27 Functional Hemispherectomy at UCLA
Cerebral hemispherectomy remains one of the more common and conceptually dramatic types of pediatric epilepsy surgery. In the 2004 International League Against Epilepsy survey of 20 pediatric epilepsy surgery centers in Europe, the United States, and Australia, cerebral hemispherectomy accounted for 16% of all surgical procedures.1 Even though cerebral hemispherectomy for epilepsy dates back to the early 1950s,2 the idea that surgeons can remove half of the brain successfully still attracts the attention of the lay media as new and astounding. The public often assumes that children after this operation must be in a vegetative state without language or personalities and is amazed to find a smiling playful interactive child starting to walk and talk. Hence, even after 50 years there is still considerable interest in the techniques of cerebral hemispherectomy in the treatment of refractory epilepsy.
This chapter will focus on one type of cerebral hemispherectomy as developed and practiced at the University of California, Los Angeles (UCLA) since 1998.3 Our procedure was designed for infants and small children with small malformed ventricular systems from severe cortical dysplasia and other malformations of cortical development. Initially, we outline the historical development and the rationale of the cerebral hemispherectomy performed at UCLA and describe the operative technique. This will be followed by presentation of the UCLA hemispherectomy cohort’s clinical features and characteristics. The last section assesses UCLA’s functional cerebral hemispherectomy along with ideas for future development.
History and Rationale for Cerebral Hemispherectomy at UCLA
The cerebral hemispherectomy technique performed at UCLA was developed based on the most common types of patients referred for the operation and our prior experience with anatomical4,5 and Rasmussen functional procedures.6 At UCLA, the typical patient referred for cerebral hemispherectomy is a child under the age of 3 years with severe cortical dysplasia and hemimegalencephaly ( Table 27.1 ).7 This patient population usually weighs less than 15 kg at surgery (some less than 6 kg) with a circulating blood volume of 500 mL to 1000 mL. Typically, the ventricular system is small or malformed, leading to challenges in operative dissection ( Fig. 27.1A-D ). Prior experience at our center with the anatomical cerebral hemispherectomy, in which the deep structures of the basal ganglia and thalamus were removed, was associated with the highest rate of seizure freedom. However, that procedure in small children with severe cortical dysplasia was also coupled with the most operative blood loss and complications.3,8 With the Rasmussen functional cerebral hemispherectomy, fewer patients were seizure free postoperatively, many patients required second operations for removal of deep brain structures associated with generating seizures, and patients experienced operative blood loss comparable to those undergoing anatomical hemispherectomy. Based on our patient population and prior surgical experience, from 1997 to 1998 the senior author developed a modified version of the functional cerebral hemispherectomy that would address these issues.
The modified UCLA functional cerebral hemispherectomy was designed (1) to optimize the probability of postoperative seizure freedom by removing deep brain structures, (2) to restrict surgical blood loss, and (3) to reduce operative complications in young infants and children with large dysplastic brains. The key concept in the development of this modified procedure was the realization that from a technical perspective, the easiest cases were those cerebral hemispherectomy patients with a prior history of perinatal middle cerebral artery (MCA) infarcts. In these cases, the ventricular system was enlarged, and the deeper structures were already atrophic.
Histopathology | Percentage of Cases (n = 96) |
Malformations of cortical development (58%) | |
Hemispheric/multilobar cortical dysplasia | 34% |
Hemimegalencephaly | 22% |
Tuberous sclerosis complex | 2% |
Other substrates (42%) | |
Rasmussen encephalitis | 18% |
Middle cerebral artery infarct/ hypoxia-ischemia | 17% |
Infections (herpes, bacterial) | 3% |
Tumor | 2% |
Trauma | 1% |
Sturge-Weber | 1% |
The central idea for the UCLA operation was to create a similar operative field by dividing the MCA branches in the anterior sylvian fissure and removing the central operculum, including the lateral thalamus and basal ganglia.9 Removal of the central operculum and deep structures disconnects almost all of the connections between the ipsilateral cerebral cortex to the ipsilateral thalamus and brainstem and provides ample exposure of the ventricular system for the intrahemispheric disconnections. The created space accommodates any postsurgical brain swelling. This technique reduces the size of the craniotomy compared with anatomical and Rasmussen cerebral hemispherectomy and, along with the early division of the MCA, minimized operative blood loss. This procedure was also designed to eliminate the need to retract firm dysplastic portions of the cerebral hemisphere with short friable veins to gain access to the anterior and posterior cerebral arteries, as necessary, for an anatomical hemispherectomy. Furthermore, the technique preserves the arachnoid granulations near the superior sagittal sinus aiming to reduce postsurgical hydrocephalus. Lastly, the functional hemispherectomy keeps enough tissue in the cerebral vault so that with growth the infant skull is less asymmetrical compared with children after anatomical hemispherectomy ( Figs. 27.1E and 27.1F ).

