Case 38 A 65-year-old woman presented with persistent painless left-sided blindness which began 2 hours prior to her admission. The referring ophthalmologist observed a retinal opacity during fundoscopy examination, suspected a central retinal artery occlusion (CRAO), and immediately transferred the patient to our department for evaluation of thrombolysis indication. She had a long history of atrial fibrillation and took an oral anticoagulant (phenprocoumon) for primary stroke prevention. Her additional vascular risk factors were arterial hypertension, diabetes mellitus, hypercholesterolemia, and coronary heart disease. Cranial CT revealed old subcortical lacunar basal ganglia lesions bilaterally (not shown). The patient’s INR on admission was 1.6 and her erythrocyte sedimentation rate (ESR), Westergren, was normal (15 mm/first hour). Right-sided CRAO presumably of cardioembolic cause. B-mode imaging revealed moderate atherosclerotic plaques without obvious lumen narrowing in both carotid arteries. Doppler spectrum analysis of the carotid and vertebral arteries was normal (not shown). The STeA and axillary arteries (AxA) showed bilateral normal B-mode and color-mode images and did not show signs of vasculitis. No halo sign was present. All detectable flow signals were normal (not shown). All intracranial basal cerebral arteries of the anterior and posterior circulation showed normal flow signals during transtemporal and transforaminal insonation (not shown). Transorbital color-coded duplex sonography (TOCS) was started after reducing insonation energy below a mechanical index (MI) of 0.26. Normal flow signals were noted in both OAs using a 2-MHz phased-array probe. Utilizing a 7-MHz linear probe, the CRA and accompanying central retinal vein (CRV) were detected. Normal flow signals were observed on the right side. On the left side, however, only the CRV was visualized. A small echogenic spot was observed in projection of the CRAs vessel sheath (Fig. B38.1). Right-sided CRAO presumably of cardioembolic cause. Given an INR of 1.6 and exclusion of other possible contraindications, intravenous thrombolysis with 60 mg recombinant tissue plasminogen activator (IV rt-PA) was administered 3 hours after the onset of blindness. After treatment she reported a returning ability to discriminate between light and dark, but no amelioration of visual acuity. Cardioembolism was assumed and oral anticoagulation was restarted using dabigatran. MRI revealed ~35 small ischemic lesions on diffusion-weighted imaging, predominantly affecting cortical locations (Fig. B38.2). Fig. B38.1 TOCS. Top: Left-sided insonation. Left: B-mode image, linear probe. Note the bright echogenic spot in projection to the CRA (“spot sign”) reflecting embolic vessel occlusion (arrowhead). Middle: Missing color signal of the CRA but also of the CRV. Note the prominent ciliary vessels, in part maintaining retinal perfusion. Right (transcranial probe): Normal antegrade left OA flow (flow velocity 26/9 cm/s). Bottom: Right-sided insonation. Left: B-mode image, linear probe: Normal appearance without spot sign. Middle: Clear blue- and red-coded vessel signal within the optic nerve indicating patency of the right CRA and corresponding vein (arrows). Right (transcranial probe): Normal OA flow, comparable to the CRA occlusion side. Note, a normal OA flow does not exclude CRA occlusion (flow velocity 28/10 cm/s). Fig. B38.2 MRI, diffusion-weighted images, axial plane. Multilocular fresh ischemic infra-and supratentorial lesions in the anterior and posterior circulation mainly in the cortical areas indicative of