39 New Techniques in Electrophysiological Assessment of Pediatric Epilepsy
In pediatric epilepsy, neocortical seizures are more common compared with adult epilepsy. Epilepsy monitoring with subdural grid and depth electrodes has been used in pediatric epilepsy surgery to delineate the epileptogenic zone and provide valuable insights into the underlying mechanisms of the epileptogenic network in children with intractable epilepsy.
This chapter presents full-band electroencephalography (EEG), ranging from high-frequency oscillations (HFOs) to infraslow EEG (very slow EEG response or direct current [DC] potential shifts), and including EEG source localization (ESL) and nonlinear analysis techniques of seizure prediction by detecting neural network changes in simplicity or complexity. We discuss their roles in improving our understanding of the complex epileptic networks in children.
Conventional analog EEG consists of five bands between 0.5 Hz and 80 Hz: δ <4 Hz, θ <8 Hz, α <13 Hz, β <30 to 40 Hz, and γ <80 Hz. Previously limited by EEG data storage capacity, low sampling rate, and low frequency filtration at the amplifier level, the ends of the EEG spectrum comprising HFOs (> 80 Hz) and infraslow EEG (< 0.5 Hz) now lie within current technological capability and are revealing valuable information. Advanced digital EEG has become able to analyze full range of brain activity from scalp to intracranial recordings.
Full Band EEG
HFOs
Recent advances in digital EEG technology have enabled us to record HFOs > 80 Hz with high sampling rates of 1 to 10 kHz. HFOs above γ frequency are generally termed ripples(80–250 Hz) and fast ripples(≥ 250 Hz) based on their frequency ranges.
HFOs in the brain can be physiological or epileptic.1 Gamma activities and ripples have been noted in the normal and epileptogenic brain; however, fast ripples have been recorded specially in the epileptogenic brain.2 Examples of physiological HFOs include cognitive γ oscillations of 30 to 70 Hz,3 40 to 200 Hz activity in the visual cortex,4 and 500 to 600 Hz in somatosensory evoked potentials.5 HFOs can be recorded intracranial EEG at the onset of focal epileptic seizures.6–10 Power spectral analyses show that the location of HFOs at seizure onset indicate the ictal onset zone.6–9,11 HFOs are also recorded in interictal EEG in epileptic patients.2,12–15 Therefore, HFOs have drawn attention in the field of epilepsy surgery.
Recording HFOs
Recording fast ripples up to 500 Hz requires an EEG sampling rate ≥ 1 kHz according to the Nyquist theorem. Monitors to display EEG waveforms need to have high enough resolution to show recorded HFOs properly. Scalp EEG has recorded HFOs up to 120 Hz using a 500 Hz sampling rate for epileptic spasms in children.16,17 Most studies on HFOs have been done with intracranial macro- and microelectrodes. The electrodes must be placed very close to the area generating HFOs, otherwise HFOs may not be recorded because of focality of the HFOs.
Analysis of HFOs
HFOs analysis encompasses visual and computer evaluation. Visual analysis of HFOs requires high-pass filtering at high frequencies (e.g., 100 Hz) and temporal expansion with high sweep speeds (e.g., 100 mm/s). This filtering and expanding waveform can realize superimposed HFOs in the interictal and ictal discharges.10,15
In computer analysis, time-frequency analyses have been widely used. These analyses transform a one-dimensional signal in the time domain into a two-dimensional domain of both time and frequency. Short-time Fourier transform and wavelet transform are common methods. The authors apply multiple band frequency analysis (MBFA) to demonstrate the rapidly changing property of HFOs in time-frequency domains in detail.
Short-time Fourier transform is a well-known method; however, frequency resolution (frequency band width in which the power spectrum is calculated) depends on time resolution (time window width for power spectrum calculation), and there is a trade off between these resolutions.18,19 To achieve high time resolution to reveal rapid spectral changes of HFOs, frequency resolution has to be sacrificed.
Wavelet transform was developed to capture rapid spectral changes of nonstationary signals.18,19 In wavelet transform, time resolution is high and frequency resolution is lowin analysis of high frequencies. The relationship is reversed at lower frequencies, with low time resolution and high frequency resolution. Wavelet transform is useful in detecting changes in power spectra of HFOs; however it is difficult to investigate changes in frequency in detail, particularly at higher frequencies.
MBFA was developed to analyze rapid spectral changes of evoked potentials over time with high frequency and time resolutions.20 MBFA decomposes the raw signal into component frequency bands by applying multiple sequential band filters. Application of time windows divides the component frequency bands into chronologically sequential segments for which the power values can be calculated and demonstrated graphically. In MBFA, frequency and time resolutions are fixed for all frequency bands, similar to the short-time Fourier transform.
