8 Neuropsychologist’s Role in the Management of Brain Tumor Patients
Abstract
Brain tumors represent a dynamic disease in which neuropsychological functioning can change dramatically throughout the disease course. High rates of cognitive dysfunction have been reported in brain tumor patients and the presence of cognitive impairment is associated with decreased quality of life and functional independence, and shorter survival time. Neuropsychologists are becoming increasingly involved in the assessment and management of brain tumor patients at all stages of care. This chapter provides an overview of the neuropsychologist’s role in the management of patients with brain tumors with specific focus on the intraoperative stage.
8.1 The Neuropsychological Exam
Neuropsychology is a specialized field within clinical psychology that focuses on evaluating brain–behavioral relationships or the effects of disease or injury on cognitive and emotional functions. A neuropsychological evaluation provides important information about the structural and functional integrity of the brain through careful clinical interview and the administration of a series of standardized tests that are compared relative to a normative sample or to the patient’s own previous performance in the case of longitudinal follow-up. The domains often evaluated include intelligence, memory, attention, processing speed, learning and memory, language, spatial abilities, executive functions, and sensorimotor abilities in addition to assessment of mood and quality of life. It is essential to broadly sample across neurocognitive domains when evaluating patients with brain tumors, as patients may present with broad and nonlocalizing deficits in addition to more focal features.
The typical structure of a neuropsychological exam includes a record review and clinical interview to obtain relevant history followed by administration of paper-and-pencil, question-and-answer, and computerized cognitive and emotional measures. Testing can range from 15 to 30 minutes using brief, bedside screening tools (e.g., Mini-Mental State Examination [MMSE] and Montreal Cognitive Assessment [MoCA]) to more comprehensive assessment lasting up to several hours where multiple cognitive domains are evaluated in detail. While the brevity and ease of administration of screening tools are appealing, they tend to lack sensitivity in detecting more mild cognitive impairment. Specifically, when comparing the MMSE to a more comprehensive neuropsychological test battery in a sample of brain tumor patients, the MMSE showed a sensitivity of only 0.50. 1 Further, screening tools also lack sensitivity in detecting longitudinal change in cognitive functioning associated with treatment effect. 2 Accordingly, more comprehensive testing batteries sampling across cognitive domains is preferred, though test selection and battery length must be carefully considered given the propensity for fatigue in this patient population. The extent of testing is often dictated by the clinical questions being asked and the patient’s functional level. Use of measures that are repeatable with multiple forms and minimal practice effects are preferred since patients are typically reevaluated postoperatively and often at regular intervals throughout the disease course. Assessment of mood, quality of life, and symptoms common to patients with brain tumors is also important.
8.2 Neuropsychology in the Preoperative Stage
The pattern of neuropsychological deficits in patients with brain tumors can vary greatly depending upon lesion location and other patient and tumor characteristics. 3 While deficits can be observed in a variety of domains, memory and executive functions seem particularly vulnerable (Fig. 8‑1). 4 , 5 , 6 Patients with tumors in the dominant hemisphere tend to have more cognitive deficits than patients with tumors in the nondominant hemisphere, 7 and while focal syndromes (e.g., aphasia with left perisylvian tumors) can be seen, cognitive dysfunction can also be more mild and nonfocal than typical of other neurologic insults such as stroke. 8 This may be explained by more widespread effects of the tumor via its infiltrative nature and associated edema and mass effect, which can disrupt broader cerebral networks and cause impairments in domains more distal to tumor location. Growth momentum also plays an important role in a patient’s cognitive presentation with greater neuropsychological impairment observed in patients with rapidly growing tumors compared to slow-growing tumors. 3 , 9 , 10 It is likely that the slow growth rate mitigates the impairment that might be expected based purely on lesion location and size in part by allowing for greater functional reorganization and neural compensation. In fact, there are numerous case examples in the literature where large amounts of tissue can be surgically removed in patients with low-grade gliomas in expected eloquent regions without any observed functional consequences. 11

Obtaining a comprehensive neuropsychological evaluation prior to surgery is ideal and provides the medical team with a better understanding of the unique impact of the tumor on cognition. This allows for better differentiation between the cognitive effects of the tumor versus surgery and other therapies (e.g., radiation and chemotherapy) as a patient is being followed over time. Obtaining a preoperative neuropsychological evaluation also captures individual differences that can influence cognition, such as preexisting cognitive weaknesses from learning or developmental delays, cognitive changes from preexisting medical problems (e.g., vascular risk factors and seizure disorder), and the impact of cultural differences on test performance. Without having a pretreatment baseline, abnormal test performances attributed to these factors can sometimes be misinterpreted as related to the disease. It is not always feasible to obtain a comprehensive evaluation prior to surgery given the medical urgency and short time frame to proceed to surgery, especially in high-grade glioma patients, and in such cases baselining a patient after surgery but prior to initiation of adjuvant therapies is a reasonable alternative. Establishing a baseline is also critical to monitoring disease status over time. Studies have shown that changes in neuropsychological status can predict tumor recurrence, 12 in some cases even before evidence of radiographic progression. 13 Being able to detect such changes over time is dependent on having a baseline or benchmark for longitudinal comparison.
