INTRODUCTION
Dementia is a significant cause of disability and mortality among the elderly1, accounting for nearly 30% of the population-attributable risk of death after age 852. With the continued growth of the world’s oldest population segments, the numbers affected by dementia are expected to rise exponentially, reaching 81.1 million by the year 20403. The most common form of late-life dementia, Alzheimer’s disease (AD) accounts for about 50% of dementia among people in their 60s, but may represent as much as 80% in those over age 804,5. The annual cost of caring for one person with AD is estimated to range from $9000 early in the disease course to nearly $20 000 in mid-course6. Given the rapid growth in the proportion of those who survive into the latest stages of AD, in the United States alone, the total annual economic burden of the disorder is estimated at $100 billion7, making it the country’s third most costly health condition after cancer and heart disease8.
With the exception of familial, early-onset forms of AD, there is no single identified cause of the disorder. Current treatments are largely symptomatic, providing only temporary improvement in cognition and neuropsychiatric symptoms. The past several decades have witnessed an explosion of research into risk and protective factors for AD, which someday may yield effective strategies for prevention. This chapter will review the current evidence for risk and protective factors associated with AD and end with a discussion of preventive strategies.
Age and Sex
In discussion of prevention, it is useful to dichotomize those risk or protective factors for AD that are potentially subject to intervention versus others. The latter, immutable risk factors are age, female sex and family history. Family history and genetic risk are reviewed by Tsuang (Chapter 43) and are not addressed here. Age is the single strongest risk factor for AD. After age 65, the prevalence and incidence of the condition appear to double with every five years of additional age until 909, when the rates either plateau or decline. In the Cache County, Utah, population, for example, prevalence estimates ranged between 0.61% at ages 65-69 to nearly 19% at ages 85-8910. Incidence rates exhibited a similar trend11. Although data are scarce for persons older than age 90, some studies have suggested a plateau or even a decline in incidence rates in extreme old age.
Second only to age in importance is genotype at the polymorphic locus APOE that encodes the cholesterol transport protein apoE. The onset of AD is strongly accelerated among individuals homozygous for allele e4 (about 2% of most populations), but also tends to be earlier in people with a single e4 allele (typically 25-30%). These allele-specific modifications in onset result in increased AD incidence at younger ages, which appear as an overall increase in risk estimates that are typically adjusted for age12.
Sex differences in the prevalence and incidence for AD have also been reported, with higher percentages observed in females in some (e.g. see10,11,13,14) but not all populations (e.g. see15,16). Whether this trend is found in any one study may depend on its relative proportions of very old subjects, among whom rates in women may be disproportionately elevated11,14.
The mechanisms underlying the sex-specific difference in AD risk are of interest, but are presently unknown. Some authors have attributed the difference in risk to the greater longevity of women or the higher prevalence of vascular dementia in men (possibly deterring a diagnosis of AD even in the presence of its characteristic pathology), possible hormonal effects, or even the notion that current cohorts of elderly women have less neural or cognitive reserve than men, owing to fewer years of formal education17. The last explanation seems to us not only contentious but unlikely.
The increasing risk of AD with age (and in women) may instead reflect a host of background physiological changes that accompany ageing including programmed ‘intrinsic’ factors such as apoptosis, dysfunction of repair processes by progenitor cells in the dentate gyrus of the hippocampus or vascular endothelium, chromosome telomere shortening which signals apoptosis and damage to mitochondrial DNA (reviewed by Drachman18). ‘Extrinsic’ factors that occur as the result of normal wear and tear or exposures that accumulate over the lifetime may also contribute. These processes include oxidative stress and the glycation of proteins which interact with reactive oxygen species to form advanced glycation endproducts (AGE). AGE may trigger inflammatory cytokines that damage the vascular endothelium. Chaperone proteins that normally repair or eliminate misfolded proteins may fail18, leading to myelin breakdown. Cortico-cortical association fibres are especially vulnerable, and correspond to brain regions most affected in AD (reviewed by Bartzokis19). The degree to which individuals experience these age- related changes likely varies according to their genes, environmental exposures and lifestyle factors, at least some of which may be related to biological sex or gender roles.
Neural and Cognitive Reserve
The notion of neural and cognitive reserve reflects an underlying idea that the brain can withstand some degree of compromise while still maintaining normal cognitive and behavioural functions through the recruitment of alternative neural networks20,21. Cognitive reserve (first proposed by Robert Katzman) is a concept whereby various cognitive strategies may be used to compensate for deficits. Both forms of reserve are malleable and may be built up through the accumulation of life experiences. Years of occupational and educational attainment are thus seen as predictors of reserve. Theoretically, higher levels of reserve could provide some degree of protection against the expression of clinical AD symptoms in the face of a specified burden of pathological change. Indeed, many epidemiolog- ical studies have reported an inverse relation between educational or occupational attainment and risk of AD, but studies have also failed to find this association (reviewed by Stern)21. The discrepant results may reflect inadequate assessment, particularly of activities in late life that continue to build neural and cognitive reserve. There is some evidence that stimulating activities in late life may reduce the risk of AD or dementia (see lifestyle factors under preventive strategies). Other explanations are possible, however, particularly when the studies employ two-stage screening and examination for dementia22.
