Activation Procedures
Thoru Yamada
Elizabeth Meng
Activation procedures include various sensory and pharmacological stimulations to alter the physiological state. They are usually aimed at eliciting or enhancing abnormal activity, especially epileptiform activity. The most commonly used sensory stimulation is photic stimulation. Others include tactile or electrical stimuli for somatosensory stimulation and music or sounds for auditory stimulation. Pharmacological activation includes pentylenetetrazol to induce a seizure or benzodiazepine to attenuate one. The most routine activation procedures in any EEG laboratory are hyperventilation (HV), photic stimulation (PS), and sleep.
Hyperventilation
NORMAL HYPERVENTILATION RESPONSE
This procedure consists of deep and regular breathing at a rate of about 3 to 4 per 10 seconds for a period of 3 to 5 minutes. In young children, HV can be successfully performed by asking the child to blow on a pinwheel. A characteristic HV response consists of bilaterally diffuse and synchronous slow-wave bursts, initially with theta frequency and then progressing to delta frequency. This is called “HV buildup” (Figs. 9-1A and B and 9-2A and B). The amplitude may reach as high as 500 µV. Theta-delta buildup by HV is usually anterior dominant in adolescents or adults but may be posterior dominant in children. These occur in a serial semirhythmic fashion with fluctuating amplitude (Video 9-1). The effect is most prominent in children between the ages 8 and 12 years and progressively decreases toward adulthood (compare Figs. 9-1B and 9-2B); a clear HV response is seen in about 70% of children, but in adults, it may be less than 10%.1 HV effects, however, vary considerably from one individual to another. Physiologically, HV reduces the carbon dioxide concentration (PCO2), which causes vasoconstriction and reduction of cerebral blood flow. The reduction of PCO2 (hypocapnia) is likely the major factor in producing HV buildup.1 HV buildup is enhanced by a blood sugar level below 80 mg/100 mL.2 Therefore, HV buildup may be more prominent when the patient is hungry or his/her last meal was some time ago. In subjects who show a low-voltage and poorly defined alpha rhythm, HV may bring out a better defined alpha rhythm.
Some delta bursts induced by HV may include “spiky” or spike-like discharges (small notched spikes preceding or mixed with theta-delta activity) especially in children. Unequivocal spikes or clear focal or lateralizing (focal) changes elicited by HV are considered to be abnormal. After cessation of HV, the patient may complain of numbness or tingling in the fingers and lips, transient blurring of vision, or ringing in the ears. Some may even show changes of consciousness or awareness. These symptoms are self-limiting and are not necessarily associated with EEG changes or related to the degree of buildup. Likewise, after cessation of HV, the slow waves disappear quickly and the EEG returns to the pre-HV state within 30 seconds. In some subjects, though, the effect may continue for a minute or longer. One should be cautious in interpreting a long-lasting post-HV effect, since some subjects may continue to hyperventilate even after being told to stop. The technologist should observe carefully to make sure that the patient did indeed stop HV. If the delta bursts appear longer than 1 minute in the post-HV period, they are not likely related to the HV effect. (An exception to this is seen in moyamoya disease.)3,4
ABNORMAL HYPERVENTILATION RESPONSE
HV is a well-known activation procedure for inducing absence seizures. HV activates more than 80% of untreated children with absence seizures.5 It is important for the technologist to document clinical signs associated with an absence seizure. With a sudden onset of rhythmic (monomorphic) 3-Hz spike-wave bursts (Fig. 9-3; Video 9-2, also see Video 10-6), the patient usually stops HV and often stares into space, sometimes with eyelid or facial muscle twitches. If 3-Hz spike-wave bursts last longer than 5 seconds, the technologist is usually able to observe a clinical change by examining the patient’s level of consciousness. An astute technologist will quickly ask the patient to remember words presented during the event and ask the patient after the event if he or she can recall the presented words. If the patient’s communication or consciousness is impaired, the patient will not be able to recall the word spoken during the episode. A more accurate assessment may be made by testing reaction time; the patient is instructed to press a button (which makes a mark on the EEG recording) in response to an auditory signal given by the technologist during the event of 3-Hz spike-wave bursts. With cessation of the spike-wave
bursts, the patient usually resumes HV without being prompted by the technologist. There is no postictal confusion or impaired consciousness.
bursts, the patient usually resumes HV without being prompted by the technologist. There is no postictal confusion or impaired consciousness.
![]() FIGURE 9-2 | Comparison of resting EEG in the awake state (A) and EEG during hyperventilation (B) in a 38-year-old man. Note the generalized bursts of 4- to 6-Hz theta waves. This is unusually prominent HV response in this age of patient. The frequency of bursts is faster than that seen in children (Fig. 9-1B). |
HV may also activate focal or other types of generalized seizures, or precipitate interictal epileptiform activity, though the incidence of such activation is far less (˜5%) than that for absence seizures. Much more vigorous and prolonged HV is usually required to elicit partial seizures.6
HV may accentuate focal slowing, which is sometimes useful for verifying uncertain or subtle focal features observed in the resting EEG. One unique HV effect has been observed in moyamoya disease in which the delta bursts reappear 3 to 5 minutes after cessation of HV, called the “re-buildup” HV effect.3,4
CONTRAINDICATIONS OF HYPERVENTILATION
The American Clinical Neurophysiology Society (formerly American EEG Society)7 recommended that HV should not be performed in certain clinical settings.
