6 Functional Networks of the Human Cerebrum
6.1 Introduction
In this chapter, I take the ambitious step of trying to cobble connectomic data into a coherent model of human brain networks. It should be acknowledged that by doing so, I am putting out some data which may need to be revised in the future. Quite simply, we are still working a lot of these data out.
Despite the challenges of working with incomplete data to try to create a world view of how higher brain functions, there are some major potential rewards. 1 , 2 Presently, we do surgery on the brain without more than cursory models of how the brain works, many of which date from decades ago which predate MRI let along functional imaging. It is almost certain that an incomplete anatomic model is better than no real model. Further, while functional MRI and other types of brain mapping have provided some insight into how some of these functions are organized, often the exact anatomy is imprecisely defined and the exact connectivity is poorly understood. Knowing that the function is grossly located in the lateral frontal lobe may work in research, but millimeterscount in surgery.
The goal of this chapter is to provide an advanced anatomic framework of the gross cerebral anatomy underlying specific higher brain functions from which to base any further discussion on how to preserve cerebral functions in surgery. You can’t validate or invalidate an idea with no clear hypothesis, and you can’t build a model without attempting to synthesize existing data into coherent frameworks. This chapter is the first that I know of to try to demonstrate the anatomy of higher brain functions in a surgically and anatomically useful framework, and hopefully, it will be the beginning of a long future of critically revising and refining these models.
6.2 Methods and Assumptions
If you look at maps which attempt to summarize every single connection in a network, such as the motor network, the complexity is stunning, and it strikes you that we will never be able to meaningfully use something so complex in daily practice. 1 , 2 Further, it is likely that almost every part of the cerebrum has at least some connections at a microscopic level with almost every other one. Despite this, we as neurosurgeons know from experience, that you can make a significant transgression without destroying observable brain functions in any tragic manner, so it is certain that many of these connections are redundant, or at least compensable. It is likely that there are no individual neuron or small collections of neurons anywhere in the brain that cannot be cut without causing a deficit, assuming that other similar neurons remain to compensate for its loss.
These networks do not strive to account for every possible connection in the human cerebrum between sites of relevance, nor do they attempt to include every area of the brain which has been noted to be activated in one study or another. It is probable that the basal ganglia, basal forebrain, the ascending seratoneric system, and or the cerebellum could probably be put somehow by someone into every single one these circuits based on some study, again, we have to cut somewhere, and we want to focus on what is necessary or central to maintaining the brain function of interest. There are no bonus points for adhering to strict interpretations of connectionism, and what matters is whether the patient can do the function you aim to preserve after the surgery or not.
We have grounded all of our work with connectomics in the Human Connectome Parcellation scheme. It is not the only way to divide up the human cortex into segments; however, it is based on extremely high-quality data, and it is a sensible, data-driven scheme which correlates many previous schemes in addition to identifying new regions. Examples of the parcellations can be seen in the figures in Chapter 3; however, thoroughly describing these areas is beyond the scope of this book and would require a book of its own.
With the idea that these models are aimed at clinically useable as opposed to comprehensive maps of brain function, I went about making these maps in the following manner:
We utilized neuropsychologic and functional imaging literature paired with my own experiences with awake brain surgery to create a basic idea about what the networks should roughly entail.
We performed coordinate meta-analysis to determine statistical locations of activation in a standardized coordinate space. This techniques allows one to combine the published coordinates from the fMRI and lesion literature to make a statistical map of significant sites of brain actuation.
We converted the Human Connectome project parcellation scheme to create ROIs of the parcellations in this coordinate space. We then matched these ROIs to the activation estimates yielded by coordinate based meta-analysis to figure out which areas were being activated according to these studies.
We then utilized tractography to identify which fibers, if any, joined these areas. This creates a backbone for the network. It is important to note that the term backbone does not imply that we have identified all possible sites involved in the network. It is certain that the creation of higher human abilities involve interactions between networks. For example, interactions between visual, semantic, and face motor systems are necessary to name objects.
6.2.1 The Motor Network
(Fig. 6.1, Fig. 6.2, Fig. 6.3)







This system is the most familiar to neurosurgeons, as we have been making sure patients were “moving all four” for our entire careers. Thinking in terms of basic motor cortex issues is not foreign or novel to us; however, I would argue that most of us have a relatively overly simplistic view of the motor system, which overestimates how great a patient who is “moving all four” is really doing from a motor standpoint, and in some cases, puts our goal to use all four extremities at serious risk. In short, you cannot leave motor strip, disconnected from the rest of the network, floating in the air, and expect it to move the hand. The failure to apply these facts has been at the root of many episodes where the arm doesn’t work and no one can quite figure out why.