HFOs in Interictal Periods
Bragin et al2 used microelectrodes to record ripples at 100 to 200 Hz and fast ripples at 200 to 500 Hz in hippocampus and entorhinal cortex in patients with mesial temporal lobe epilepsy. Staba et al13 reported interictal HFOs within the bandwidth of 80 to 500 Hz in hippocampus and entorhinal cortex of patients with mesial temporal lobe epilepsy using depth electrodes. The hippocampus and entorhinal cortex ipsilateral to seizure onset showed a higher ratio of the number of fast ripples to ripples compared with the contralateral side. Urrestarazu et al14,15 also demonstrated interictal 100 to 500 Hz HFOs recorded from depth electrodes in focal epilepsy. Their results indicated that in temporal lobe epilepsy, fast ripples were more restricted than ripples to the electrodes located within the seizure onset zone, especially to the hippocampus.15
HFOs in Ictal Periods
Allen et al6 found 80 to 115 Hz HFOs at the onset of frontal lobe seizures. Fisher et al7 reported a twofold increase in the 40 to 50 Hz range and up to a fivefold increase in the 80 to 120 Hz portion of power spectrum at seizure onset in patients with various etiologies. Traub et al8 reported ictal HFOs up to 130 Hz in children with epilepsy secondary to cortical dysplasia. Jirsch et al10 demonstrated well-localized, segmental, fast ripples at 250 to 500 Hz in mesial temporal lobe seizures. Akiyama et al21 reported the presence of 60 to 150 Hz HFOs during epileptic spasms of focal origin ( Fig. 39.1 ). Ochi et al22 demonstrated HFOs up to 250 Hz in pediatric focal neocortical seizures.
Propagation of HFOs during Seizures
Ictal HFOs had different patterns and distributions among seizures in pediatric neocortical epilepsy. Ochi et al22 analyzed spatiotemporal dynamic changes of ictal HFOs by MBFA and compared the distribution and frequency of ictal HFOs with the postsurgical seizure outcome. When HFOs early in the course of a clinical seizure were confined to the ictal onset zone, resection of this zone stopped seizures. However, when the predominant HFO zone extended beyond the resection margins or the area outside resection margins recorded higher frequency HFOs than resection area, seizures continued postoperatively.
Topographic HFO Movie
Visualization of HFOs on the brain surface picture enhances the understanding of the relationship between their topographic distribution and anatomical structures. Akiyama et al23 developed a movie mapping technique to demonstrate power changes of ictal HFOs over time during neocortical epileptic seizures. They identified the ictal HFO frequency range and averaged the HFO power values within the frequency bands at each electrode. Topographic maps of HFO power values were superimposed onto a picture of the brain surface. These maps were integrated into a movie file to animate the spatiotemporal changes of ictal HFO power over the grid electrodes correlating with progression of seizures.

HFOs and Muscle Activity
During seizures, intracranial EEG electrodes can record HFOs of extracranial origin caused by ictal muscle movements. Ot-subo et al24 reported confounding of intracranial HFOs during mesial temporal lobe seizures in a patient with recurrent seizures. Power spectral analysis of HFOs using MBFA demonstrated differences in spatial distribution and frequency properties between HFOs originating from the brain and muscles. Muscle HFOs were observed at fixed electrodes close to temporal muscles and were of random scattered frequency bands, whereas ictal epileptic HFOs showed a sustained, high-frequency band in the ictal onset zone and subsequently propagated to other areas through the epileptic network. This phenomenon of extracranial HFOs recorded in intracranial EEG electrodes was termed a reverse breach rhythm, compared with a regular breach rhythm in which Intracerebral discharges appeared at high amplitude through the skull defect.
Infraslow EEG
Traditionally dismissed as artifacts and actively filtered out, infraslow EEG activity (often referred to as DC-EEG) has been recognized in association with seizure activity as far back as 1963.25 DC-EEG implies a frequency of 0 Hz, but in reality infraslow EEG consists of extremely low-frequency signal of < 0.5 Hz.26
Interest in this infraslow EEG has waxed and waned27 because the data collection was hampered by the need of specific equipment. Unlike HFOs, which can be measured by most commercially available EEG recording equipment with a sufficiently high sampling rate and storage capacity, infraslow frequencies require DC-compatible amplifiers with nonpolarizable Ag-AgCl electrodes and stable gel-electrode interfaces. In addition, skin preparation is required to eliminate skin potentials by scraping or puncturing the skin with microneedles.28
Visual interpretation of infraslow EEG is complicated by the masking effect of higher frequency activity and difficulty in differentiating between genuine potential changes, artifacts, and surface potential changes related to the blood—brain barrier and cerebral blood flow.29–31 Applications of infraslow EEG include study of the preterm neonatal brain,32 sleep,33 cognition and motor activity,34 and seizures. Initial studies on seizures used invasive monitoring in animals and humans and has demonstrated infraslow activity during 3 Hz generalized spike-and-wave discharges,35,36 temporal lobe seizures in humans and monkeys,37 and focal epilepsy.38 Noninvasive recordings of 35 seizures in seven adult patients with temporal lobe epilepsy provided lateralizing information consistent with conventional scalp EEG in all and intracranial EEG in four patients.39 In both temporal and extratemporal lobe epilepsies, infraslow EEG analysis with source localization gave independent localization data in 6 of 30 patients. Source analysis was done in adult patients who had single epileptic foci and no skull breaches. Surgery was performed in five, all with temporal lobe epilepsy.40 Further study of the utility of infraslow EEG in neocortical epilepsy and in pediatric subjects is desirable.
