3 Animal models of hydrocephalus
Adult Hydrocephalus, ed. Daniele Rigamonti. Published by Cambridge University Press. © Cambridge University Press 2014.
Pulchra sunt, quae videntur pulchriora quae sciuntur longe pulcherrima quae ignorantur. (Beautiful is what we see, even more beautiful what we know, but most beautiful what is still unknown to us.)
The purpose of any animal study in human medicine is in very general terms to learn about human conditions. Thus, considerations on transferring results from the laboratory to man – either to normal human physiology or to human disease depending on the purpose and design of the study – is pivotal to all studies of this kind. As details about particular models are easily accessible through goal-directed internet searches, the aim of this chapter is to convey an overview of the general principles in different types of animal models. In addition, some of the ethical and historic contributions are included, as they are important, but generally less present in our awareness when we search for scientific information.
In order to address these very general statements, this chapter will describe the following issues:
ethics of animal models;
formal requirements – general to any experimental model;
specific requirements for models of hydrocephalus;
existing and preferred models;
recent and current research areas in experimental hydrocephalus.
Ethics of animal models
Ethical standards for experimental studies involving animals are legally set by laws and regulations. Although these may differ between individual countries, they all share the same mindset and purpose – i.e. to ensure animal welfare by reducing or if possible completely preventing suffering in animals used for experiments. Within the EU, this is also covered by EU directive No. 86/809.
The ethical mindset behind the legislation demands that animal models must provide new knowledge. The number of animals used and the inflicted suffering in a given experiment must be balanced against and justified by future progress in treatment of human diseases and definition of important issues for health improvement. As early as 1959, standards were coined in the publication Principles of Humane Experimental Technique by Russell and Burch [1]. They identified replacement, reduction, and refinement as important ethical principles, which are still the basis for present day legislation and ethics [2,3].
The replacement principle requires that alternative methods to animal experiments for obtaining the same knowledge must be exploited first. This entails techniques of cell, tissue, or organ cultures. Relative replacement is a recent modification of this principle, in which in vitro experiments and/or computer models are conducted in parallel to an animal study thus replacing part of the study. Alternatively animals with a lower phylogeny can also be included in such experimental designs [4]. In vitro studies generally can contribute knowledge at cellular and molecular levels, but are of limited use in elucidating integration between several biological factors and between organ systems in an entire organism. Experimental animals therefore fill the gap between in vitro research and studies in humans, where they typically are included in protocols designed to investigate pathogenesis and pathophysiology of diseases, to test toxicology in drug development, and in preclinical studies [5].
The reduction principle entails that planning and execution of an experimental study considers bringing the number of animals used to a statistically safe minimum by precision in study design and by meticulous pre-experiment analysis of statistical power. Technical improvements ensuring reliability in measurements thus reducing methodological error is another way to decrease random variation and thus make it possible to reduce the necessary sample size. In keeping with this principle, data for as many biological parameters as possible should be provided by each experiment if this can be done without increasing the exposure to suffering unnecessarily.
Refinement refers to improvement of experimental methods with the specific purpose of reducing distress. Inherently this comprises optimizing anesthesia and analgesia. Development of transgenic animal models removes the need for infliction procedures in order to induce a pathological condition post-partum and may thus be regarded as part of this principle.
Formal requirements – general to any experimental model
The advantage of laboratory investigations is the ability to create a biological system as simplified as possible by avoiding or eradicating factors with a confounding influence on the results. The cleanest setting in this respect is of course cell or organ cultures, where the biological environment can be maximally controlled. Naturally, such simplistic biological systems carry substantial inherent limitations, and investigations in entire vertebrate organisms are still the closest to replace investigations in the human body.
Animal models resemble human disease by conditions which are genetically determined, naturally acquired, or induced by the investigator. Apart from producing the desired disease or condition, a large number of physiological factors must be taken into account. Some may be obviously related to the disease in question (e.g. a female animal model in investigation of ovarian cancer); others may be less obvious, but nevertheless important to standardize in order to reduce variability and maximize reproducibility and reliability [6].
Gender is important due to the influence of hormonal cycles and gender-related behavior. In addition, the possibility of gender-related disease effect and response to intervention must be considered. The usual solution is to use animals of male gender for initial experiments and continue with these unless the disease or question addressed requires female animals; e.g. if studying idiopathic intracranial hypertension (IIH), which is a condition related to hydrocephalus with a much higher incidence in young women.
