode of systolic blood pressure < 90 mm Hg, from injury to arrival at the hospital, doubles the mortality. In the NICU, eliminating hypotension would reduce unfavorable outcome (Glasgow Outcome Scores 1, 2, and 3) by 9.3%. 22 Additionally, patients with spinal cord injuries are prone to sustained hypotension, which, if not corrected, can lead to infarction.
To limit the effects of hypotension, the following recommendations are made:
Patients with severe traumatic brain injury should have cerebral perfusion pressure > 50 mm Hg in most cases.
Patients should be kept euvolemic to slightly hypervolemic, with a normal central venous pressure 6–8 mm Hg.
Episodes of hypotension/suboptimal cerebral perfusion pressure should be treated first with fluids, then with vasopressors as necessary.
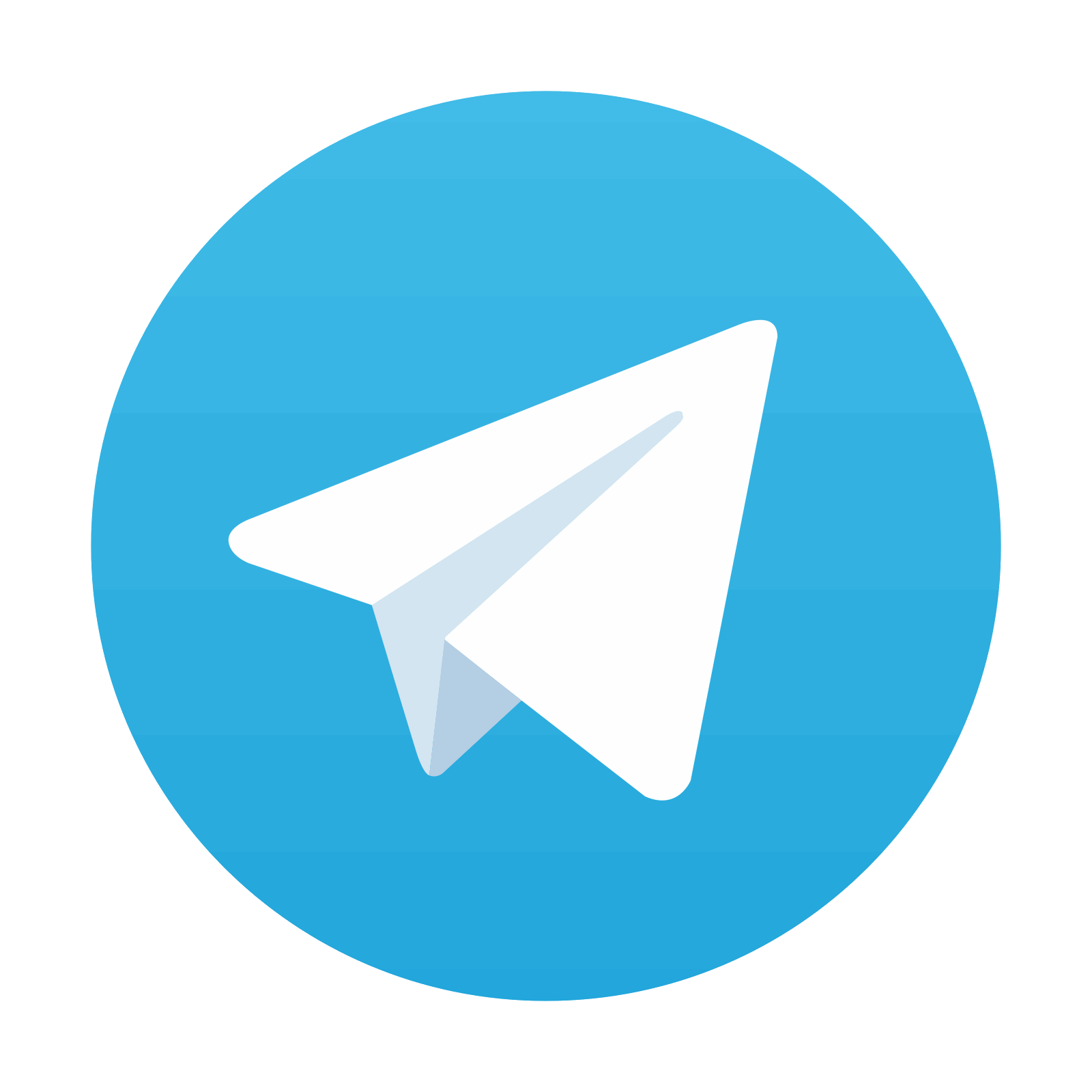
Stay updated, free articles. Join our Telegram channel

Full access? Get Clinical Tree
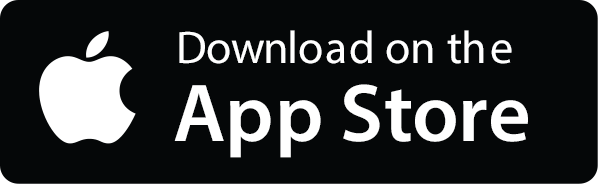
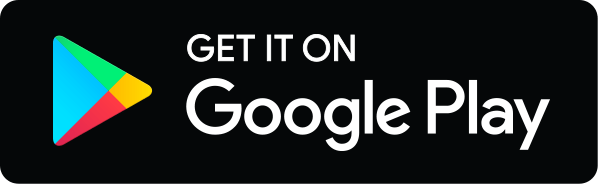