Neuropsychological testing can also inform surgical risk to cognitive functioning. Preoperative neuropsychological testing has been shown to be a strong predictor of postoperative outcomes in specific cognitive domains with greater preoperative memory and language performance associated with greater postoperative declines in these areas. 14 The duration or persistence of a presenting deficit can also be used to assess risk. Consider, for example, a patient presenting with a transient aphasia that resolves with steroid treatment. The transient nature suggests lesion proximity to language areas, presumably from edema or mass effect, but the fact that it resolved indicates that those systems have not been irreversibly damaged by the tumor and that with careful surgical planning risk can be mitigated. This is in contrast to a patient who presents with a persisting deficit where predictive value is less clear.
Preoperative neuropsychological testing adds further value to the broader presurgical workup in determining the feasibility of brain mapping procedures, whether pre- or intraoperative. Patients presenting with severe deficits such as profound aphasia may be poor candidates for functional magnetic resonance imaging (fMRI) or intraoperative mapping. If brain mapping is deemed feasible, this preoperative assessment is highly beneficial for determining specific domains for intraoperative focus and identifying situations in which paradigms need to be modified (e.g., to meet the ability level of the patient). Given that patients vary considerably with respect to functional level, it is important to take a highly individualized approach to selecting testing materials for intraoperative mapping. Baselining a patient on intraoperative mapping protocols during the preoperative assessment familiarizes the patient with the testing protocols they may be exposed to during surgery and allows the examiner to select only those items that each individual patient can reliably and accurately respond to, thus creating a set of test stimuli that are specific to that patient. By doing so, the examiner can have greater confidence that errors occurring during surgery represent potential surgical effects or true disruption from stimulation rather than lack of knowledge or preexisting deficits.
8.3 Neuropsychology in the Intraoperative Stage
Intraoperative mapping requires a coordinated effort across disciplines (Fig. 8‑2). There is considerable variability across institutions in the personnel used to perform behavioral and cognitive testing during awake surgery. Speech pathologists, neurologists, neurosurgical fellows or residents, anesthesiologists, and surgical nurses have all been used in this capacity. Neuropsychologists are particularly well suited for this role given their expertise in psychometrics and test design, functional neuroanatomy, and their high-level conceptual understanding of cognitive functions, which allows for more informed task development, task selection, and interpretation of mapping results. In fact, recent evidence suggests higher rates of gross total resection, shorter duration of surgery, and lower rates of unexpected residual tumor when neuropsychologists are used for intraoperative mapping. 15

There is also significant variability in the extent and timing of cognitive testing during surgery. Direct cortical stimulation (DCS) is often used to explore the cortical surface for eloquence prior to starting surgical resection and for targeted mapping of white matter tracts and deep subcortical gray matter structures. However, DCS constitutes a relatively small portion of the overall surgery and there is often significant time during which resections are performed without stimulation. During these times, intraoperative cognitive monitoring (IOCM) can be conducted wherein the patient is continuously tested and assessed for cognitive change or evolving deficits. When changes in performance are observed, the neurosurgeon is notified, and surgical decisions are made accordingly. IOCM is particularly valuable in the context of negative mapping when DCS produces no disruptions or responses. Negative mapping is quite common and can be due to factors such as small craniotomy with minimal exposed cortex, individual variability in functional organization, inadequate stimulation parameters (e.g., mA limited by after-discharges), functional reorganization associated with the brain lesion, use of inappropriate tasks to assess the target area, and neural connectivity engaging a distributed network that cannot be sufficiently disrupted by stimulation of a small area. 16 Negative mapping does not negate the possibility of postoperative deficits, and IOCM can help mitigate this risk as it can capture cumulative or additive effects of active resection on cognitive networks.