Weight and age of the experimental animals. The neonatal, infant, and juvenile organism is in many respects different from adults both in humans and animals. Particularly when studying diseases of the brain, it is relevant that there is a much higher degree of neuronal plasticity in younger individuals and that the response to disease or injury in the developing brain is different from that in the mature brain. Furthermore, pediatric hydrocephalus, either congenital or acquired at an early age, differs from hydrocephalus in the adult in almost every aspect of the disease including clinical presentation, underlying causes, treatment reactions, complications, and prognosis.
The influence of external factors such as confinement, handling, nutrition, and physical environment (lighting, day/night cycles, temperature, humidity, sound and noise impact) may all influence the stress level and general biological responsiveness of laboratory animals.
Analogy with human pathology, and reproducibility and reliability of the pathological process are extremely important for the validity of the model. Consequently, a model subjected to defined conditions for testing, intervention, or observation must produce identical or closely comparable results regardless of time and place.
A system for evaluating the validity of a given model has been proposed by Tkacs and Thompson [6], who distinguish between predictive and construct validity. Predictive validity deals with the question of accuracy and predictability in transferring observations and data from interventions in the model to clinical intervention in the human organism. This principle is often evident in testing and predicting drug response and can also be used to observe and predict long-term consequences of a disease process with or without different types of intervention. Construct validity is a concept which questions the theoretical rationale behind the model. Clinical, pathological, and physiological similarities are the key issues used to address the congruence between the model and the human clinical condition. Another theoretical principle is the “bedside to bench” concept, according to which specific biological variables most prominent in the human disease must be identified and then “mimicked” in the desired animal model.
Animal models may be classified into the following main types:
A living organism with a hereditary or naturally occurring disease/pathology resembling a similar disease in humans. The limitations are an often less than optimal resemblance to human disease.
A living organism in which changes in one or more variables or in one or more organs are induced with the purpose of altering the normal condition to resemble human disease.
Transgenic animals, in which a specific genetic or hereditary condition is induced by genetic manipulation.
Requirements and challenges for models of hydrocephalus
Creating new insight and hypotheses based on the original clinical problem is the hallmark of animal model research. From this point of view, it seems reasonable to define unanswered questions about hydrocephalus physiology and pathology, to address controversies in its treatment, to systematically collect knowledge on the incidence and types of clinical complications, and then consider which of all these questions and hypotheses can be answered in clinical protocols – and how studies in animal models can clarify those that cannot. Taking this approach, a group of clinicians and scientists with broad expertise in clinical and experimental hydrocephalus proposed ten unanswered questions about hydrocephalus which were most urgently in need of clarification [7].
(1) How do we define hydrocephalus?
(2) How is cerebrospinal fluid (CSF) absorbed normally and what are the causes of CSF malabsorption in hydrocephalus?
(3) Why do the ventricles dilate in communicating hydrocephalus?
(4) What happens to the structure and function of the brain when it is compressed and stretched by the expanding ventricles?
(5) What is the role of cerebrovenous pressure in hydrocephalus?
(6) What causes normal pressure hydrocephalus?
(7) What causes low pressure hydrocephalus?
(8) What is the pathophysiology of slit ventricle syndrome?
(9) What is the pathophysiological basis for neurological impairment in hydrocephalus, and to what extent is it reversible?
(10) How is the brain of a child with hydrocephalus different from that of a young or elderly adult?
This group further pointed out that, for many of the proposed questions, “medical factual knowledge” is based on very incomplete evidence and dogmatic beliefs. As clinical diagnosis, treatment decisions, and handling of complications all rest on this, the group concludes “our understanding of hydrocephalus is not nearly as sophisticated or complete as we might have imagined. Fifty years after the introduction of shunts for the treatment of this previously untreatable disorder, we must acknowledge that the shunt is not a cure for hydrocephalus; it is only a ‘patch,’ and an unreliable one at that. We know a lot about hydrocephalus, but much of what is true about hydrocephalus is strange, and is not necessarily the same as what we think we know. There is surprisingly little scientific basis for many of our commonly held opinions.” They also address the need to think in contexts different to the previous mainstream and thus in reality set the challenge for hydrocephalus animal model research: “we must be willing to expand into an exciting, albeit more complex, scientific frontier of CSF physiology that relies less on bulk flow.”
