6 Pathophysiology of gait dysfunction in normal pressure hydrocephalus
Adult Hydrocephalus, ed. Daniele Rigamonti. Published by Cambridge University Press. © Cambridge University Press 2014.
Introduction
Normal pressure hydrocephalus (NPH) presents in adult patients with gait dysfunction, cognitive decline, and urinary incontinence. Gait dysfunction typically represents the first or most prominent of these symptoms, and commonly exhibits greatest improvement following cerebrospinal fluid (CSF) drainage [1–4]. Despite their importance and debilitating effect on NPH patients, gait abnormalities remain unexplained by a unified, accepted theory. In contrast, more appears to be known regarding the mechanism of urinary dysfunction in idiopathic NPH. In this context, bladder irregularity appears to arise from detrusor overactivity in relation to aberrant cerebral autonomic control and exaggerated micturition reflexes [5].
Older theories regarding gait dysfunction in NPH targeted the pyramidal tracts controlling lower extremity movement. These hypotheses conjectured that third ventricular dilation compressed descending motor tracts within the internal capsule. Such compression could cause demyelination and/or axonal loss, resulting in amplitude reduction and latency prolongation [4]. However, later research using motor evoked potentials (MEPs) showed no evidence of aberrant conduction along the central motor pathway [4]. Furthermore, ventricular compression upon descending motor tracts in the internal capsule also should profoundly impact arm and hand movements. Nowak and Topka showed the hypokinetic movement disorder in NPH extends to upper limb motor control, specifically with grasp-lift synergy and force overshooting [6]. Nonetheless, upper limb involvement remains secondary to the hypokinetic gait disorder in the primary symptoms of NPH. These multiple findings have raised questions regarding the role of pyramidal tracts in the pathophysiology of NPH gait dysfunction. Additionally, the typical lack of lower extremity weakness in NPH patients might argue against pyramidal tract dysfunction. No commonly accepted theory regarding the mechanism of gait impairment in NPH has been otherwise formulated.
There are several anatomic substrates that can affect gait, posture, and balance, complicating the exact localization of this deficit in NPH [1]. For instance, balance control depends on the interaction between many areas that could be affected by NPH, including the frontal lobes, basal ganglia, midbrain, or brainstem postural control centers [1]. As such, several mechanisms to explain the pathophysiology of gait dysfunction in NPH have been proffered and corroborated by small cohort or research studies. This chapter presents objective measures of gait dysfunction that have been used clinically and highlights some of the major theories postulated to explain gait dysfunction in NPH.
Objective measures of gait dysfunction in NPH
Gait dysfunction in NPH has characteristic features that include a slow pace, short stride length, wide stance, and low foot-floor elevation [7,8]. Often, patients show hesitation with initiation of walking and turning. Both subjective and objective measures can be used to evaluate gait dysfunction in NPH and improvement following CSF drainage. Subjective rating scales are often used for clinical assessment. These may vary from broad categorical ratings often used in large cohorts (e.g. patient bedridden, patient ambulates but is unstable) [9], to more specific ratings (e.g. tandem walking disturbed, start hesitation) [10]. These types of evaluations are simple and represent an efficient manner of assessing gait. However, objective measures may provide more rigorous and reproducible assessments for comparison. Importantly, use of specific objective measures may allow for hypothesis testing regarding the pathophysiology of gait dysfunction in NPH.
Objective measures of gait can be used to quantify the pattern of walking and step-taking, focusing on walking speed, stride length, cadence, equilibrium, and posture. In general, the characteristic gait in patients with NPH reflects hypokinetic movement – steps tend to be short and hesitant. Several other neurodegenerative conditions including Parkinson’s disease (PD), progressive supranuclear palsy (PSP), subcortical arteriosclerotic encephalopathy (SAE), and corticobasal degeneration involve hypokinetic gait. This has led to speculation that there may be some common neural substrates involved in these different conditions. Specific measures of gait that have been useful in characterizing NPH include speed, stride length, step height or floor-to-foot clearance, and gait equilibrium [1,8]. In the typical NPH patient, all of these variables are decreased or aberrant in the case of dynamic equilibrium [1]. Other measurable variables of gait in neurodegenerative conditions include postural and turning stability [2]. It is interesting that CSF drainage in NPH patients correlates with improvements in some measures of walking (e.g. walking speed and turning), but not necessarily all those that characterize the disorder [1,10]. Several authors and research groups have used objective gait parameters in the evaluation of NPH patients following therapeutic CSF drainage to develop and test various hypotheses regarding this enigmatic pathophysiology.