an embolic shower unnoticed by the patient. Upon reassessment 3 months later, her visual function had not shown any further improvement. Unchanged findings were seen in all intracranial and intraorbital arteries; in particular, the left CRA remained without flow signal and the spot sign was still present. Permanent CRAO probably of cardioembolic origin with additional nonsymptomatic multilocular brain microemboli. This 65-year-old woman had acute painless monocular blindness due to CRAO. CRAO is an ophthalmologic emergency which corresponds to an ischemic stroke to the brain (Biousse 2014). It manifests with a sudden monocular loss of vision. Since this phenomenon was first described by Graefe in 1859 (von Graefe 1859) its therapy has been intensely debated. The incidence of acute CRAO is ~2/100,000. In a population-based Korean study, 3,464 patients were identified between the years 2008 and 2011, resulting in a calculated incidence of 1.8 per 100,000. Men are more frequently affected than women (2.15 versus 1.47). Similarly to ischemic stroke, the incidence increases exponentially with age, reaching 10.08 per 100,000 person years in the age group 80–84 years (men 14.65, women 8.00) (S.J. Park et al 2014). Similar data were reported for the white American population of the United States with incidences of 1.9 per 100,000 (Leavitt et al 2011). More than 95% of CRAOs are caused by noninflammatory vascular conditions, and the remaining cases occur within the context of GCA. The common vascular risk factors are also the primary risk factors for CRAO (Hayreh et al 2009). In patients <50 years old, rare conditions like factor V Leiden prothrombin mutation, protein S and protein C deficiency, AT-III deficiency, antiphospholipid antibodies, sickle cell anemia, and paraneoplastic syndromes have to be considered as supporting factors (Chen and Lee 2008). Specific ophthalmologic risk factors include raised internal eye pressure, optic nerve drusen, and preretinal vessel loops, which all potentially lead to reduced perfusion pressure at the optic nerve head (Brown et al 1979, 1981). CRAO is conventionally diagnosed by ophthalmologic fundoscopy. Within the first 7 days after symptom onset, the following findings can be seen: cherry-red spot (90%), retinal opacity in the posterior pole (58%), retinal arterial attenuation (32%), cattle trucking (19%), optic disk edema (22%), and pallor (39%). Thereafter, optic nerve atrophy (91%), rarefication of retinal arteries (58%), and the development of cilioretinal collaterals can be observed. Naturally, CRAO with sparing of the cilioretinal artery shows less pathologic alteration of the macula and the optic nerve head (Hayreh and Zimmerman 2007). Because the vascular risk factor profiles are identical, CRAO patients have a higher general risk of ischemic stroke (Chang et al 2012). Interestingly, patients with isolated transient monocular blindness (amaurosis fugax) and carotid stenosis seem to have a lower risk of ipsilateral ischemic stroke than patients with a hemispheric TIA. The general risk of ipsilateral stroke recurrence in patients with retinal ischemia might be due to differences in plaque vulnerability. Histologic studies of plaque material following carotid endarterectomy (CEA) have shown that plaques from cerebral ischemia patients contain significantly higher proportions of lipids, inflammation, and plaque instability than those in patients with retinal ischemia (Howard et al 2013). Only in the presence of other factors like age >75 years, male gender, history of stroke/TIA, ICA stenosis grade 80–94%, and peripheral artery