Included contraindications are acute stroke, recent intracranial hemorrhage, large-vessel stenosis, recent TIA, moyamoya disease, severe cardiopulmonary disorders, and sickle cell disease or trait. All these conditions are related to cerebrovascular problems.
Photic Stimulation
Photic stimulation is a routine activation procedure performed in most EEG laboratories. This is done primarily to elicit a photoparoxysmal response for the diagnosis of photosensitive epilepsy. Photic stimulation provides other physiological responses but of less diagnostic value.
PROCEDURE
Photic stimulation commonly uses a stroboscope to deliver a repetitive, diffuse, flashing light of brief duration (10 to 30 ms). The repetitive rate is usually 1 to 30 Hz. The strobe light is placed directly in front of the patient’s eyes at a distance of about 30 cm. The patient’s eyes are usually closed during delivery of photic stimulation, but some laboratories prefer to have the eyes open at first and closed during the middle of photic stimulation (because this may enhance the incidence of the photoparoxysmal response).
The duration of each set of repetitive stimuli is usually 10 seconds, followed by 10 seconds of resting time before delivering the next set of stimuli. The stimulus rates vary according to laboratory protocols and technologist preference. It is typical to deliver a series of six to eight different frequencies. Some laboratories include crescendo type and decrescendo type of stimulus rates with a progressively increasing stimulus rate from 1 to 30 Hz and then a progressively decreasing stimulus rate from 30 to 1 Hz (see Fig. 7-26C).
PHOTIC DRIVING RESPONSE
The photic driving responses elicited by 5- to 30-Hz stimuli consist of occipital dominant rhythmic waves with a one-to-one frequency relationship with each flash (Fig. 9-4A).
Within one set of flashes, the driving response frequency may change from one-to-one to a harmonic or subharmonic pattern (Fig. 9-4B). The responses are usually most prominent at the flash frequency closest to the frequency of the individual’s alpha rhythm. In a young child, the driving response may be elicited at theta frequency flashes. The amplitude of the photic driving response is generally higher in children and better visualized in adults with low-voltage background activity. The patients who have large lambda waves and/or POSTS tend to have large photic driving responses (see “Lambda Waves,” Chapter 7; see also Fig. 7-26A-C). A photic driving response is absent in a blind person, but absence of a photic driving response per se is not an abnormal finding. It should be noted that the photic response with a slow frequency (<3 Hz) is not a driving response but rather an evoked potential elicited by each flash (Fig. 9-5). In some subjects, there are diffuse sharp discharges at the onset or offset of photic stimulation, called the on-response and off-response, respectively (see Fig. 9-4B).
Within one set of flashes, the driving response frequency may change from one-to-one to a harmonic or subharmonic pattern (Fig. 9-4B). The responses are usually most prominent at the flash frequency closest to the frequency of the individual’s alpha rhythm. In a young child, the driving response may be elicited at theta frequency flashes. The amplitude of the photic driving response is generally higher in children and better visualized in adults with low-voltage background activity. The patients who have large lambda waves and/or POSTS tend to have large photic driving responses (see “Lambda Waves,” Chapter 7; see also Fig. 7-26A-C). A photic driving response is absent in a blind person, but absence of a photic driving response per se is not an abnormal finding. It should be noted that the photic response with a slow frequency (<3 Hz) is not a driving response but rather an evoked potential elicited by each flash (Fig. 9-5). In some subjects, there are diffuse sharp discharges at the onset or offset of photic stimulation, called the on-response and off-response, respectively (see Fig. 9-4B).
PHOTOPAROXYSMAL RESPONSES
This was formerly referred to as the “photoconvulsive response,” but the use of this term is now discouraged. Photoparoxysmal responses (PPRs) typically consist of high-amplitude generalized irregular spike-wave or polyspike-wave bursts, with either bianterior or biposterior dominance (Figs. 9-6A and B and 9-7A and B; Videos 9-3; see also Video 10-7; Figs. 10-6B, 10-18B, and 10-21B). The most effective frequency is 15 to 20 Hz.8 The incidence of PPR is highest between ages 6 and 15 and decreases with age.9,10 PPRs correlate highly with a diagnosis of epilepsy; approximately 70% to 80% of patients with PPRs have epilepsy.11,12 The seizure correlation is especially high in PPRs with spike-wave bursts that persist well beyond termination (>200 ms) of the flash stimulus (sustained PPR) (see Figs. 9-6A and 9-7B), as compared to the PPRs in which the spike-wave bursts cease at or before the termination of the flash stimulus13 (self-limited PPR) (Fig. 9-6B). The frequency of PPRs is typically independent of the photic stimulus rates. Some of the atypical photic driving responses may consist of spike-wave-like discharges which are time locked and sustained in a one-to-one relationship with the stimulus rate. These should not be considered a PPR (Fig. 9-8).
