33 Vagus Nerve Stimulation
Implantable devices for chronic vagal nervous stimulation (VNS) have been used since 1988 as an adjunct in the treatment of medically refractory epilepsy.1 Over the intervening 20 years, a substantial body of literature, including several randomized, controlled trials2,3 has confirmed the usefulness of VNS in refractory epilepsy. In this chapter, we review the historical and scientific basis of VNS and its application in pediatric epilepsy and other neurological disorders.
History of VNS
The first description of VNS for seizure control was by James Corning in 1884.4 Based on the hypothesis that cerebral hyperemia produced seizures, Corning devised an “electro-compressor” combined with a transcutaneous vagal nerve stimulator with mechanical carotid compression. Despite simultaneously decreasing cardiac output and occluding carotid flow, Corning’s results were inconsistent, and his techniques were abandoned.
In the 1930s, Bailey and Bremer first demonstrated that stimulation of the vagal nerve affected electroencephalography (EEG) patterns in cats.5 Several subsequent reports confirmed that manipulation of the vagus by ligation6 or stimulation7–11 decreased EEG spikes in various animal models of seizures. Based largely on these studies, safety and efficacy studies were conducted in primates12 before proceeding with implanting the first VNS devices in human subjects.1,13
Anatomy and Physiology of Vagal Nerve Stimulation
Approximately 80% of the vagus is composed of afferent fibers,14 including myelinated A and B fibers and unmyelinated C fibers. General sensory afferents from the pharynx and larynx, external auditory apparatus, and posterior fossa meninges travel through the vagus en route to the spinal trigeminal tract and nucleus. Perhaps more relevant to VNS physiology, visceral sensory fibers ascending through the vagus project diffusely throughout the central nervous system.15 Although many of these fibers synapse in the nucleus of the tractus solitarius, others travel directly to their target in a mono-synaptic pathway. Several of the targets of these projections, which include the dorsal raphe, locus ceruleus, nucleus ambiguus, cerebellum, parabrachial nucleus, hypothalamus, amygdala, thalamus, hippocampus, and insular cortex, are involved in pathways for rhythmic excitability or have the potential for epileptogenicity themselves.16,17 Indeed, recent work using positron emission tomography, single photon emission computed tomography, and functional magnetic resonance imaging to evaluate VNS-related changes in the brain showed variable alterations in frontal, parietal, and temporal activity but consistent, widespread limbic involvement (reviewed in Barnes et al18), including ipsilateral brainstem, cingulate, amygdala, and hippocampus, as well as the contralateral thalamus and cingulate.18 Further, in a single patient, high-frequency VNS produced a measurable decrease in hippocampal sharp waves.19 Preliminary animal studies have confirmed the inhibitory effect of VNS on Hippocampal activity but have not yet been published.20
Although the precise mechanism of seizure suppression by VNS remains to be elucidated, progress has been made in identifying central effects of peripheral VNS. VNS has been shown to increase neuronal discharges from the locus ceruleus21 and dorsal raphe nucleus,22 and chemically induced lesions of the locus ceruleus attenuate VNS-induced seizure suppression.23 Cross-modulatory pathways exist between the nucleus of the tractus solitarius, the locus ceruleus, and the dorsal raphe nucleus,24 implicating ascending transmitter pathways in the action of VNS. Recent microdialysis experiments have demonstrated that VNS increases norepinephrine levels in the amygdala,25 hippocampus, and cerebral cortex.26 Indeed, the data showing that locus ceruleus neurons are the primary source of norepinephrine to the hippocampus27 and the cortex,28 strongly implicates the role of this nucleus in the mechanism of action of the VNS.
The effect of VNS on cerebrospinal fluid concentrations of amino acid neurotransmitters is less clear. γ-aminobutyric acid (GABA) has been shown to increase in all patients with VNS implanted for seizure control, including patients who did not have a clinical response to the VNS.29 The excitatory amino acid aspartate was noted to decrease in these patients, consistent with an overall inhibitory condition. The serotonin metabolite 5-HIAA also was elevated. In patients who underwent VNS placement for depression, the results were quite different, with no observed change in GABA, 5-HIAA, or norepinephrine.30 Whether the differences in these studies are due to indication for VNS (epilepsy or depression), anticonvulsant or antidepressant medications, or experimental technique or aberration is unknown. Further investigation will be required to parse out these differences.