Existing and preferred models
In general, animal models are classified as rodent and non-rodent types, the background of course being that rodent models are by far the most commonly used because their small size makes handling considerably easier and cheaper. This is also true for animal models of hydrocephalus, where various types of rodent models, particularly rat models, are by far the most commonly used. The difference in size, cerebral phylogenic development, anatomy, cerebrovascular anatomy, and functional organization pose a problem when transferring results from rodent models to the human brain [5,6]. Anatomical differences frequently also imply differences in physiology and metabolism, and studies in “higher” animals are generally recommended to supplement rodent studies [6]. Primates are the closest to humans, but induction and studies of human-like diseases in these involve practical, economic, and ethical problems.
Naturally occurring hydrocephalus is quite rare in animals – exemplified by the fact that application of the search term “hydrocephalus” yields very few results in the veterinary literature [8]. The earliest scientific documentation in a modern sense is the paper written in the 1660s by the Danish scientist and scholar Nicolaus Steno on an anatomical study of a hydrocephalic calf [9]. The general belief at that time held since ancient Greece was that the “spiritus animalis” (CSF) was propagated from the brain through the cranial nerves into the muscles by pumping movements of the pineal gland. The motion of the pineal was determined by the will, and this pumping made the muscles swell and move voluntarily. By meticulous anatomical dissection and stringent scientific deduction based on his observations, Steno was able to disprove this theory by showing that the pineal gland cannot move. He further showed that the ventricles were blocked by a mass, which makes this the first scientific observation defining obstruction of the CSF pathways as a cause of hydrocephalus. This dispute between generally held opinion and rational analysis is still an inspiration for modern hydrocephalus research.
Apart from the study of a genetic predisposition to ventriculomegaly, Chiari malformation, and epilepsy in King Charles spaniels [10], the veterinary literature contains relatively few publications on hydrocephalus, consisting of single case reports on sporadically occurring hydrocephalus in different species including dog [11], cat [12], and parrot [13]. This implies that experimental studies on hydrocephalus are impractical to carry out on the naturally occurring condition, and such studies are thus based entirely on experimentally induced hydrocephalus. Basically, experimental hydrocephalus models are produced either by physical/surgical manipulation introducing an agent into CSF spaces or by genetic alteration of animals.
Hydrocephalus induced by physical or chemical agents
As early as 1914 W. S. Thomas published a paper describing a handful of published reports on sporadic cases of human hydrocephalus secondary to meningitis or mass lesions, concluding that systematic experimental studies require reliable induction by injection of substances capable of blocking the aqueduct or fourth ventricle outlets [14]. In his paper, he meticulously describes preparation of the substance (aleuronate granules), the surgical technique in dogs, and a time course experiment with gross anatomical and microscopic findings. The experiment was further divided into a group of adult and a group of juvenile animals. He was able to produce a severe inflammatory reaction, ependymal proliferation, ventriculomegaly as well as clinical and pathological evidence of increased ICP. Since then it has been recognized that injection of noxious substances into the CSF pathways can reliably cause inflammation and hydrocephalus.
As severe inflammation is not part of the picture in most types of human hydrocephalus except in relation to meningitis, substances which do not produce inflammation have been used and shown to obstruct the CSF pathways, e.g. silicone oil [15] or acrylic glue [16].
Induction of hydrocephalus by injection of kaolin into the basal cisterns was reported by Dixon and Heller in 1932 [17]. Currently, although other substances injected into the basal cisterns have been shown to produce hydrocephalus, kaolin remains the most commonly employed substance to produce hydrocephalus by injection into the CSF spaces. Kaolin is an inert silicate, and although there is no demonstrable inflammation distant from the injection site, it has been speculated whether a reaction to the injected substance could play a role in the biological response in addition to the mechanical obstruction at the injection site. Injection into the cisterna magna, fourth ventricle or both causing blockage of the fourth ventricle outlets and thus obstructive hydrocephalus is the original method, which has been shown to produce hydrocephalus in many different species including rodents, rabbits, cats, dogs, and sheep [17–19] (Figures 3.1 and 3.2). As obstructive hydrocephalus, particularly in adults, accounts for only a portion of human hydrocephalus cases, a method for kaolin deposition into the basal cisterns to create “communicating experimental hydrocephalus” with CSF passage through preserved anatomic fourth ventricle outlets has recently been described [20].
Figure 3.1 T2-weighted serial coronal sections of a normal rat. The ventricular system is very slim, and the uncompressed structures of the brain occupy most of the intracranial volume. Courtesy of Osama Abdullah, Imaging Core Facility, and James P. McAllister II, PhD, University of Utah, as well as Anders Skjolding, MD, Copenhagen University Hospital.