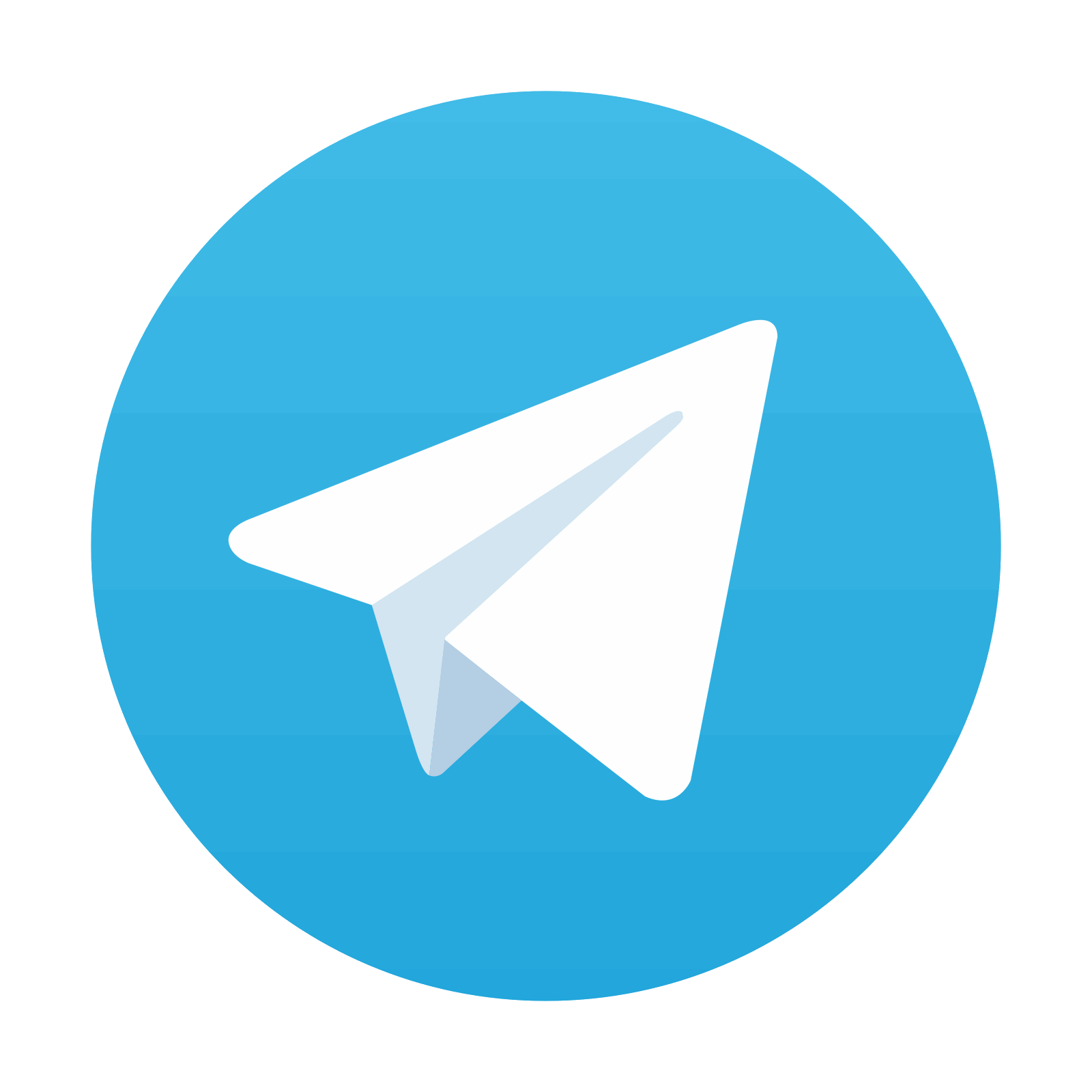
Stay updated, free articles. Join our Telegram channel

Full access? Get Clinical Tree
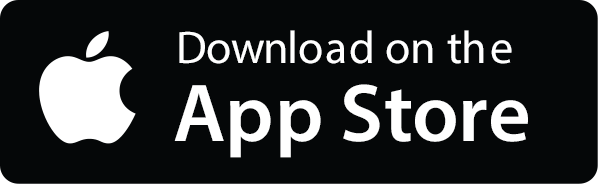
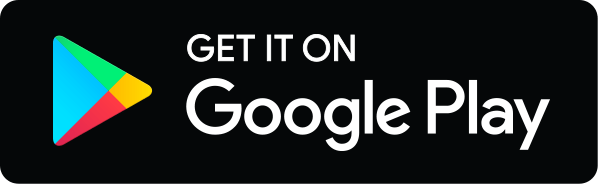