Tentative theories to explain gait dysfunction in NPH
Midbrain atrophy or compression
Located in the dorsolateral region of the midbrain, the mesencephalic locomotor region (MLR) plays an important role in the initiation of locomotion in humans and other animal species [1,3]. Based on animal studies correlating abnormalities in this anatomic region to gait disturbance in PD, the MLR has been implicated in gait dysfunction of NPH patients [1]. Lee et al. conducted a retrospective review of 21 NPH patients and age-matched controls showing significantly smaller baseline midbrain diameter in NPH patients [1]. Furthermore, midbrain diameter (measured anteroposteriorly in the midsagittal plane) related inversely to the severity of gait disturbance (Figure 6.1) [1]. These findings were partially supported by separate research showing increased diameters and wider angles between the cerebral peduncles of NPH patients based on magnetic resonance imaging (MRI) [11]. These combined results suggest that midbrain atrophy or compression potentially contribute to gait dysfunction in NPH.
Figure 6.1 Measurement of midbrain maximal anteroposterior diameter (as demonstrated by the black bar) based on sagittal MRI in an NPH patient preoperatively.
Other studies evaluating midbrain size following CSF diversion corroborate the potential role of midbrain cytoarchitecture on gait dysfunction in NPH. Mocco et al. showed that increases in postoperative midbrain anteroposterior and transverse diameters in shunted NPH patients correlated with gait improvement [2] (Figure 6.2). The authors further postulated two mechanisms to explain the beneficial role of midbrain expansion on gait disturbance following ventricular shunt placement in NPH. One theory respects the adverse effect of ventricular pressure on descending MLR efferent tracts contacting the medullary reticular formation. These tracts help modulate reticulospinal fibers within the ventromedial medulla that contribute to gait and balance control. Interruption of modulatory input into the medullary reticular formation could cause locomotion and balance disturbance [2]. Furthermore, axonal decompression and reversal of this attenuated MLR input may account for the rapid gait improvement exhibited by NPH patients following CSF drainage [2]. In an alternate theory, the mechanical effects of hydrocephalus may adversely affect MLR synapses within the medulla. Therapeutic CSF drainage may restore synaptic integrity and allow reversal of symptoms [2]. These theories focus on midbrain compression, rather than atrophy, as a potential culprit in gait dysfunction in NPH.
Figure 6.2 Measurement of anteroposterior (AP) and transverse left–right (LR) diameters of the midbrain at the pontomesencephalic junction based on head CT in an NPH patient preoperatively.
The correlation between decreasing midbrain anteroposterior diameter and gait dysfunction in NPH may alternatively result from midbrain atrophy. However, potential locations or mechanisms of midbrain atrophy remain unclear. Growing evidence suggests the pedunculopontine nucleus (PPN), located within the midbrain tegmentum, plays an important functional role in human locomotion [1]. In disease processes such as PSP and PD, the PPN exhibits significant degeneration of cholinergic neurons. This measurable loss of neurons has been linked to gait freezing and postural instability in neurodegenerative conditions [1]. Additionally, focal lesions within the midbrain tegmentum housing the MLR may precipitate gait and postural dysfunction [1,2]. As an integral part of the MLR, the PPN represents a potential pathological substrate for gait disequilibrium in NPH. However, other authors challenge the role of PPN degeneration in NPH owing to lack of supporting evidence [11]. Further research may help elucidate the potential roles of PPN and MLR underlying midbrain atrophy in NPH.