Acute and long-term alterations in genetic expression have been identified in animal models of VNS. Cunningham et al (2008) reported increased c-Fos expression acutely after initiation of VNS in the nucleus of the tractus solitarius, hypothalamus, stria terminalis, and locus ceruleus. Both expression of c-Fos and the long-term neuronal activation biomarker delta-FosB were observed in the cingulate cortex and dorsal raphe nucleus with chronic VNS only31 Moreover, long-term changes in GABA(A) receptor density have been found in patients with chronically implanted VNS compared with matched control subjects.32
Evidence for VNS in Epilepsy
After the initial case series describing the use of VNS in humans with intractable partial seizures1 and several other studies reporting favorable outcomes,13,33–35 the Vagus Nerve Stimulation Study Group formed to investigate the safety and efficacy of VNS. In conjunction with Cyberonics, Inc., three open-label and two double-blind randomized controlled trials were conducted. The first trial (EO3) included 114 patients older than 12 years of age with at least six seizures per month while on antiepileptic drugs.36 Subjects were randomized to receive low-stimulation (30-second VNS every 90 minutes; 1 Hz; 130 μs; ≤ 3.5 mA) or high-stimulation (30 second VNS every 5 minutes; 30 Hz; 500 μs; up to 3.5 mA) VNS. After 14 weeks of VNS, the high-stimulation group exhibited a 30.9% reduction in seizure frequency compared with an 11.3% reduction in the low-stimulation group, a statistically significant difference.
The second major trial (EO5) enrolled 199 patients older than 12 years with intractable partial-onset seizures.3 Patients were again randomized to low- or high-stimulation for 3 months and evaluated for seizure reduction and device safety. The high-stimulation group exhibited a 28% reduction in seizure frequency versus a 15% decrease in the low-stimulation group. In addition, the high-stimulation group demonstrated improved global evaluation scores, but dysphonia and dyspnea also were more common in this group.
In 1999, long-term efficacy and safety results from the five Vagus Nerve Stimulation Study Group clinical trials (EO1-EO5)2, 3, 36–39 were reported in a meta-analysis.40 A total of 454 patients were followed for up to 3 years using one of three methods for data analysis (last visit carried forward method, constant cohort method, or declining N method). Although only 1 to 2% of patients become seizure free, many derived long-term benefit from VNS. Median reduction of seizure frequency was 35% at 1 year after VNS initiation, 44.3% at 2 years, and 44.1% at 3 years. More than 50% seizure reduction was seen in 23% at 3 months, 37% of patients at 1 year, 43% at 2 years, and 43% at 3 years after VNS initiation. Adverse effects, including hoarseness, decreased over the follow-up period. Additional studies have reported similar results in terms of safety and efficacy of VNS over even longer durations, further confirming the long-term benefits of VNS in select patients.41,42
The U.S. Food and Drug Administration (FDA) approved VNS (Cyberonics, Inc., Houston, TX, USA) in 1997 as an ad-junctive therapy for use in medically refractory epilepsy patients older than 12 years with partial-onset seizures. FDA approval for VNS therapy for the indication of depression came in 2001.
VNS in Pediatric Patients
As noted previously, the studies conducted by the Vagus Nerve Stimulation Study Group enrolled only patients at least 12 years of age. Based on preliminary results from a small series of children in which VNS was placed for medically and surgically refractory seizures,43 the Pediatric VNS Group performed a compassionate use prospective open safety study (EO4).44 A total of 60 pediatric patients (younger than 18 years) with various seizure types were included in EO4, with 16 patients younger than 12 years old. The median reduction in seizure frequency was 23, 42, 34, and 42% at 3, 6, 12, and 18 months, respectively. Two years later, a multi-institutional, retrospective study of 95 pediatric VNS patients reported favorable results for safety and efficacy, with a mean seizure reduction of 36% at 3 months and 45% at 6 months.45
Several reports have now been published that consistently demonstrate the utility of VNS in the pediatric epilepsy population in terms of seizure control46–48 and overall quality of life.49–54 The majority of these studies reported results for all seizure types (absence, atonic, simple partial, complex partial, generalized tonic—clonic, Lennox-Gastaut syndrome) and were not restricted to partial-onset seizures as had been the Vagus Nerve Stimulation Study Group studies. One group reported seizure reduction rates for atonic (80%), absence (65%), complex partial (48%), and generalized tonic—clonic (45%) seizures.49
Although the previously mentioned data are compelling, there have been no prospective, randomized, controlled trials to rigorously evaluate VNS in children. Currently, FDA approval for VNS in the pediatric epilepsy population is limited to adolescents older than 12 years of age with medically refractory partial-onset seizures. Nonetheless, because many children are refractory to anticonvulsants and are not candidates for resective neurosurgical procedures, VNS remains an important adjunctive therapeutic option and is frequently used in an “off-label” capacity.