We discuss the motor system first because it is the center piece of many of the other networks, as motor output is essential for many of these networks to manifest that they are working. It is probable that most core neurofunctional units of the cerebrum must access the motor system at some point to make a clinically observable output, however indirect that may be. For most systems below the sublime intellectual and the limbic, this is a short and direct path, so it’s valuable to know this system in detail before moving on.
The Major Players
The Primary Motor Cortex (M1)
For most neurosurgeons, the motor system is synonymous with M1, the corticospinal tract, and probably if asked, we would throw in the basal ganglia, and the cerebellum. It does not need to be restated here that this gyrus is a critical end effector of motor function as it provides the lion share of corticospinal axons, and its injury is clinically obvious.
What is interesting is that there is evidence that it is not the original motor site during evolution as it comes from a phylogenetically younger lineage than the supplementary motor area (SMA), which also contributes to the corticospinal tract. This is logical given its key role in coordinating complex coordinate movements such as alternating finger movements, which would not be needed in lower animals which hemibody movements may suffice. It also, in my opinion, argues why SMA syndrome in its early stages appears so much worse of a motor problem than motor cortex transgression.
The somatotopic organization of the motor cortex should be quite familiar to anyone planning on doing complex glioma surgery, and I will not belabor this point.
The Supplementary Motor Area (SMA)
This is located in the medial bank of the posterior SFG just anterior to the motor cortex. This area has been known to play a role in motor planning for decades, and although its exact role in this is still being elaborated, I think it’s safe to say it is an important player in motor initiation given how bad SMA syndrome looks at least initially: usually some combination of dense hemiplegia and mutism.
The literature tells us that SMA syndrome improves around 90% of the time in long term follow-up, and in my experience it often does improve, but I think some observations should give us great pause prior to charging in there to get a little bit more tumor out of the SMA areas. First, it has been my experience that recovery is not always complete, i.e., these patients often do not reach a normal baseline either from a motor, speech, or volitional standpoint, and I suspect that in many studies, these patients were counted in the 90% “recovery.” Second, even with that liberal definition, 10% of patients are left hemiplegic and/or mute permanently. Third, recovery probably involves the contralateral motor system taking over, and cutting the corpus callosum fibers can prevent recovery from happening (see Chapter 17). Fourth, and most salient, the idea that the SMA was a part of the brain you could sacrifice and then just ride it out until they recover, comes from the epilepsy world, where resections are usually cortical, and there is no need to do any other treatments on a time schedule. Gliomas are not purely cortical, and the patients need to get to adjuvant treatment in most cases in a time frame much faster than SMA syndrome usually recovers.
In most cases, I would argue that its best to leave it in the network, the amount of increased resection obtain by taking it out is usually very small and doesn’t justify the problems it causes.
The area traditionally viewed as SMA is really four areas: 6 medial anterior (6ma), 6 medial posterior (6mp), superior frontal language area (SFL), and supplementary and cingulate eye field (SCEF).
Premotor Areas (PMA)
These portions of the inferior and middle frontal gyri just anterior to the motor cortex, are the point of access to the motor system for numerous neurofunctional systems, including the speech, praxis, and spatiotemporal systems.
In addition to its well-known role in organizing motor signals to be sent to downstream effectors such as the motor cortex, a common way it interacts with other systems is as a judge which compares and assesses information deciding the relative importance of various streams of information.
The Human Connectome Data suggests that original Brodmanns area 6 on the lateral convexity can really be viewed as 3 clusters:
Dorsal premotor area: 6 anterior (6a) and 6 dorsal (6d) which lie on the posterior SFG near its junction with the motor cortex.
The MFG cluster: these lie in the precentral sulcus near its junction with the MFG and includes the frontal eye field (FEF) and premotor eye fields (PEF) that surround area 55b, which is clearly a new language area.
Ventral premotor area: these include areas 6 rostral (6r), and 6 ventral (6v) which lie in the opercularis portion of the IFG.