Other potential explanations for midbrain volume loss in NPH focus on chemical and mechanical mechanisms. One theory postulates that hydrocephalus induces abnormalities in the dopaminergic system of the substantia nigra, resulting in decreased dopamine levels [1]. Alternatively, mechanical compression from an expanding third ventricle on the rostral midbrain may induce volume loss or atrophy [1]. By whatever mechanism, midbrain atrophy or compression represent attractive targets potentially correlated to gait disequilibrium in NPH.
General consensus does not exist to fully support the correlation between midbrain size and gait dysfunction in NPH, however. At least one prospective study conducted in Sendai, Japan, refuted the role of the MLR in NPH gait disequilibrium. Hiraoka et al. [3] showed that baseline measurements of midbrain anteroposterior and lateral dimensions and cross-sectional areas in NPH patients did not correlate with baseline gait severity. Furthermore, this study reported no significant relationship between gait improvement and changes in midbrain parameters following shunt insertion [3]. The authors of this study questioned the measurement techniques employed by the earlier studies of Lee et al. [1] and Mocco et al. [2], citing the inaccuracy of midbrain diameter measurements on routine computed tomography (CT) and MRI. The authors claimed that ventricular deformation and brainstem tilting invalidated midbrain parameters measured on routine imaging [3]. To combat these limitations, Hiraoka et al. instituted advanced reconstruction techniques to obtain cross-sectional areas of the midbrain on consistent axial images through the top of the inferior colliculus and the PPN [3] (Figure 6.3). Their findings seriously questioned the role of midbrain size and the MLR in gait dysfunction of NPH patients. The presence of conflicting views regarding the contribution of midbrain abnormalities to NPH gait disturbance warrants further investigation.
Figure 6.3 Measurement of anteroposterior (AP) and transverse left–right (LR) diameters of the midbrain based on MRI in an NPH patient preoperatively. Advanced reconstruction techniques were used to acquire an axial image through the pedunculopontine nucleus and top of the inferior colliculus. These techniques are described in Hiraoka et al. [3].
Cortical dysfunction
Recognition of cortical involvement in locomotion stems from multiple research efforts evaluating gait in healthy individuals and those with cognitive disturbances. Prior notions described gait as an automated motor activity with minimal cortical input. However, more recent analysis supports the contribution of higher-level cognitive function to walking [12]. The specific facets of cognition relevant to gait control include executive function and attention. Anatomically, executive function largely localizes to the frontal lobes, with input from other cerebral distributions [12]. The anatomic regions primarily involved include the dorsolateral prefrontal cortex (DLPFC; Brodmann’s area 9) and anterior cingulate cortex (ACC; Brodmann’s areas 24, 32) [12]. Abnormalities in executive function and attention may contribute to gait impairment in neurological disorders such as Parkinson’s disease, cerebral infarction, and NPH [12].
Several research endeavors have focused on dual-tasking experiments to portray the effects of abnormal executive function and attention on gait control. Dual-tasking represents the ability to perform two separate tasks simultaneously. The performance of both tasks requires higher-level function and the executive balance of competing attention demands. Multiple research efforts incorporate dual-task assessment to investigate the attention-demanding properties of ambulation [12]. For instance, executive dysfunction has been correlated to decreased gait speed and increased gait variability under dual-task conditions [12]. As idiopathic NPH represents a disorder of both gait and cognition, dual-tasking represents a useful experimental paradigm to evaluate these patients [12–14].
Impaired cortical and executive function may negatively impact gait, especially during dual-task ambulation. In experiments performed on non-demented and demented subjects with or without impaired executive function (IEF), Allali et al. showed the importance of cortical contributions to gait stability and dual-tasking [13]. Patients with dementia and IEF exhibited significantly greater stride times than demented patients without IEF and non-demented patients. Dementia (with or without IEF) correlated to greater stride times during dual-tasking and to greater stride time variability as compared to non-demented patients [13]. Additionally, the deleterious effect of IEF on gait heightened during dual-tasking. As frontal lobe function worsened, stride time variability increased. The authors of this study linked stride time variability to loss of dynamic stability in gait [13]. Therefore, cortical and executive dysfunction may contribute to gait impairment and instability in demented patients, including those with cognitive deterioration as in NPH. In PD, executive dysfunction likely contributes to abnormal gait as well. Clinical trials have reported the successful use of methylphenidate in these patients to improve executive function and gait speed, and decrease gait variability [12].