occlusive disease is the recurrence risk for ipsilateral stroke increased. The 3-year risk of ischemic stroke under secondary prevention was 1.8% in the group with 0–1 of the above risk factors, 12.3% in those with 2 risk factors, and 24.2% in patients with 3 or more risk factors. CEA led to a 3-year absolute risk reduction for ipsilateral strokes by 2.2% in the group with 0–1 risk factors, 4.9% in those with 2 risk factors, and 14.3% in patients with 3 or more risk factors (Benavente et al 2001). The typical clinical manifestation of CRAO is sudden, dramatic, painless, monocular visual acuity reduction, and 80% of patients have residual visual acuity of ≤5% (Hayreh and Zimmerman 2005). CRAO can be divided into four subgroups: A spontaneous improvement in visual acuity and visual field may occur within the first days after CRAO. A relevant improvement of visual acuity in spontaneous recanalization is, however, only observed in up to 10% of cases (Atebara et al 1995). The majority of patients remain with a visual acuity of 10/100 or worse. The extent of recovery depends on the occlusion type, duration of the occlusion, and the related impairment of retinal perfusion as well as on the “retinal ischemic tolerance time” (Hayreh and Zimmerman 2005). Animal experiments in apes indicate that ischemia durations of up to 97 minutes could be tolerated without residual retinal damage. At 105–240 minutes different patterns of retinal damage were observed, while durations of >240 minutes led to complete or nearly complete optic nerve atrophy as well as severe and irreversible retinal destruction (Hayreh et al 2004). If patients reach the hospital within this time frame, therapeutic approaches may vary greatly, specifically because there is no consensus concerning the management of acute CRAO (Biousse et al 2007). In general, noninvasive and invasive approaches can be distinguished. Standard therapy contains medication with antiplatelet drugs, anticoagulation with heparin, sublingual application of nitroglycerin, systemic application of pentoxifylline, carbogen gas or hyperbaric oxygen inhalation—all aiming to improve oxygen transport via vessel dilation. Massaging the optic bulb purportedly promotes mechanic thrombus resolution. Intravenous application of acetazolamide and mannitol combined with a paracentesis of the anterior optic chamber may reduce the intraocular pressure and raise perfusion pressure in the CRA. Methylprednisolone has also been used in the past to reduce edema (Atebara et al 1995, Deutsch et al 1983, Harino et al 1995, Rassam et al 1993, Rumelt and Brown 2003). All of the standard therapeutic approaches mentioned above have been applied as mono- or combined therapies and are reported, on average, to improve vision in 15–21% of patients (Fraser and Adams 2009, Schumacher et al 2010). However, considering all available contradictory data, a significant alteration of the natural CRAO course cannot be achieved with any of the standard therapeutic approaches (Atebara et al 1995, Karjalainen 1971, Margo and Mack 1996, Mueller et al 2003). Analogous to ischemic stroke, thrombolysis can also be used as a CRAO treatment. Small, mostly retrospective, case series show a clinical benefit of intra-arterial thrombolysis with visual improvements achievable in 60–70% of cases, especially if treatment is started within the first 4 hours after symptom onset (Aldrich et al 2008, Arnold et al 2005, Biousse et al 2007, Richard et al 1999, D.P. Schmidt et al 2002, Schumacher et al 1993, Weber et al 1998). Subsequently, EAGLE (European Assessment Group for Lysis in the