Basal Ganglia
The putamen, globus pallidus, subthalamic nucleus and substantia nigra are well known to all as key parts of the motor system. This is not a book about movement disorders, so we will not belabor the details of the direct and indirect pathways of circuitry and their roles in the regulation of smooth movement and movement initiation. Sufficed to say that in general you should not be working between parts of the basal ganglia if your goal is preserve good motor function, though this can be done in specific circumstances which are described in Chapter 14.
More important to the practice of glioma surgery is the observation that the basal ganglia receive input from the motor planning areas, among other areas, and if you undercut the motor planning areas such that you disconnect them from the basal ganglia, it is probable that the motor circuit is significantly disturbed.
Cerebellum, VA/VL Nuclei of the Thalamus
The need for cerebellar input to achieve coordinated and smooth movement is well known, and needs no introduction. Most relevant to the supratentorial brain is the descending corticocerebellar tract, which parallels the corticospinal/corticobulbar fibers, and the ascending inputs which enter the motor unit via the VA/VL thalamic nuclei, which send fibers in the motor system via the thalamic peduncle.
Somatosensory Cortices
For years, I was under the impression that the goal of brain mapping and finding the sensory strip, was to have a clear idea where the motor strip was. It was best, obviously, to avoid the sensory cortex, but at worse the issue would be a numb leg and/or hand. I suspect many still think in these terms today, though if asked on the boards no one would suggest this is a good idea out loud.
The problem with this idea is that the central sulcus is not the Sylvian fissure, a deep aneural cleft, but rather there are dense, bidirectional communications between the motor and sensory cortices. It should not surprise anyone that proprioception and touch are key to useful control of movements, especially for the hand. It makes no sense to preserve movement in a hand which one has no control over and then applaud yourself because they are “moving all four.” Neurosurgery is difficult, and we shouldn’t and can’t dwell on all problems we cause, but at the same time we shouldn’t ignore that they are there and refuse to strive for better results.
This fact highlights a key flaw in our thinking that can only be exposed by careful thought given to the study of brain connectivity. For years, we have strived to be less damaging to the brain, and have devised many techniques to cut less brain to get to a lesion. Exploitation of subarachnoid spaces is one such approach. Using a trans-sulcal approach to minimize brain trajectory length is another. In many cases trans-sulcal approaches make a great deal of sense, but like all dictums, they should not be applied blindly. Remember that the bottom of a sulcus is not like the floor of the third ventricle, it is not aneural but rather it is cortex and transiting U-fibers, and that a trans-sulcal approach down the central sulcus in some cases, may be more damaging than a slightly longer brain path which does not disconnect the sensory and motor cortices from each other. The shortest path is not always the best.
Superior Parietal Lobule and Banks of the Intraparietal Sulcus
As described below, higher level visual processing has generally been described as flowing out from the occipital lobe in two basic streams: The Ventral “what” pathway and the Dorsal “where” pathway. The dorsal stream (“where”) is progressively processed in the precuneus and neighboring occipital lobe before sending its output to the superior and inferior parietal lobules. While these two lobules are both known for spatiotemporal processing, they are quite different functionally, as the inferior parietal lobule is phylogenetically much newer (these cytoarchitectural areas probably do not exist in macaques and lower monkeys), and its damage causes a very different syndrome that superior parietal lobule damage. For this reason, the inferior parietal lobule is included with other networks.
Because the direct input from the visual system to the motor system is at best limited, the superior parietal lobule probably is involved in integrating visual, proprioceptive, and somatosensory inputs and feeding this information forward to the motor system where it helps shape movements. Numerous studies have especially linked the intraparietal sulcus and its banks to maps of personal and extrapersonal space, and their roles in visual guidance shaping of hand movements to conform to targets during reaching, grasping and manipulation.
It seems likely that the superior parietal lobules functions are densely bilaterally connected as its transgression is generally well tolerated, though the phenomenon of optic ataxia, meaning difficulty and inaccuracy moving the hand to visually directed targets should point out the possibility that parts of this gyrus may be important for use of the hand, and we have encountered poor coordination when working in this area before.
The role of the intraparietal sulcus and its banks, which in likelihood is where our spatiotemporal maps of the world are stored, should give us pause before choosing this sulcus as a route to the atrium of the lateral ventricle. This is in addition to the frequent location of the medial parts of the SLF deep to this sulcus in some patients.