Other studies support the potential role of cortical dysfunction in NPH gait impairment, highlighting the relationship between gait and cognition [14]. For instance, Armand et al. reported the enhancement of gait parameters during dual-tasking in NPH patients following CSF drainage. Importantly, gait speed and stride length significantly improved only during dual-tasking, without improvements observed for single-task ambulation [14]. Aberrant integration or distribution of attention to simultaneous thoughts or tasks may negatively impact gait equilibrium. Furthermore, the authors of this study argue that dual-tasking, rather than single-tasking, better evaluated gait improvement in NPH patients following CSF drainage – dual-tasking may represent a more sensitive test [14]. The exact role of cortical dysfunction in the pathogenesis of abnormal gait requires further analysis.
Cortical-subcortical and intracortical circuit abnormalities
Several research studies target cortical-subcortical circuit pathology as the culprit in NPH gait dysfunction. Neighboring the lateral ventricles, multiple tracts descend from cortical regions to synapse upon groups of neurons within the basal ganglia, cerebellum, brainstem, and spinal cord. These tracts include fronto-ponto-cerebellar fibers, corticospinal tracts controlling lower extremity movement, and the frontal cortical-subcortical basal ganglia loop [1,14]. Compression from ventricular enlargement may temporarily damage these tracts and produce gait disequilibrium with frontal-like disturbances [1,2]. Therapeutic CSF drainage may alleviate the pressure affecting descending tracts, improving gait control and equilibrium. Specifically, beneficial modifications of periventricular regional hydrostatic pressure may relieve compression of frontal-subcortical tracts [14]. Subsequent gait improvement, especially during dual-tasking that involves executive function, could represent restoration of frontal cortical-subcortical tract physiology [14].
Aberrant circuitry responsible for NPH gait impairment alternatively may lie within the motor cortex. Recent studies have implicated intracortical connections, rather than subcortical tracts, in the development of gait dysfunction in NPH. Under this premise, the correlation between frontal lobe dysfunction and gait impairment occurs due to elevated corticospinal excitability in the setting of disinhibition [15]. Chistyakov et al. stimulated the leg representation of motor cortex using transcranial magnetic stimulation (TMS) and monitored motor evoked potentials (MEPs) in patients with NPH gait dysfunction before and after shunting. They found reduced resting motor threshold (rMT) at baseline, which indicates increased corticospinal excitability, and reduced short intracortical inhibition (SICI), which is thought to reflect abnormal function of GABAergic interneurons [15]. Ventricular shunting significantly increased both of these variables (rMT, SICI) in NPH patients exhibiting dramatic improvement in ambulation [15]. The authors concluded that motor cortex disinhibition and abnormally increased corticospinal excitability result in reduced control of motor output, contributing to NPH gait impairment [15].
Several studies have evaluated the potential role of abnormal cortical and subcortical connections in the pathophysiology of NPH gait dysfunction. Aberrant circuitry may involve reduced intracortical inhibition within motor cortex, decreased inhibitory control from pre-motor regions, dysfunction of the frontal-subcortical basal ganglia loop, or abnormal function of other frontal-subcortical tracts (including the corticospinal tract) [14,15]. Regardless of the exact mechanism, aberrant functional connectivity within or between frontal, prefrontal, and basal ganglia regions likely contributes to NPH gait dysfunction.