Eye), a larger randomized trial including 84 patients, was initiated. In this study patients were treated either by intra-arterial thrombolysis within 20 hours of symptom onset or by conventional standard therapy. The mean onset time was 13.8 hours in the thrombolysis group and 11 hours in controls. The results were disappointing, in that no difference was observed between the intra-arterial thrombolysis group (60% visual acuity improvements) and the conventional treatment groups (57.1% improvement). Surprisingly, the results in the control group contradicted historic data on recovery rates. In addition, the complication rates were significantly higher in the thrombolysis group (37% versus 4.3%) (Schumacher et al 2010). The main problem of the trial was, however, that performing local arterial thrombolysis was logistically difficult, which effectively led to relevant time delays in the onset of treatment. In contrast, the logistical effort and time delay using systemic thrombolysis is much lower. However, convincing data concerning the effectiveness are missing. In a small randomized study, 8 patients each were treated either with 0.9 mg/kg IV rt-PA (10% as bolus) within 24 hours after symptom onset or with conventional standard therapy. The latter did not lead to visual acuity improvement (defined as 3 lines or more) in any of the patients. In the thrombolysis group, only the 2 patients (25% of this group) with treatment initiation within 6 hours of symptom onset achieved a relevant visual improvement (Chen et al 2011). Similarly, positive results were obtained in a study that included patients within a 12-hour time window who received a fixed dose of 50 mg rt-PA. Of 28 patients, only those treated within 6.5 hours had clinical improvement (41%), and none of the patients included after 6.5 hours improved (0%) (Hattenbach et al 2008). A recent study including 46 patients with ophthalmologically CRAO distinguished between patients with and without a spot sign in the CRA. The spot sign was considered to present calcified emboli. Of 11 patients treated with intravenous thrombolysis (0.9 mg/kg; 10% bolus) in a median time of 4.25 hours, all 4 patients without the spot sign had significant visual amelioration and restored blood flow, whereas none of the 7 patients who had the spot sign responded clinically and the CRA remained occluded (Nedelmann et al 2015). Considering these data, time to treatment seems to be a relevant factor for CRAO patients with regard to good clinical outcome (“time is eyesight”). Currently, systemic thrombolysis in evident CRAO should be performed within 4 hours. Like brain vessel disease, partial occlusion, partial recanalization, and residual flow might occur, which may increase the retinal ischemic tolerance. Therefore, intervention within 6 hours still seems indicated and even therapy up to 12 hours might be considered in individual patients without the spot sign and after ruling out vasculitis. In all circumstances, pretreatment cranial CT needs to be performed to exclude relevant recent silent embolic brain infarction—a contraindication for thrombolysis. High complication rates (roughly 10% had bleeds in the two randomized trials mentioned earlier) are, however, most likely due to long time windows (20 and 24 hours) (Chen et al 2011, Schumacher et al 2010). Thus, controlled trials aiming to assess CRAO thrombolysis within a 6-hour time window would be desirable. Finally, information from data registries should be collected to open the way for future controlled trials. In the present case, we began therapy 3 hours after symptom onset. Quick