6.2.2 The Anatomy of the Network (Fig. 6.1, Fig. 6.2, Fig. 6.3)




Using the SMA as a starting point, the SMA fibers exit the gyrus in three primary bundles. The lateral bundle is the FAT which connects the SMA to the Premotor areas in the posterior portions of the MFG and IFG. The middle bundle descends vertically to contribute to the corticospinal tract, and to communicate with the basal ganglia. The medial bundle enters the corpus callosum, wrapping around the cingulate gyrus and cingulum en route.
The callosal fibers are interesting as they have a mix of homotypic fibers which interconnect the bilateral SMAs, and heterotypic fibers which connect the SMA with the contralateral MFG and IFG (which we call the crossed FAT pathway). I suspect this pathway is necessary for the SMA syndrome to recover (it allows the other side to take over). This is also the principle reason why I think mid-callosal butterfly gliomas are probably still largely inoperable, or at least you can’t get much of them out safely.
The FAT is one of the principle components of the motor planning system as it is the principle way the SMA and PMA communicate, and I suspect it is one way to cause hemiplegia without entering the motor strip, though I have had great respect for it ever since I learned about it.
The motor planning sites communicate through vertical, apically directed small tracts aimed at the basal ganglia. Thus, the descending fibers of the motor system generally begin anterior to the motor cortex. They also have U-fiber connections directly linking the motor planning areas to the motor cortex. The thalamic peduncle carries this information back up to the motor strip.
Sensory information probably enters the motor network through two routes. First, the central sulcus contains U-fiber communications between the primary sensory and motor cortices. In addition, the Superior parietal lobule and banks of the intraparietal sulcus send processed information to the middle frontal gyrus via the medial parts of the SLF.
Ramifications of this Anatomy for Surgery Near the Motor Network
The motor network is more extensive than just the motor strip and its fibers, and thus motor mapping should start well anterior or posterior to the motor strip, and the end point we are looking for ideally should integrate numerous aspects of movement, more than just moving “yes/no.” decisions. We cannot always save proprioceptive function in some cases, but we should base these decisions on facts on the ground, and should think about motor mapping as a study of the overall integration of all aspects of motor function.
The cuts around the motor system are largely coronal and straight cuts which parallel the descending and ascending pathways between the motor cortices and their basal ganglia, thalamic, and cerebellar sources.
Cutting through the medial SLF can have motor ramifications.
If you find that you have entered the body of the lateral ventricle through a lateral approach, you probably have screwed up. There are too many tracts blocking that route. At minimum, you have transgressed the superior thalamic peduncle.
The Two Pathway Networks: Language, Praxis, and Neglect
Conceptual Framework
I have grouped these three networks together as they share remarkable parallels in anatomic organization, the involved cortices, and more interestingly, in the way they process information. Thus, understanding one system provides major insights on how the other ones work.
I have speculated that this similarity comes from the evolution of the semantic areas of the cerebrum namely the posterior temporal lobe and inferior parietal lobule. In short, animal psychology has long postulated that the idea that animal intelligence can be accurately depicted as a fraction of the global human intelligence is inaccurate. In many cognitive domains, animals perform quite close to humans, while others they lack entirely. This suggests that increasing intelligence with evolution was achieved not merely in the increase in cerebral volume, but also by the creation of new brain areas and networks.
While monkeys such as macaques have an inferior parietal lobule and intraparietal sulcus, cytoarchitecturally these structures are better characterized as analogs of Brodmann’s area 7, and thus the supramarginal and angular gyri and posterior temporal lobes are substantially bigger in humans than monkeys. 3 In fact, in addition to the fold increase in the relative size of the prefrontal cortices, this is one of the most conspicuous area of difference with the monkey to human leap. Thus, it is not surprising that a large number of human like brain functions localize strongly to this part of the brain. Interestingly, while it will not surprise you that experimental brain lesioning in macaques has failed to provide an acceptable model for aphasia or apraxia for tool use, it is of interest that the cortical lesioning in macaques is also unable to recreate human hemineglect, as lesioning the parietal lobe merely leads to an optic ataxia like syndrome similar to that seen with lesioning of the superior parietal lobule.