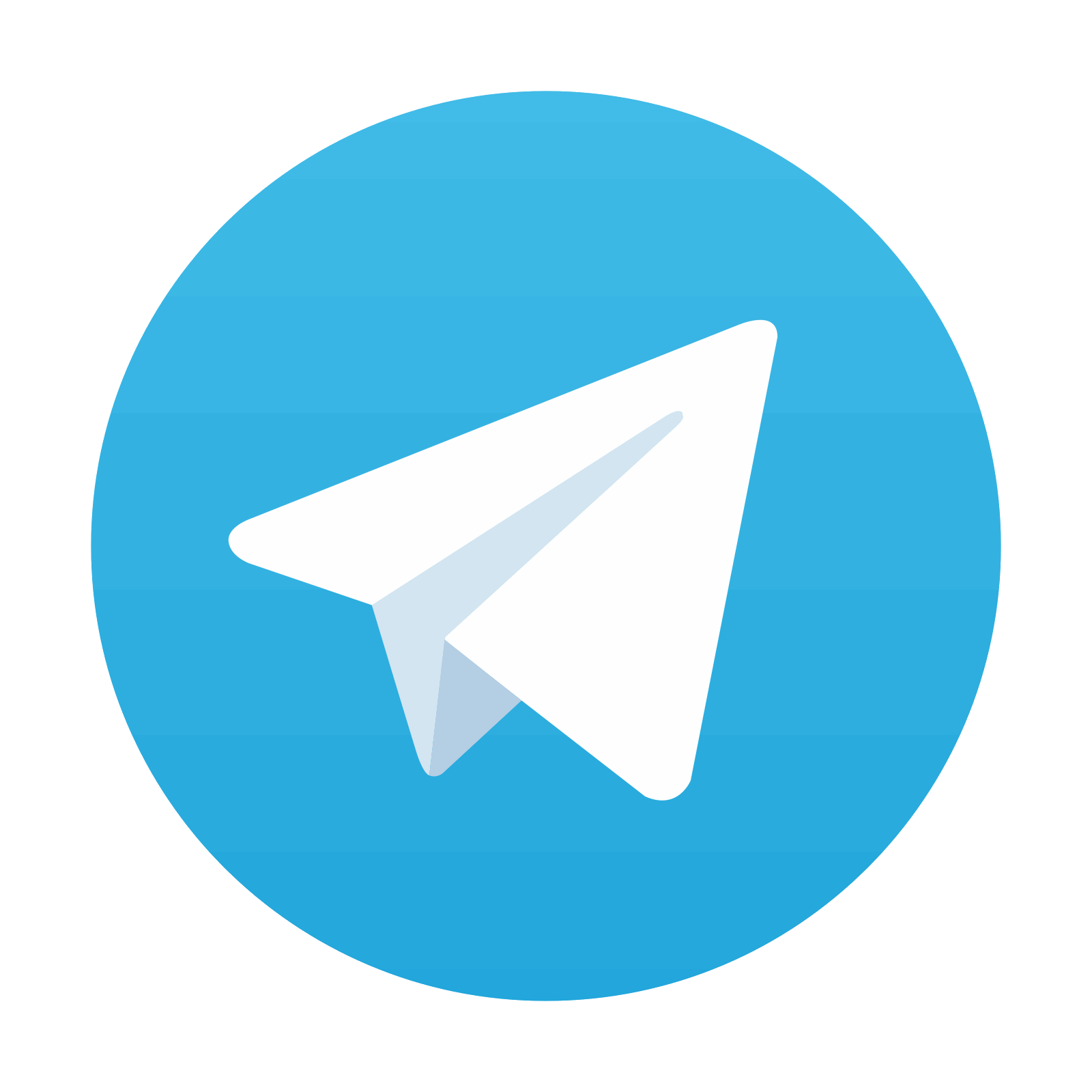
Stay updated, free articles. Join our Telegram channel

Full access? Get Clinical Tree
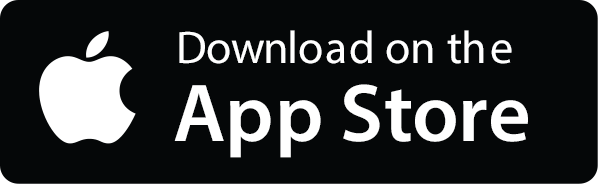
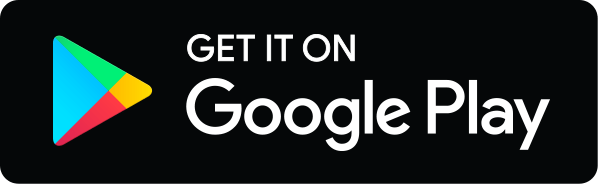