Figure 3.2 T2-weighted serial coronal sections of a rat 2 weeks after induction of hydrocephalus by kaolin injection into the basal cisterns. The ventricles and aqueduct are massively dilated. The cortex and subcortical white matter are severely thinned. Courtesy of Osama Abdullah, Imaging Core Facility, and James P. McAllister II, PhD, University of Utah, as well as Anders Skjolding, MD, Copenhagen University Hospital.
Inducing hydrocephalus by changing the composition of the CSF is an entirely different principle, and includes models of intra- or periventricular hemorrhage [21] or alteration of CSF osmolarity [22]. Intraventricular infusion of mock-CSF may be regarded a model of acute high pressure hydrocephalus analogous to these models [23].
Nonmechanical induction methods, such as viral and bacterial inoculations, can also produce communicating hydrocephalus. However, as these models involve the additional biological response to the infectious agent, they are not recommended for studies of the effects of hydrocephalus per se [18].
Genetically determined hydrocephalus in inbred strains or by genetic manipulation
The HTX rat is a genetically determined model with heterozygous disposition to congenital hydrocephalus due to stenosis of the aqueduct that develops in fetal life [24].
This strain develops very severe obstructive hydrocephalus soon after birth and is therefore the most widely used model for neonatal or juvenile hydrocephalus. Other less used models of inborn hydrocephalus are an inbred Wistar-Lewis rat strain with aqueductal stenosis and several mouse strains, e.g. hy1, hy2, and hyh mice [25–28].
Hydrocephalus can also be induced by transgenic manipulation, i.e. artificial insertion of DNA sequences. Transgenic animals can be produced by injection of foreign DNA into a fertilized egg or developing embryo. Several mouse hydrocephalus models are produced by genetic manipulation [19,28]. One such model is a transgenic mouse in which cytokine transforming growth factor-β1 (TGF-β1) is overexpressed [29]. Knockout models constitute another type of genetic manipulation, in which the function of a gene is removed by mutation or deletion. In hydrocephalus research, this technique has been utilized to remove subsets of the aquaporin water transport protein family (AQP-null mice) [30]. AQP4-null mice show accelerated progression of ventriculomegaly relative to wildtype controls following induction of hydrocephalus [31].
Recent and current research areas in experimental hydrocephalus
A published report of a meeting for hydrocephalus and CSF investigators to discuss advances in animal models highlights research areas in brain development, anatomical and functional characterization of choroid plexus and periventricular structures, biochemical and growth promoting constitution of CSF, and mechanical as well as biochemical tissue damage as main areas where animal research can contribute to the advancement of knowledge about the hydrocephalic brain [26].
The topics in recent publications direct us to areas which are presently regarded as most important in experimental hydrocephalus research, i.e. brain development and anatomy of CSF pathways and associated areas [32–35]; neuroinflammation, tissue damage and repair [36–44]; aquaporins and molecular water transport [45,46]; and biomechanical properties of hydrocephalic brain tissue [20,47,48].
An important advance in experimental studies has been the application of CSF shunting techniques in experimental hydrocephalus [18,42], which offers unique and much needed possibilities for systematic studies on factors in shunt complications – bearing in mind both the dismal actuarial shunt survival coupled with the fact that shunt insertion still accounts for treatment of the great majority of hydrocephalus cases. Shunted animal models also provide a unique potential for insight into questions concerning the destructive effects of hydrocephalus on the brain and its development, as it is rarely possible to obtain brain tissue from humans with hydrocephalus [45]. Which effects and which pathological processes are reversible? Is there a critical time limit for relieving CSF pressure beyond which the pathological effects become irreversible? Shunting was previously only technically possible in animals large enough for implantation of the tubing for clinical use in humans. Procedures and implants have recently been developed also for smaller animals, so that systematic studies on the effects of shunting can be applied to rodents, which remain the most widely used animal models for hydrocephalus research.
Normal pressure hydrocephalus (NPH)
Most of the hydrocephalus animal models are neonatal or juvenile animals and the majority of research is thus directed at congenital or pediatric hydrocephalus. There are, however, models in adult or aging animals that contain the possibility to address NPH-related issues in animal models. Kaolin injection in adult rats is thus proposed as a model of NPH [49,50]. In addition, a minority of HTX rats develop a mild and later occurring hydrocephalus which could also be a potential model for NPH [19].