The anatomical aims of the technique are the same as other cerebral hemispherectomy operations: remove or disconnect all the connections of the ipsilateral cortex to the ipsilateral thalamus and disconnect the connections between the two cerebral hemispheres. This is accomplished by removal of the central operculum and the mesial disconnection that follows the tentorium incisura. The therapeutic goal is to eliminate seizures as soon as possible to optimize cognitive and psychosocial development.10
Presurgery Evaluation
Chapter 24 provides a general overview of the presurgical evaluation of patients for cerebral hemispherectomy. At UCLA, the decision to offer cerebral hemispherectomy is a risk-benefit analysis addressing the risks of continued seizures including increased mortality and severe developmental delay from an epileptic encephalopathy versus the benefits of stopping the seizures early in life and risks of surgery.11 There is no age or size that is too small for cerebral hemispherectomy if the seizures are severe enough. However, children generally weigh at least 5 kg or more before surgery.3 Patients usually have tried three or more antiepilepsy drugs (AEDs) before presurgical evaluation. In infants and young children, the presurgical evaluation takes place within weeks to months prior to surgery. Children with refractory infantile spasms and status epilepticus are referred for presurgical evaluation promptly.12,13 Standard presurgery evaluation includes scalp electroencephalography (EEG)/ video telemetry, magnetic resonance imaging (MRI), 2-de-oxy-2[18F]fluoro-D-glucose (FDG)-positron emission tomography (PET), and, more recently, magnetoencephalography (MEG).14–17 Even with high field strength MRI, we have found that FDG-PET is still very important in the presurgical evaluation, especially for infants and young children with cortical dysplasia, because the area of hypometabolism is often larger than the abnormality identified by structural MRI.18 Hemiparesis is not a requirement before consideration of cerebral hemispherectomy at our institution.
Operative Technique
This section outlines the operative technique of functional hemispherectomy as performed at UCLA in 2008. As with any surgical technique, the operation has evolved over time, and additional subtle alterations can be expected as the team gains more experience.
Preincision
After induction of general anesthesia and endotracheal intubation, a transurethral catheter is inserted. The anesthesia team then inserts an arterial line, a central venous catheter (groin or neck placement), and two peripheral intravenous lines as large as the child’s veins can tolerate. Laboratory parameters, including hematocrit, blood chemistry, acid-base status, coagulation, and fibrinogen level, are performed throughout the surgery. For children weighing less than 10 kg, and especially for infants with hemimegalencephaly, blood must be available in the operating room before skin incision. Blood replacement begins with the skin incision in smaller children. Antibiotics, steroids (dexamethasone; 0.1 to 0.5 mg/ kg), and mannitol (1 g/kg) are given before skin incision.
Head position is lateral with the skull slightly higher than the chest, and the lateral frontal-temporal region is parallel to the floor ( Fig. 27.2A ). The patient is supine, and a small roll is placed to elevate the ipsilateral shoulder. The child is positioned on a sheepskin pad, and all pressure points of the heels and elbows are padded. In children older than 18 months, a Mayfield head holder (Integra, Plainsboro, NJ) is used; and in infants younger than 18 months of age, a pediatric horseshoe is preferred. When positioning on the horseshoe, particular attention is paid to the dependent pressure points over the contralateral eye, orbit, and ear. A T-shaped incision is marked on the skin ( Fig. 27.2A ). One arm of the incision is paramedian at the level of the ipsilateral midpu-pillary line, extending from the hairline to the parietal region, staying parallel to the sagittal suture. The other arm of the incision begins just in front of the tragus at the zygoma, extends vertically, and then curves slightly frontally to intersect with the first incision at a 90-degree angle at or near the coronal suture. Local anesthetic with epinephrine is infused into the skin incision and at the Mayfield pin sites.
Opening
The opening is a standard osteoplastic craniotomy with minor modifications to expose the anterior middle fossa and orbital frontal floor ( Fig. 27.2B-D ). We do not routinely use intraoperative neuronavigation. The skin flaps are elevated, preserving the temporalis muscle, fascia, and pericranium ( Fig. 27.2B ). In children, we use monopolar electrocautery sparingly. The skin flaps are wrapped in lap sponges and are held back with fishhooks and rubber bands are attached to the Greenberg retractor system (Codman, Raynham, MA). We elevate and incorporate into the skin flap the frontal-temporal fat pad and underlying fascia extending from the frontal portion of the zygomatic arch to the root of the zygoma ( Fig. 27.2B ; white asterisk). The temporalis muscle and fascia are incised along its fibers in the low temporal region to expose the zygomatic root and the frontal “keyhole” ( Fig. 27.2B ; black arrows).
