4 Posterior Interbody Cages
4.1 Introduction
4.1.1 Interbody Cage Overview
Interbody cage technology has continued to evolve since its first use in spinal fusion. Initially, autologous bone graft was utilized to induce fusion; however, this was often associated with substantial postoperative complications. 1 , 2 , 3 , 4 , 5 Interbody cages were eventually developed as a standalone construct to improve stability and distraction within the intervertebral space while also promoting bone ingrowth. 6 , 7 As such, interbody cages have allowed for improved fusion rates while decreasing postoperative pain, hospital length of stay, and complication rates. 8 Indications for posterior lumbar interbody cages are provided in ▶ Table 4.1.
A variety of lumbar interbody cages have been developed to maximize the implant’s ability to correct deformity, provide mechanical stability, and provide the optimal environment for vertebral arthrodesis. In order to perform this, a cage must exhibit a small material volume in order to maximize the volume for bone graft. 9 Additionally, the interbody cage must exhibit a large footprint to optimize the interface between the prepared vertebral end plates and interbody cage. 10 Finally, the interbody cage should provide restoration of disk height and lordosis along with the restoration of load bearing to the anterior column. 11
4.1.2 Interbody Cage Classification
Interbody Cage Geometry—Standard Cages
Interbody cages are classified by one of three different structures: horizontal cylinders (HCs), vertical rings (VRs), and open boxes (OBs). HCs were one of the first structures to be developed and have been considered successful in correcting deformity and providing stability. 12 However, these interbody cages are not frequently utilized in today’s surgical setting.
A VR is another type of interbody cage. These interbody cages are utilized with anterior and lateral approaches to the lumbar spine. The design of VR cages was initially adapted from the design of femoral cortical rings. 13 This structure of cage has exhibited moderate ability to correct mechanical deformation. Disk height has previously been demonstrated to be maintained well with this implant, typically exhibiting an average loss of 1 mm at 1 year postoperatively. 9 Additionally, the increase in neuroforaminal area is similar to that provided by HCs. 9
Indications |
|

OB cages are the third type of geometry for interbody cages (▶ Fig. 4.1). They are one of the most frequently utilized interbody cage structures, and are often used for minimally invasive transforaminal lumbar interbody fusions (MIS TLIFs). 14 OB cages have exhibited satisfactory outcomes in correcting mechanical deformity, achieving stability, and promoting arthrodesis. 9 With appropriate surgical technique, OB cages can restore disk height and potentially increase neuroforaminal area. 9 Restoration of lumbar lordosis with OB cages has also been demonstrated to be similar to that achieved with HC cages. 14 These cages have recently been developed to exhibit a wedge shape in order to improve lordosis. OB cages have also demonstrated successful fusion rates, likely due to the sufficient volume available for bone graft and wide surface area for contact with the vertebral end plates. 9
Interbody Cage Geometry—Expandable Cages
Expandable interbody cages have recently been developed in attempts to overcome some of the challenges associated with minimally invasive techniques. 15 Due to the narrow surgical access corridor and anatomic positioning of minimally invasive posterior approaches, the interbody cages utilized in these techniques are often limited in size. 15 , 16 These smaller implants reduce the contact surface area between the vertebral end plates and the interbody cage, increasing the risk for subsidence and pseudarthrosis. 16 , 17 , 18
Some expandable interbody cages are capable of expansion in both the medial-lateral and cranial-caudal planes. These attributes may facilitate fusion and reduce the rate of subsidence by increasing the surface area of the vertebral end plate that is in contact with the implant. However, despite these theorized benefits, there remains limited data regarding the clinical efficacy of expandable cages. 15 , 19
Interbody Cage Materials
Two predominant materials are typically utilized for interbody cages: metal and carbon fiber. Titanium is the predominant material utilized for metal cages, and polyetheretherketone (PEEK) is typically utilized in carbon fiber cages. To determine the success of a particular material for use as an interbody cage, the biologic response, biomechanical strength, and radiographic characteristics are typically assessed.
Both titanium and PEEK cages have exhibited favorable outcomes for arthrodesis and biomechanical strength. Due to the material’s strength, titanium cages are thought to have increased stability and reduced micromotion when compared to PEEK cages. 9 However, PEEK cages may exhibit several advantages over titanium cages, including the avoidance of metal allergies, radiolucency, and reduced artifact on MRI. 20 , 21 , 22 , 23 , 24 These radiographic advantages allow for better analysis of arthrodesis postoperatively. PEEK cages also exhibit moderate stiffness with some elasticity, potentially increasing fusion rates. 21 However, despite these proposed benefits, superiority of PEEK cages over titanium cages remains controversial. 25 Previous studies have demonstrated varying results, with similar fusion rates between titanium and PEEK cages. 25
4.1.3 Minimally Invasive Transforaminal Lumbar Interbody Fusion
Since its introduction by Holly et al, 26 MIS TLIF has been demonstrated to reduce blood loss, postoperative pain, and length of hospital stay when compared to traditional open posterior techniques. 27 , 28 , 29 , 30 , 31 , 32 As such, MIS TLIF has been increasingly utilized for the treatment of degenerative lumbar disease, trauma, and deformity. However, this technique requires surgeon experience with a variety of MIS instrumentation as well as comfortability with the approach.