Relatively little data exist regarding the safety and efficacy of VNS in the very young. Although several studies have enrolled patients younger than 2 years of age as part of a larger series, only one report has evaluated children of this age exclusively. Blount et al described their experience with VNS in six children with a mean age of 20.5 months.55 Of the six patients, five (83%) had a significant decrease in seizure frequency, and there was no treatment-related morbidity. Although preliminary, these data suggest that VNS may be a viable treatment option in very young children with refractory, catastrophic epilepsy.
Pediatric VNS and Specific Seizure Types
Several case series have been published providing early insight into the efficacy of VNS in specific epilepsy types. A multicenter retrospective study of 10 patients with tuberous sclerosis and refractory epilepsy fared particularly well, with 9 of 10 patients experiencing at least 50% reduction in seizure frequency, and half of the patients had at least 90% reduction.56 In a series of six patients with hypothalamic hamartomas, three patients had significant reduction in seizure frequency (25% to more than 90% reduction).57 Interestingly, four of four patients in this series with autism demonstrated improvements in autistic behaviors.57
The efficacy of VNS in Lennox-Gastaut syndrome has been evaluated by several studies. One multicenter retrospective investigation included 50 patients, 36% of whom had tried the ketogenic diet and 10% of whom had previously had a corpus callosotomy.58 Median seizure reduction was 58% at both 3 and 6 months after VNS. Other series have shown similar encouraging results in this difficult patient population, with 25 to 90% seizure reduction.52,59,60 In these patients, response to VNS was found to be best in those with the highest frequency of background EEG activity.46 It should be noted that one group did report disappointing results for Lennox-Gastaut syndrome.61
Two case reports have been published regarding the use of VNS in the setting of status epilepticus. One reported the implementation of VNS in a young adult with status epilepticus elicited by anticonvulsant withdrawal.62 The second case was a 13-year-old boy with status epilepticus in the setting of a long-standing history of epilepsy. In both cases, the status epilepticus was terminated by VNS, and in the second case, available follow-up demonstrated that the patient experienced significant seizure reduction in long-term.63
Operative Technique for VNS Placement
Surgical implantation of VNS is performed under general anesthesia and generally requires 1 to 2 hours of surgical time. At our institution, the operation is performed as an outpatient procedure, although other facilities admit patients overnight for observation. Unless there are special considerations, the VNS is placed on the left side. The reason for choosing the left side is based on the asymmetrical vagal innervation of the heart. Whereas the right vagus is responsible for innervation of the sinoatrial node, the left vagus innervates the cardiac atrioventricular node.64 Stimulation of the left vagus is thus less likely to actively modulate heart rate.
The patient is positioned supine with his or her neck in a neutral position. A gel roll is placed transversely under the shoulders to gently extend the neck. The operating room table is turned 90 degrees clockwise, allowing the surgeon access to the left side of the neck and chest. Before skin incision, 25 mg/kg intravenous cefazolin is administered, and the skin is prepared with antiseptic solution. The surgery begins with a transverse 2 to 3 cm incision placed, when possible, in a natural skin fold located along the mid portion of the sternocleidomastoid muscle (SCM; Fig. 33.1A ). The incision itself is located one third lateral and two thirds medial to the medial border of the SCM and is similar to that used in an anterior cervical discectomy. The platysma is then divided transversely and undermined superiorly and inferiorly to assist with exposure and retraction. The dissection is carried down through the cervical fascia and along the medial border of the SCM. The SCM is then retracted laterally, and the carotid pulse is used to locate the carotid sheath. The sheath is then opened sharply and the vagus nerve is located with the neurovascular bundle. The position of the vagus varies considerably with respect to the carotid artery and internal jugular vein, but generally the nerve is located posteriorly within the sheath and medial to the jugular. A vessel loop is then passed underneath to assist with mobilization of the nerve and subsequent placement of the VNS electrodes ( Fig. 33.1B ). Careful dissection and isolation at least 3 to 4 cm of nerve provides sufficient room for wrapping the two electrodes and anchoring coil.