References







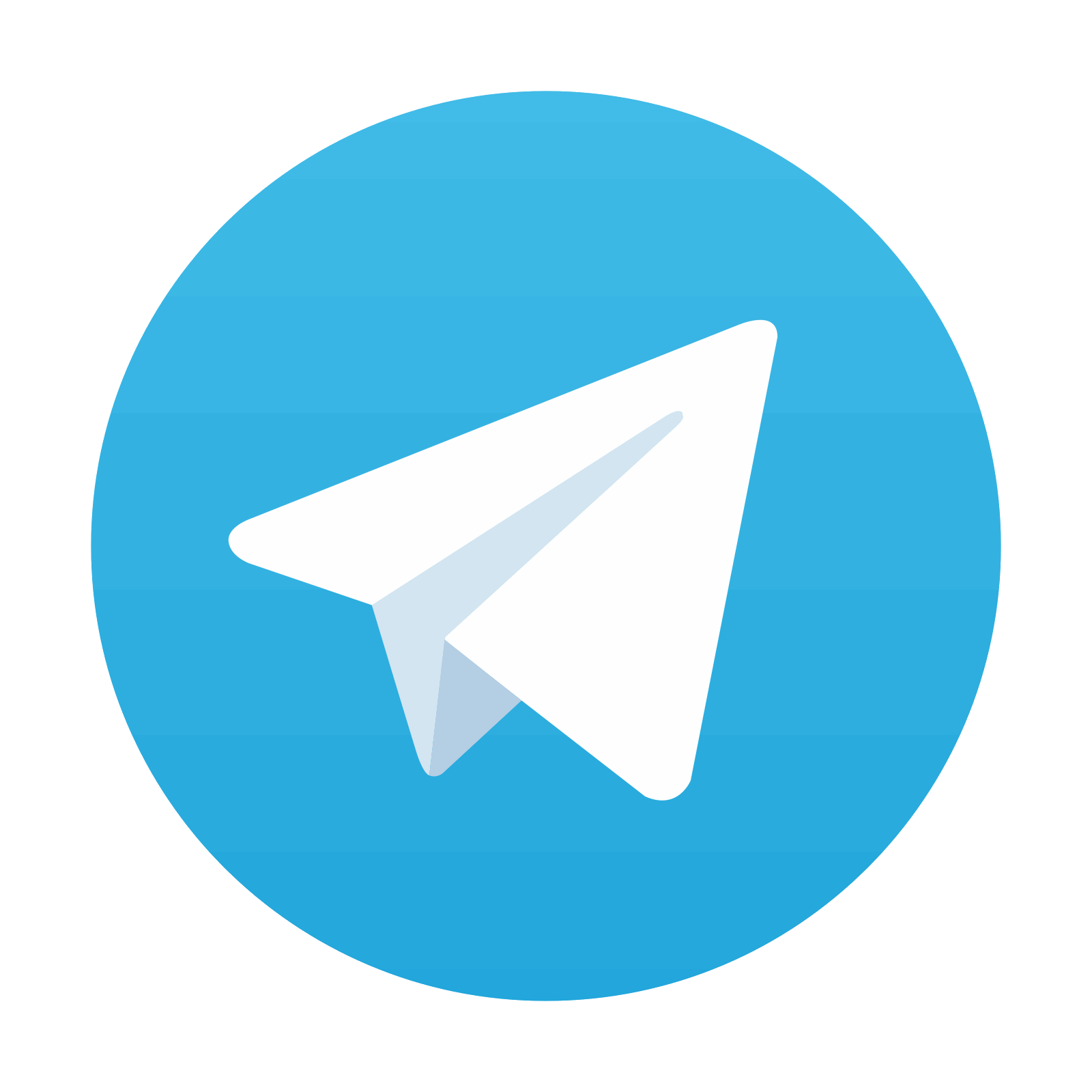
Stay updated, free articles. Join our Telegram channel

Full access? Get Clinical Tree
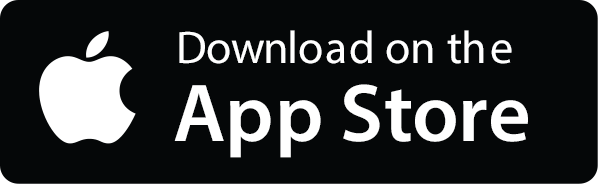
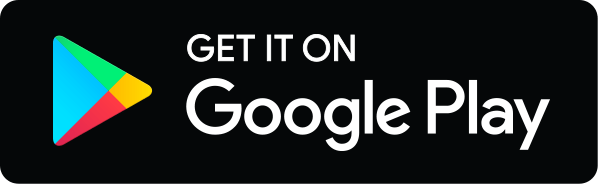