4.2 Static Carbon Fiber Interbody Cages
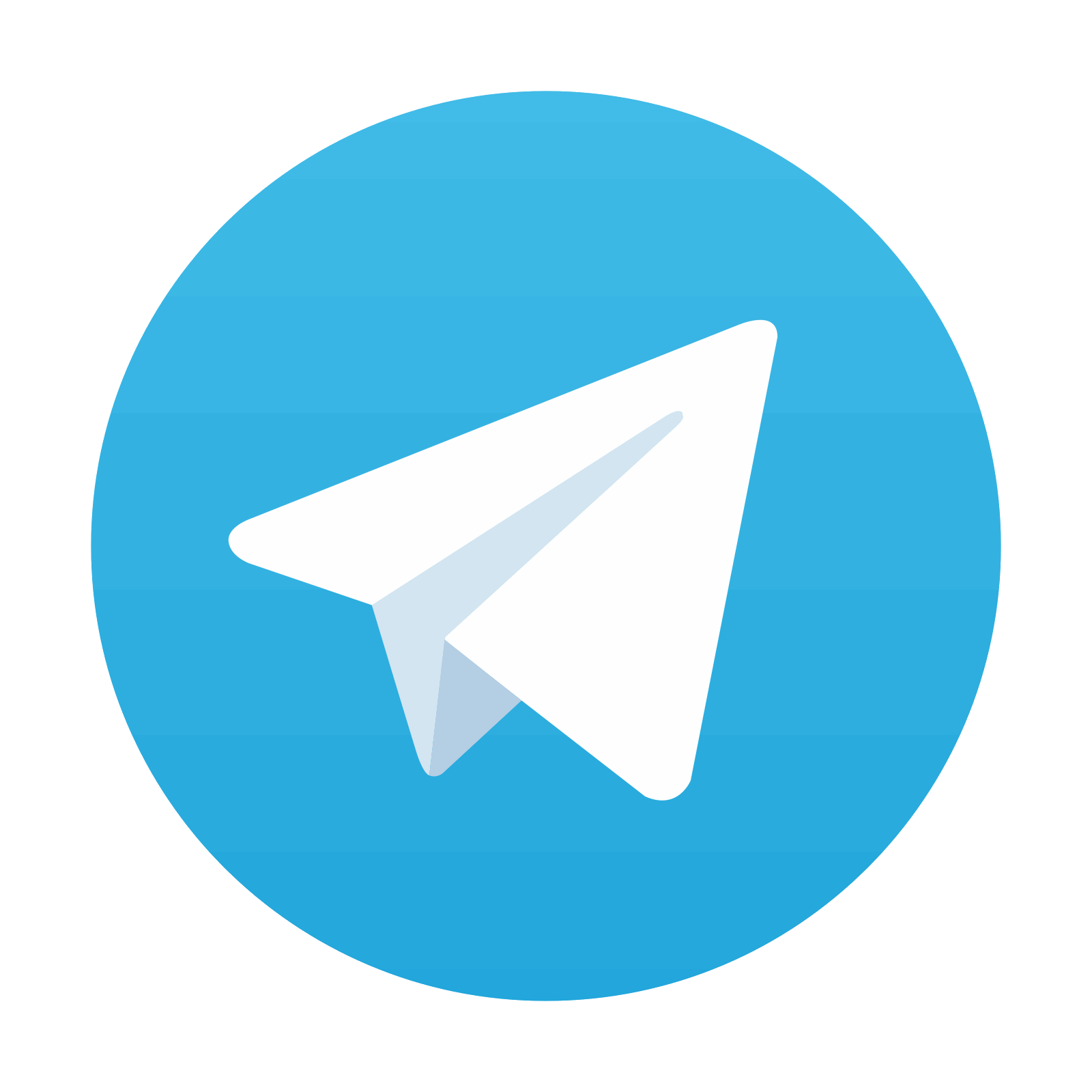
Stay updated, free articles. Join our Telegram channel

Full access? Get Clinical Tree
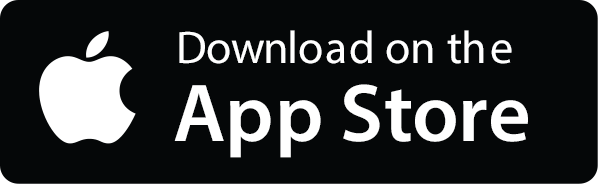
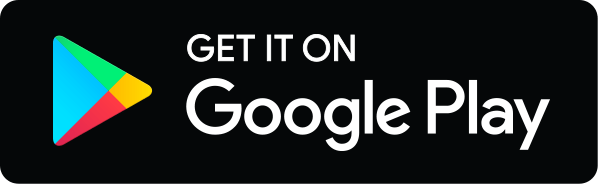