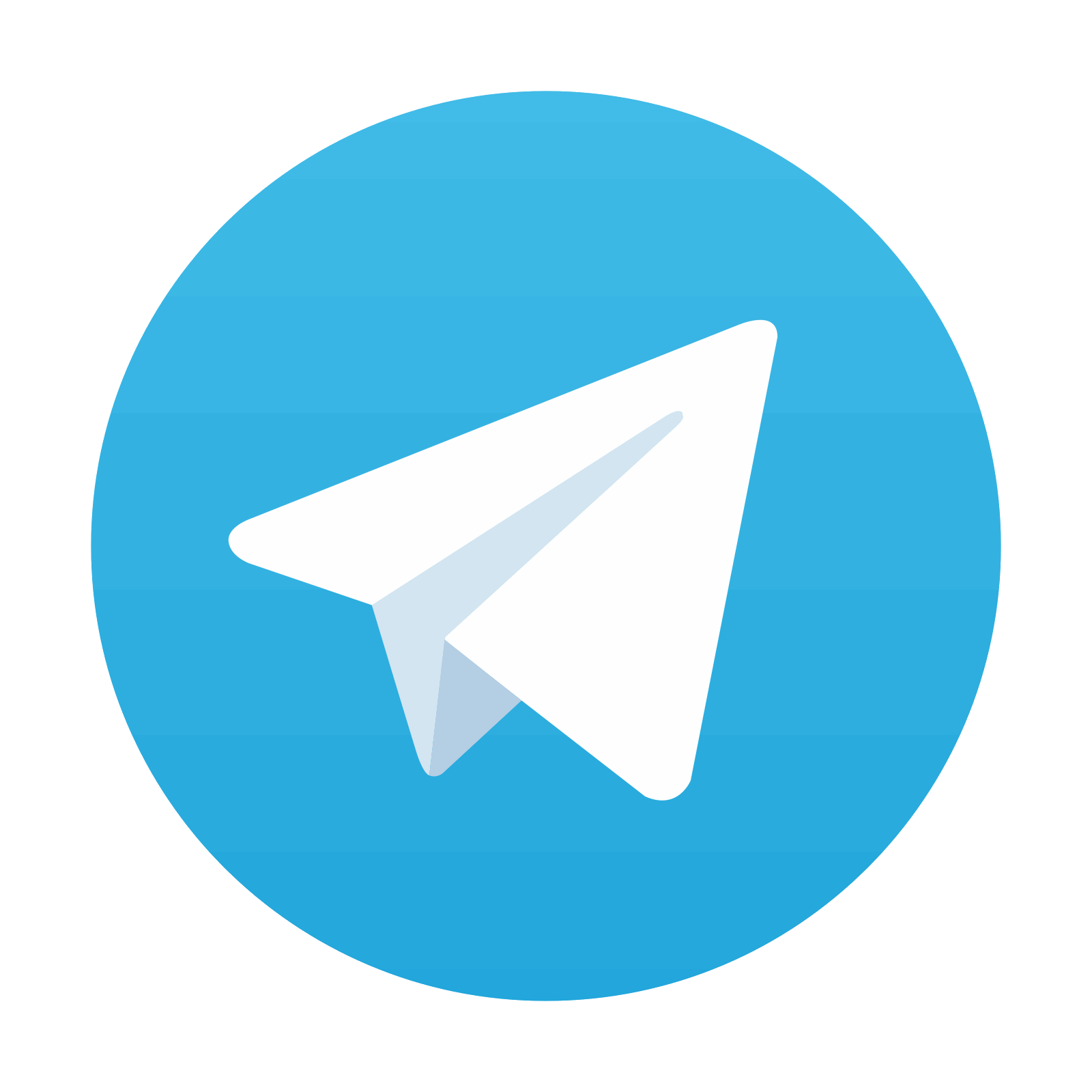
Stay updated, free articles. Join our Telegram channel

Full access? Get Clinical Tree
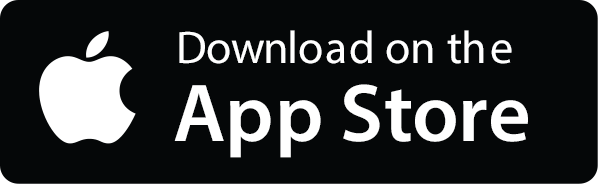
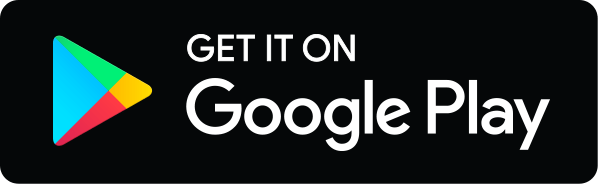