referral to neurology was possible because of pre-existing clinical pathways between our clinic and outpatient ophthalmologists in the region. Thrombolysis was administered as fundoscopy showed a typical CRAO constellation and an emergency cranial CT excluded fresh, silent ischemic brain lesions. Remarkably, the following MRI showed that, in fact, concurrent multiple small embolic infarctions had occurred. MRI results also excluded thrombolysis-induced hemorrhage. The lack of benefit of thrombolysis may be explained by the spot sign. The potential role of ultrasound sonothrombolysis in CRAO is completely unknown. Using duplex ultrasound, the most relevant differential diagnosis of a GCA can easily be assessed and its presence in case of normal STeA und AxA findings as well as a normal ESR can largely be excluded. In our case, the absence of headaches was a relevant clinical indicator against the presence of GCA. In cases of primary neurologic admission with painless monocular blindness, normal ESR, and absence of a halo sign or other signs of vasculitis in STeA and AxA, no fundoscopic or ophthalmologic confirmation is needed. In contrast, if unusual pain and raised ESR are present, immediate high-dose corticosteroids must be administered. For further reading on visual disturbances and stroke, see Case 42. The CRA is a branch of the OA which itself is the first branch of the ICA. Its diameter (0.1 mm) is much smaller than that of the OA (1.0 mm) and the detectable flow velocities are therefore low (Tranquart et al 2003) (for further reading, see Chapter 2, “Central Retinal Artery” under “Special Arterial Anatomy and Ultrasound Anatomy”). The CRA supplies blood to the optic disk and divides into an inferior and a superior main branch. Each superior branch divides into a nasal and a temporal branch, therefore securing the blood supply to all four retinal quadrants. The retinal periphery is additionally supplied by the choroid and ciliary arteries, the latter also originating from the OA. An important anatomic variant is the presence of a cilioretinal artery. A fluorescence angiography study that included 1,000 patients reported that this vessel could be detected in 49.5% of cases (Justice and Lehmann 1976). If present, the cilioretinal artery supplies the papillomacular bundle, which assures a macular blood supply in CRAO and may lead to preservation of the central macular vision. However, another study that analyzed 260 patients, of whom 35 were identified as having a cilioretinal artery, >50% still suffered from a relevant reduction of visual acuity (Hayreh and Zimmerman 2005). This finding is probably explained by large variation in the diameter and territory supplied by the artery. In recent years, ultrasound of the eye has increasingly been used to study acute visual disturbances and retinal ischemia. Orbital color-coded duplex sonography (OCCS) is a simple noninvasive method with great diagnostic and therapeutic potential in diseases associated with raised intracranial pressure (ICP) and vascular diseases involving the eye. High-resolution OCCS can visualize signs of elevated ICP (papilledema, increased optic nerve sheath diameter) and may contribute findings in embolic as well as vasculitic occlusions of retinal vessels. It is widely available, as it can be performed with any of the modern ultrasound systems provided with a linear high-frequency probe. To prevent eye damage, caused by cavitation or heating of sensitive structures like the lens, retina, or choroidea, study times should be as short as possible following the ALARA (“as low as reasonably