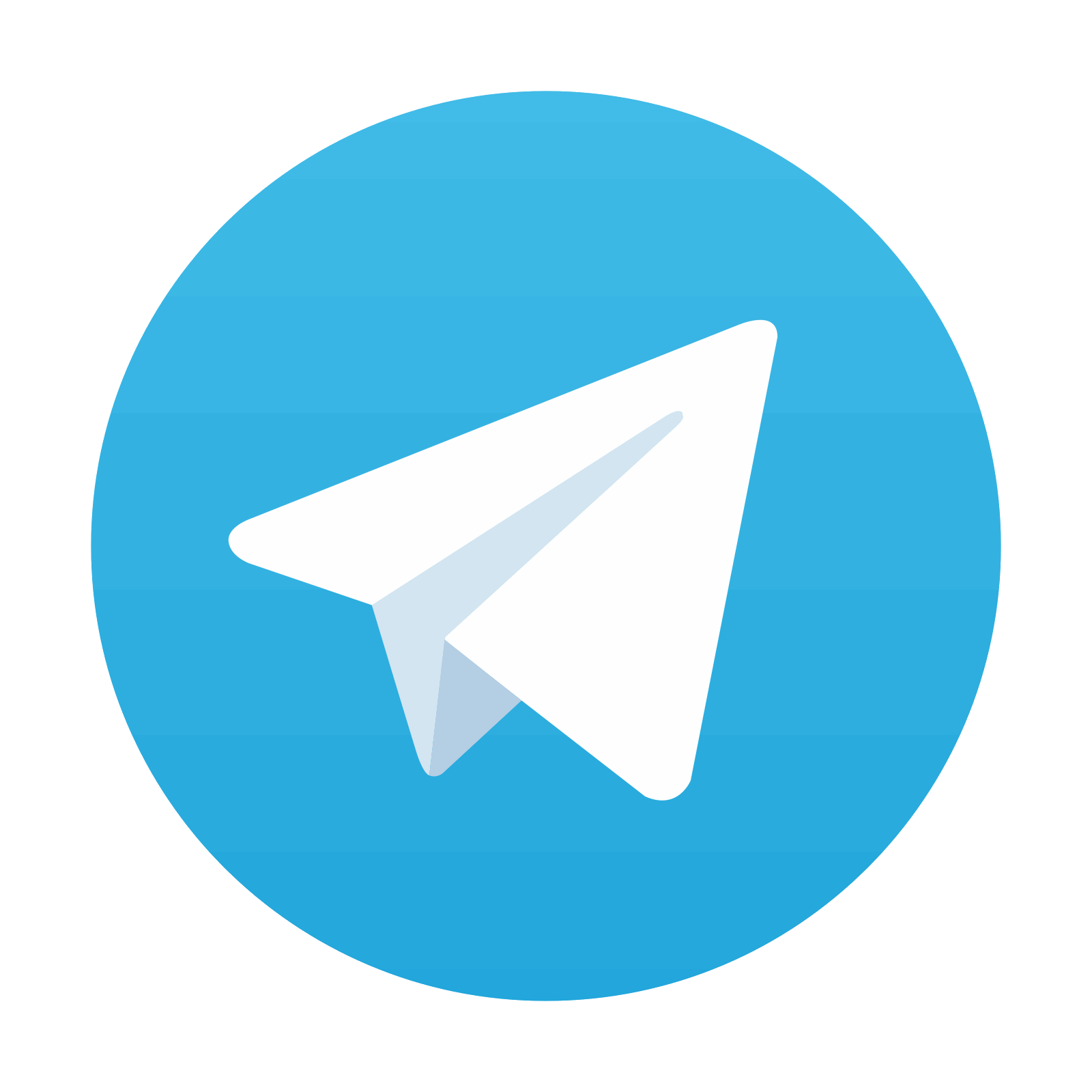
Stay updated, free articles. Join our Telegram channel

Full access? Get Clinical Tree
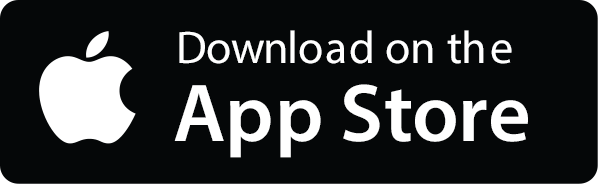
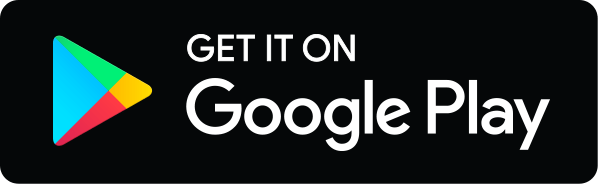