A highly individualized approach to task selection for DCS and IOCM is advocated. Tasks should be selected based on knowledge of functional neuroanatomy and the proximity of the tumor to known eloquent areas, localization data obtained from functional neuroimaging studies and diffusion tensor imaging (DTI), and review of presenting symptoms and baseline neuropsychological test performance. Testing should also be multidimensional in nature as tumors can be located in areas where more than one function has proximity to the tumor border. Take for example the patient shown in Fig. 8‑3 where there is a lesion located in the posterior left middle temporal gyrus. This lesion has proximity on its posterior border to the angular gyrus, indicating possible risk to semantics, reading, and other dominant parietal functions (e.g., writing, math, right–left orientation, and finger localization); immediate proximity at the anterior border to the arcuate fasciculus (AF), suggesting risk to phonological retrieval systems; and immediate proximity on its medial border to a bundle of fibers containing the inferior longitudinal fasciculus (ILF), inferior frontal occipital fasciculus (IFOF), and optic radiations (OR), indicating possible risk to semantics (from the ILF and IFOF) and vision (from the OR). In this case, vascular anatomy complicated the surgical pathway to the lesion and fMRI showed robust language activation around the lesion. Mapping this type of case requires testing of multiple functions, DCS to inform the approach and particular high-risk areas, as well as continuous IOCM to monitor for moment-to-moment fluctuations in neurocognitive functioning.

Awake craniotomies have historically focused on sensorimotor and language mapping. Sensorimotor mapping is relatively straightforward with respect to anatomic localization and technique and is discussed in detail in another chapter of this book. Mapping language is far more complex both conceptually and anatomically. It is widely accepted that language involves a more distributed network than Broca and Wernicke areas and that there are anatomically dissociable regions that are specialized in processing specific linguistic aspects of language. See Hickok and Poeppel, 17 Chang et al, 18 and Binder 19 for a review of eloquent regions associated with critical language functions. For example, there are regions in the ventral temporal occipital, or fusiform area, that are specialized in processing orthographic or written letter content, areas in the mid-superior temporal gyrus and sulcus dedicated to processing phonology or speech sounds, areas more posterior in the superior temporal gyrus and inferior parietal lobule involved in phonological access and retrieval, and areas in lateral middle and inferior temporal lobe, posterior inferior parietal lobe (angular gyrus), and dorsolateral frontal cortex that are involved in processing semantics (i.e., the meaning of pictures, words, phrases, etc.).
Language mapping often involves administration of relatively simple tasks to test basic, automatic speech functions, such as counting or reciting overlearned phrases (e.g., days of the week, alphabet, and Pledge of Allegiance), and if a disruption is observed, the examiner can further explore the basis for the deficit by having the patient phonate or repeat sounds such as “pa-pa-pa,” “la-la-la,” and “ga-ga-ga” to evaluate mouth, tongue, and palate movements. Object naming is considered the “gold standard” for mapping language. Naming objects engages a number of language processes, including phonological access, lexical–semantic retrieval, and speech motor functions, allowing for broad sampling of the language network with one task. There is significant individual variability in the location of object naming sites when tested with DCS, with disruptions seen over a wide area of left lateral cortex including temporal, parietal, and frontal regions. 18 Other naming paradigms have been developed that differ in input modality and semantic category. For example, auditory naming has been associated with more anterior temporal localization than visual naming, 20 , 21 , 22 greater verb than object naming specific sites have been reported in the prefrontal cortex, 23 , 24 and naming proper nouns has been associated with the temporal pole and uncinate fasciculus. 25 , 26 Repeating nonwords has been shown to be effective in mapping phonology systems in the vicinity of the AF 27 and nonverbal semantics using a picture semantic association task (i.e., selecting which picture from two choices is most related to a target in meaning) has been shown to be useful in mapping the ventral semantic stream (IFOF) in the left but also right hemisphere. 28 , 29 , 30 Further research and discussion regarding language mapping is presented in Chapter 11 (Speech Mapping).