achievable”) concept. The MI must be kept below 0.26. Using the color-mode window, the CRA is easily identified (for further reading on B-mode pathology, see Chapter 5, “Papilledema and Optic Nerve Sheath Diameter” under “Intracranial Stroke-related B-mode Pathology”). In CRAO, OCCS reveals a missing CRA signal while the CRV signal may be preserved. In embolic occlusions, a hyperechoic B-mode signal can often be detected within the optic nerve head. This is then most likely a cholesterol-rich or calcified embolus (Pfaffenbach and Hollenhorst 1972). Schlachetzki and coworkers coined the term spot sign for the above finding (2010). In an early retrospective study, the spot sign had been observed in 31% of cases with a CRAO. A more recent prospective study analyzed whether the spot sign was useful for differentiating between vasculitic and embolic CRA occlusions. In this study 24 patients with sudden-onset monocular blindness were included after exclusion of nonvascular causes and studied using OCCS. Seven of these patients were diagnosed with a bioptic GCA, none of whom showed a sonographic “spot sign.” Twelve patients had an embolic CRAO, 10 of whom had the spot sign, yielding an overall sensitivity of 83% and specificity of 100% (Ertl et al 2012). In other words, the presence of the spot sign practically excludes the presence of a GCA as an underlying cause of sudden visual loss. In a follow-up study, the patients with a positive spot sign were re-examined with OCCS after an average time interval of 17 months. The sign persisted in all patients, the CRA flow signal was still missing, and, clinically, a permanent loss of vision was observed (Altmann et al 2015). Fibrin-containing emboli are known to have a high rate of spontaneous recanalization while cholesterol-rich and calcified emboli are usually more resistant to therapeutic or endogenous thrombolysis. The persisting spot sign supports the assumption that the majority of emboli were in fact cholesterol-containing or calcified. Therefore, OCCS has the potential to select patients for systemic thrombolysis in CRAO, as presence of the spot sign indicates impaired response to a thrombolytic therapy (Nedelmann et al 2015). Whether the spot sign also permits differentiation between cardiac and artery-to-artery emboli is yet unclear. In our case, the presence of atrial fibrillation and the multilocular brain microembolism in the anterior and posterior circulation argued in favor of cardiogenic embolism. Interestingly, one patient was reported to have a “traveling spot sign.” Clinically, this patient had suffered from two amaurosis fugax events. First, a CRA stenosis with a concomitant spot sign was detected. The second event manifested as clinical worsening which was then determined to result from CRAO with more distal spot sign localization (Nedelmann et al 2014).
Left-sided Amaurosis in Left Central Retinal Artery Occlusion
Clinical Presentation
Initial Neuroradiologic Findings
Suspected Diagnosis
Questions to Answer by Ultrasound Techniques
Initial Neurosonologic Findings
Extracranial Duplex Sonography
Transcranial Duplex Sonography
Transorbital Duplex Sonography
Conclusion
Clinical Course (1)
Follow-up Neuroradiologic Findings (Day 3)
Clinical Course (2)
Follow-up Neurosonologic Findings (3 Months)
Transorbital Duplex Sonography
Final Diagnosis
Discussion
Clinical Aspects
Angiologic and Anatomic Aspects
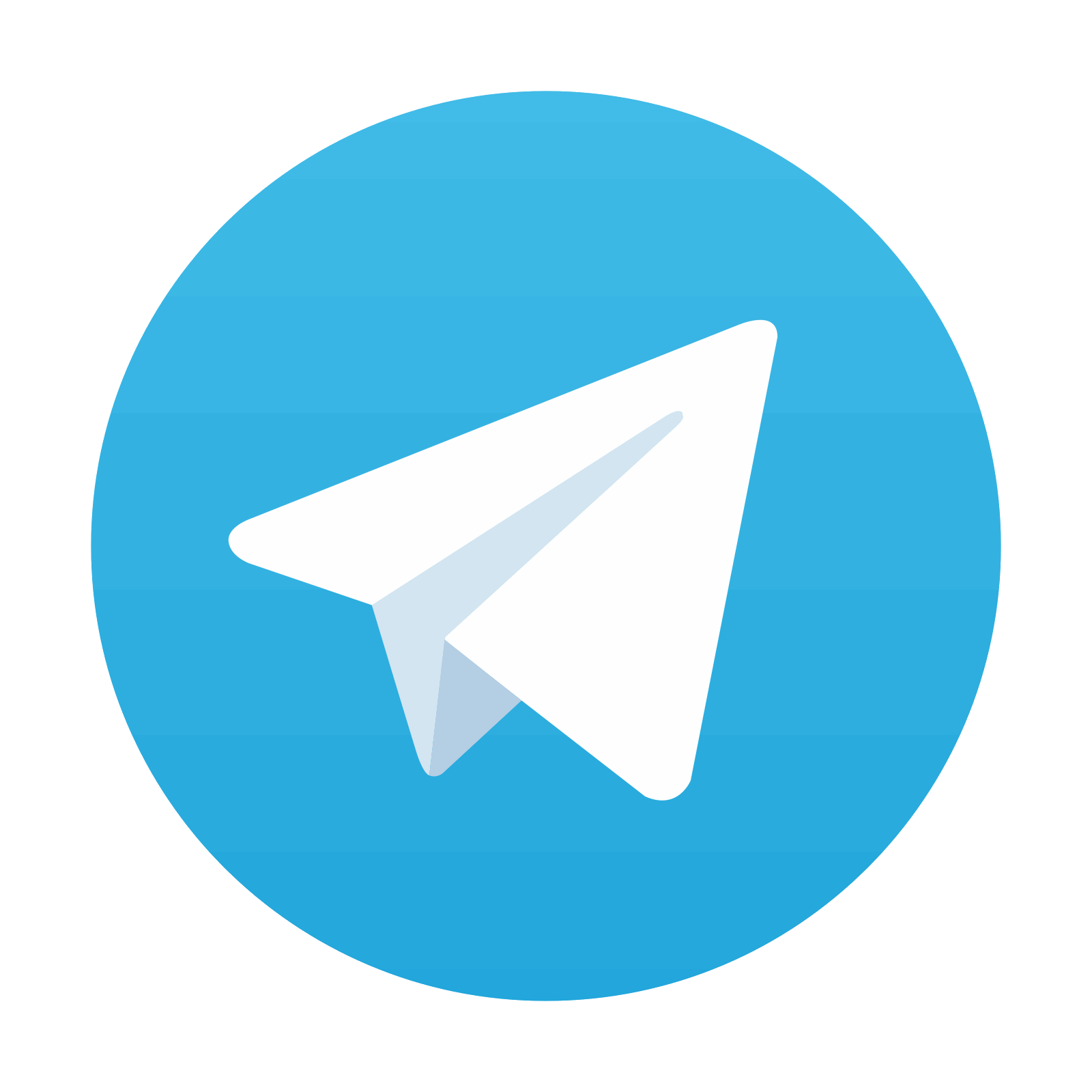
Stay updated, free articles. Join our Telegram channel

Full access? Get Clinical Tree
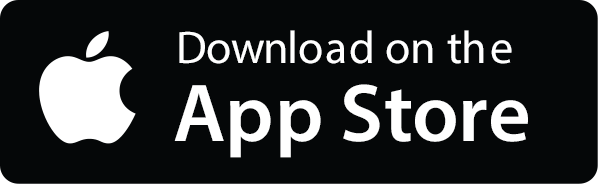
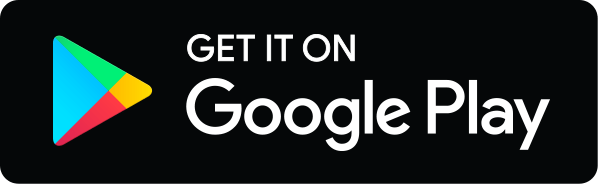