Once the cervical dissection is complete, a subcutaneous pocket is made in the chest to accommodate the VNS pulse generator. Although the location for this pocket varies with surgeon preference, we use an incision along the lateral border of the pectoralis major muscle to create a subcutaneous pocket directly over the pectoralis fascia in the infraclavicular fossa ( Fig. 33.1A ). The electrodes are then tunneled from the superior limit of the pocket over the clavicle to the cervical incision ( Fig. 33.1C and 33.2A ).
With care to minimize manipulation of the vagus, the helical electrodes are applied to the nerve by grasping the suture ends of each coil to open the configuration (Figs. 33.1C, 33.2B, and 33.2C). Once the coil has been unfurled, the electrode is wrapped around the nerve. This application process is performed first for the anchoring coil, then the positive electrode, and lastly the negative electrode ( Fig. 33.2C ). The vagus, with electrodes applied, is then replaced as close as possible to its original anatomical position within the carotid sheath with care to minimize tension on the leads. A strain-relief loop is then fashioned ( Fig. 33.1C and 33.2A) and sutured with nonabsorbable material to the SCM muscle.

The connector pins located at the distal end of the VNS lead are then inserted into the pulse generator and secured with the manufacturer’s hexagonal torque wrench ( Fig. 33.2D ). The pulse generator is then inserted into the subcutaneous pocket. Any excess length of the lead is coiled directly on the pectoralis fascia deep to the pulse generator to prevent hardware injury if revision surgery is required. Finally, a silk suture is used to secure the pulse generator within its pocket. Both incisions are then irrigated with copious antibiotic-containing saline and closed in layers with absorbable suture and liquid skin adhesive.
Special Considerations in VNS Surgery
As noted previously, because of asymmetric cardiac innervation by the vagus,64 the left side is preferred for placement of the VNS. In fact, the VNS manufacturer Cyberonics cautions that “the safety and efficacy … have not been established for stimulation of the right vagus nerve.”65 However, clinical scenarios exist in which placement of the VNS on the right side may be considered. VNS hardware infections occur in approximately 3 to 6% of cases and generally require removal of the hardware.66,67 In those patients who experience a significant reduction in seizure frequency but require VNS hardware explantation because of infection, placement of a right-sided VNS may be considered.68 A small series of right-sided VNS implantations performed under these conditions showed efficacy in reducing seizure frequency with no cardiac complications.68 Two of the three patients did experience respiratory side effects with reactive airway disease.68
Variations in the surgical technique for VNS implantation have been described to streamline the procedure or to avert predictable surgical complications. Cognitively impaired children undergoing VNS placement frequently tamper with their surgical wounds, increasing the risk of wound infection. To address this concern, alternative locations for the position of the pulse generator, including subpectoral69 or interscapular70 placement, have been reported. In addition, an alternative technique using a single, low-cervical, transverse incision for implantation of both the vagal nerve electrodes and the pulse generator obviates the need for a second incision or the tunneling of electrodes.71

In some cases of revision VNS surgery, particularly in caring for patients transferred from other facilities, the components of the VNS system may not be known. In such cases, routine x-rays of the chest and neck may be helpful not only in detecting clear fractures or disconnections but also in determining the existing VNS hardware and thus which components will be needed for the revision. For example, a chest x-ray will show the number of lead connector pins necessary for interfacing with the pulse generator. VNS Therapy Models 101 and 102R both accommodate two lead connector pins, whereas Model 102 accepts a single lead connector pin input only.