While risk for cognitive complications is often assumed to be lower in nondominant hemisphere resections, declines in cognition have been reported following these resections 31 and the concept of noneloquence in brain areas beyond dominant hemisphere language regions should be challenged. The right hemisphere is known to play an important role in visuoperception, attention, and social cognition 32 and there is increasing focus on developing tasks to assess these functions during awake surgery, which frequently involves adaptation of traditional neuropsychological measures to the operating room (OR) environment. For example, unilateral spatial neglect whereby the patient cannot attend to one side of space (most often the left side with right-sided lesions) can result from right parietal, frontal, or even subcortical damage. Line bisection tasks are commonly used to assess neglect; however, physical positioning of the patient in the OR often prohibits a precise written response. Adapted line bisection tasks can circumvent motoric requirements, such as a colored line bisection task used by the authors of this chapter. In this task, the patient is shown a horizontal line divided into two colored segments (e.g., half in red and half in green) and they indicate verbally if the two segments are equal in length or one is longer. Neglect should be suspected if the patient states that the color on the left is shorter than the color on the right when they are actually equal in length (i.e., the patient is neglecting a portion of the color segment on the left). We have also adapted cancellation tasks (which typically require patients to cross out target letters/symbols within an array of distractors) for the operating room. In these visual search tasks, the patient is presented an array of letters among distractor symbols on a screen divided into four labeled quadrants and verbally indicates the quadrant of the target letter. Accuracy and reaction time are monitored and omissions and/or slowing when searching the left side of space may indicate neglect. Double simultaneous stimuli presentation can also be used where the patient fixates on a central crosshair and stimuli (flashing dot or numbers) are presented unilateral or bilaterally and the patient has to indicate on which side they see the stimuli. Conditions where they perceive unilateral stimuli but not stimuli on the left during bilateral or simultaneous presentation may indicate neglect.
Mental rotation tasks can also be used to test the nondominant parietal lobe. In these tasks, patients identify whether two objects are the same or different by mentally rotating them. In a meta-analysis and review of neuroimaging studies, Zacks 33 found that regions in the superior parietal, frontal, and inferotemporal cortices were consistently activated during these tasks. While activation was bilateral in most areas, parietal activity was more common in the nondominant, right hemisphere (specifically posterior parietal cortex). Math tasks (e.g., basic calculation) can also be used to test parietal functioning. Acalculia can result from damage to the dominant angular gyrus, and fMRI studies have shown activation along the intraparietal sulcus, parietal lobe as well as prefrontal cortex and other regions, with laterality differences based on the nature of the task. 34 Social cognition tasks (e.g., facial emotion recognition, emotional prosody, empathy, and theory of mind tasks) have also shown to activate regions including the supramarginal gyrus, angular gyrus, superior temporal gyrus, middle temporal gyrus, and aspects of the frontal lobes 35 and deficits have been reported following right inferior frontal surgery. 36 , 37
Executive functioning is an umbrella term used to describe higher order cognitive processes such as planning, shifting from one mental set to another, updating and monitoring of information, and inhibitory control that commonly localize to frontal regions of the brain. 38 Monitoring of executive functions is, thus, particularly important during frontal resections, though executive functioning also involves more distributed cortico-cortico and cortico-subcortical networks and executive deficits can develop with damage outside the frontal lobes. Tasks to assess executive abilities intraoperatively include those assessing inhibitory control (e.g., Stroop color–word interference tests, Go/No Go tests such as squeeze if examiner taps on hand twice, do nothing if examiner taps on hand once), working memory (e.g., reverse digit sequencing), verbal fluency (e.g., saying as many words starting with a particular letter within a short period of time), and mental flexibility (e.g., Oral Trails Test requiring alternating recitation of ascending numbers and letters).
As depicted earlier, there is a growing range of testing paradigms that can be utilized for brain mapping and it is neither feasible nor clinically indicated for all patients to be administered all tests within this large range. Again, we reiterate the importance of taking an individualized and functional anatomically informed approach to task selection to optimize mapping/monitoring for any given patient. In addition to greater focus on task development, there is also increased attention on identifying the most effective and innovative methods for administering testing in the OR where efficiency is critical and surgical progress should not be slowed by cumbersome testing procedures. Laptop- and tablet-based systems are increasingly utilized to display stimuli to patients (e.g., PowerPoint presentations) and allow for extensive stimuli to be stored and displayed electronically, rather than needing hard copies of stimuli in the OR. However, the transition to computers alone does not address the often unstructured and highly variable testing methods used across institutions. Increased consensus in this regard would be valuable in allowing for more comprehensive research endeavors with larger samples across collaborating institutions and further identification of best practices. Consensus is becoming increasingly possible through development of specific surgical brain mapping software packages/applications that can be used in a standardized fashion across institutions. One such testing platform is NeuroMapper, which was developed by the lead author of this chapter. It utilizes dual iPads to administer a variety of cognitive paradigms to patients in a highly customized and flexible manner, allows for highly individualized mapping by using baseline performance to select stimulus sets for the operating room, allows examiners to quickly and easily code patient responses (e.g., correct/incorrect and types of errors made) and monitor changes in task accuracy and reaction times in real time, and video records patients responses for later review. Fig. 8‑2 shows the NeuroMapper testing platform setup in the operating room and Fig. 8‑3 shows examples of some of the tasks included in the package.