Although the osteoplastic bone flap takes a bit more time, the exposure suits our purpose, and the cosmetic effect after surgery is superior. We also feel the risk of osteomyelitis is reduced with the osteoplastic bone flap, and, by retaining the blood supply, most children form a lifelong bony union. Burr holes are drilled just above and anterior to the zygo-matic root, at the posterior margin where the ventricular catheter will eventually exit, behind the coronal suture, and in the frontal keyhole. The burr holes are connected with the drill paying attention to expose 2 to 3 cm of the orbital frontal floor, and the bone flap is “cracked” between the frontal and temporal burr holes. Additional bone is removed to expose the anterior middle fossa, middle fossa floor, and orbital frontal floor ( Fig. 27.2C ; white arrows). Bone wax is used sparingly and only in portions of bone edge that are bleeding to maximize postsurgical bony fusion. The dura is opened with the pedicle based between the frontal-temporal areas and tacked back ( Fig. 27.2D ). At this point, we inspect the lateral cortical surface of the brain for abnormal gyral patterns. We routinely perform intraoperative electro-corticography (ECoG) to identify sites to biopsy for research purposes. ECoG is performed under standard anesthetic conditions without inhalation anesthetics.14

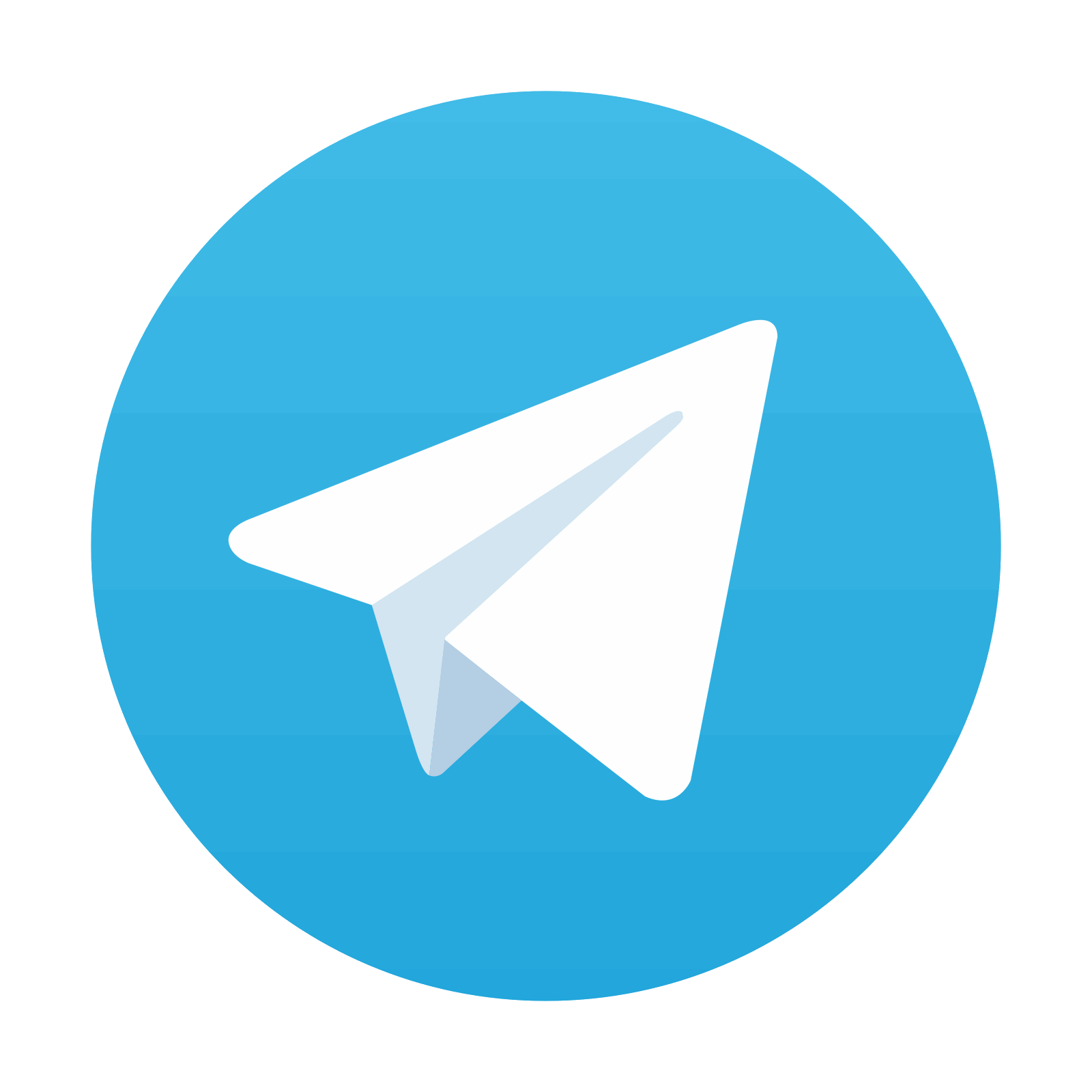
Stay updated, free articles. Join our Telegram channel

Full access? Get Clinical Tree
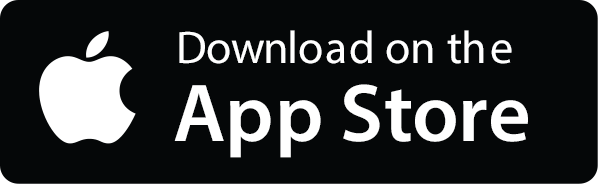
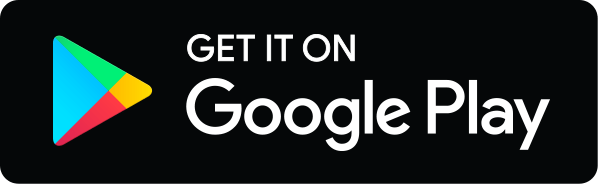