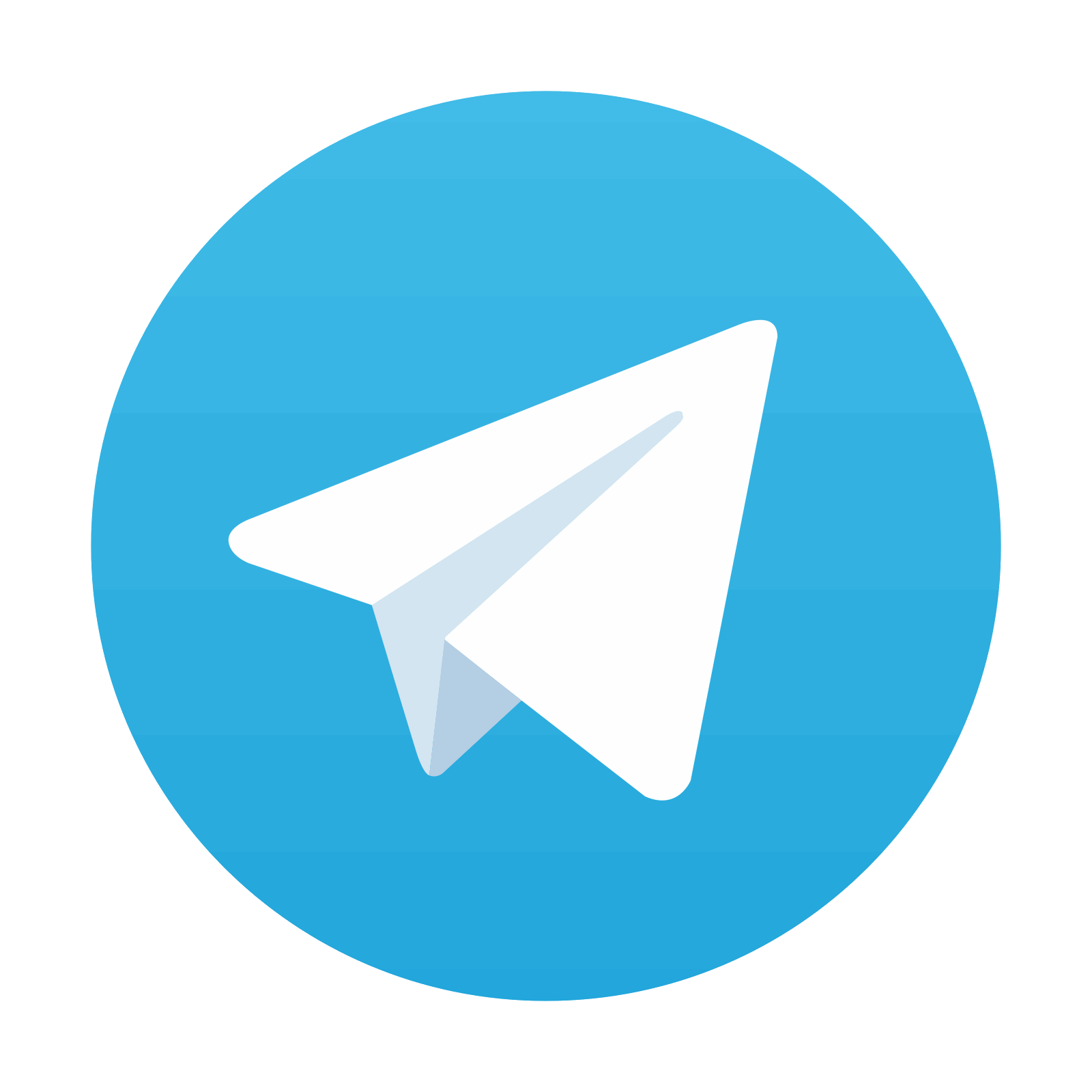
Stay updated, free articles. Join our Telegram channel

Full access? Get Clinical Tree
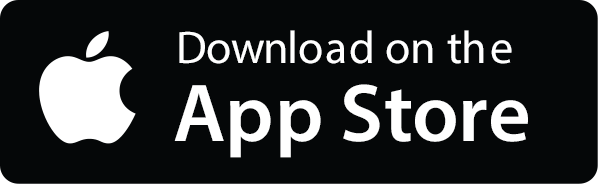
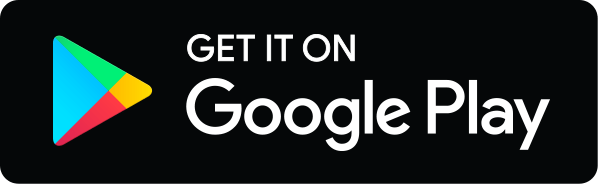