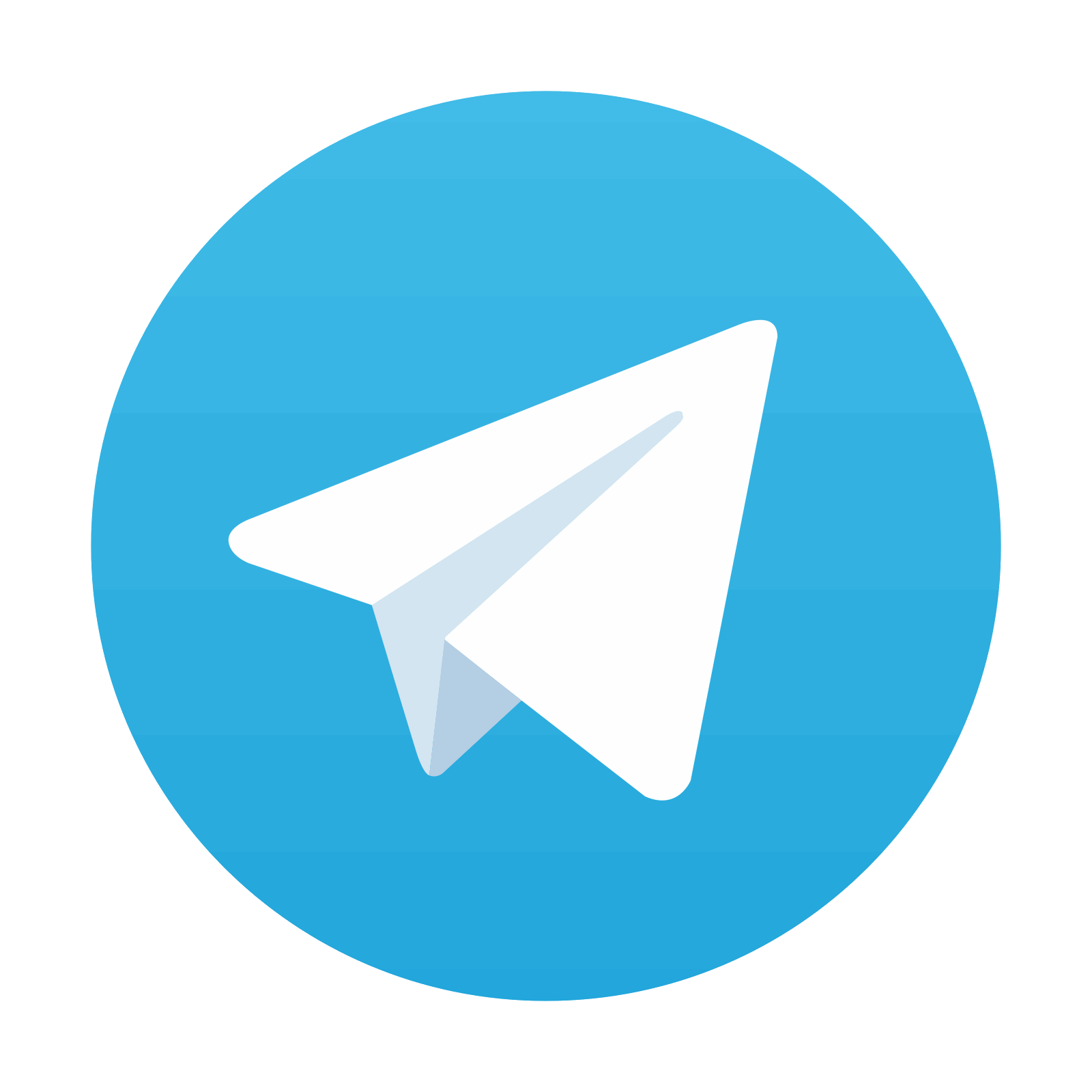
Stay updated, free articles. Join our Telegram channel

Full access? Get Clinical Tree
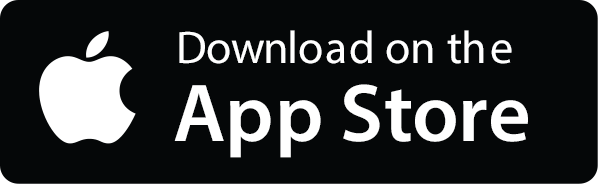
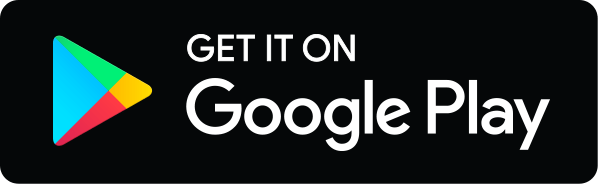