Traumatic Brain Injury (TBI)
Several epidemiological studies have reported an increased risk of AD among persons with a history of head trauma. A meta-analysis of case-control studies reports a 1.8-fold increase in risk among those with TBI with loss of consciousness. Adjusting for other factors, the higher AD risk was found only among men (relative risk of 2.723), a result confirmed by a subsequent meta-analysis24. Interactions of TBI with APOE genotype have been reported by some25 but not others26. The mechanism underlying the association between TBI and AD is unclear. Studies have reported an increase in beta-amyloid deposition and AD pathology following TBI27, and higher frequency of APOE e4 carriers among those with cortical plaques28. In animal studies, results have varied according to species and genetic variation. Increased beta-amyloid deposition was found later in the course of recovery in rodents, but earlier in pigs. In Alzheimer’s PDAPP transgenic mice, beta-amyloid levels increased acutely, but without an increase in deposition, whereas in Tg2576 APP transgenic mice, repeated trauma resulted in greater beta-amyloid deposition29. Compounds that reduce beta-amyloid deposition after brain trauma are under active investigation to reduce the neurocognitive sequelae post injury and the risk of AD (e.g. see30,31).
A number of risk factors for cardiovascular disease have been associated with AD. Hypertension, high cholesterol, diabetes, smoking and atherosclerosis have all been associated with increased risk. For hypertension, studies report a 1.2- to 4.5-fold increase in risk for AD, depending upon the age at measurement and whether systolic or diastolic measures are taken32-35. The relation between blood pressure and AD may be non-linear, as low diastolic blood pressure in mid-life has also been associated with an elevated risk33. Elevated cholesterol is associated with a 1.4- to 2-fold increase in AD risk32, diabetes, nearly a 2- to 3.4-fold increase34,36,37, smoking, a 1.6- to 2-fold increase34,38 and severe atherosclerosis, a 3-fold increase in risk39. With each additional vascular risk factor, there is a corresponding increase in risk34,35.
Interestingly, some studies report that the relation between cardiovascular risk factors and later dementia risk varies according to the timing of measurement relative to the onset of dementia as well as genotype at APOE. Although elevated blood pressure or cholesterol levels predict later AD onset, measurements of each decline prior to the onset of dementia37,40. This phenomenon may explain the negative findings reported by some studies (reviewed by Launer41). APOE genotype may also modify the association between vascular factors and AD. In a large Dutch population study of dementia and cognitive decline, the risk of AD with significant atherosclerosis (two or more indicators of plaques in the carotid artery, thickening of the intima media wall of the carotid artery, or evidence of peripheral artery disease) varied according to APOE genotype: among those with at least one APOE e4 allele, the risk of AD was 3.9, and for others, 1.439. For smoking, increased risk of AD was found only among those without e438. Further study of the interaction between vascular risk factors and APOE genotype is needed.
The mechanism underlying the relation between vascular risk factors and AD risk is unclear. Cerebrovascular pathology is common in AD brains, and includes such changes as amyloid angiopathy, cerebrovascular atherosclerosis, endothelial damage and periventric- ular white matter lesions42. Co-existing vascular lesions occur in approximately 24-28% of individuals with AD43,44 and also contribute to the dementia syndrome45. However, vascular risk factors may also promote the formation of AD lesions or otherwise contribute to toxic physiological processes. In autopsies of participants of the Honolulu Asia Aging Study, mid-life elevations in systolic and diastolic blood pressure were associated with number of senile plaques and neurofibrillary tangles, respectively46,47. In the absence of dementia, increased plaques and tangles have also been reported in middle-aged hypertensive individuals48. Cholesterol has also been found to promote the accumulation of beta-amyloid49,50.
Hypertension damages blood vessel walls and contributes to atherosclerosis47. When combined with the diminished elasticity of arterial walls, atherosclerosis reduces the brain’s capacity to eliminate beta-amyloid. Normally, beta-amyloid is cleared from brain parenchyma by drainage through the cerebral arteries. A reduction in the elasticity of the arterial walls diminishes the force needed to drain the substance51. Furthermore, damage to arterial walls may also result in a variety of negative consequences including accumulation of amyloid precursor protein (APP), oxidative stress and dysfunction of the blood-brain barrier47.
In addition to increasing the risk for adverse vascular outcomes, diabetes may affect AD risk through other mechanisms. The location of insulin receptors in the hippocampus and temporal cortex implicates insulin’s involvement in long-term potentiation and other memory-related functions52. Normally, insulin crosses the blood-brain barrier and is thought to promote beta-amyloid clearance. In diabetics, however, insulin resistance and hyperinsu- linemia in the periphery can result in reduced brain insulin levels, therefore affecting the rate of beta-amyloid clearance53. However, at autopsy, several studies report no association between diabetes and AD neuropathological markers54,55, but an association with microvascular infarcts instead55. Furthermore, diabetes is associated with the production of advanced glycation endproducts, oxidative stress and inflammation52.
Obesity
Obesity is typically measured by body mass index (BMI), which is a ratio of weight to height or kg to metres squared, waist circumference (a measure of central obesity), or a hip-to-waist ratio53. A number of studies have examined the relation between BMI and risk for dementia (reviewed by Gufstafson56). A majority of the studies indicates an elevated risk for dementia, and specifically for AD, in those with high BMI. Similar to the effects of blood pressure, the relation between BMI and AD appears to vary according to the timing of measurement in relation to dementia onset. The majority of studies examining BMI 10 or more years prior to dementia diagnosis report an increase in risk. For individuals meeting a definition of obese (BMI ≥ 30) the risk of dementia is approximately two-to-five times that of normal weight individuals. A few studies have reported no association or an increase in AD risk with lower BMI. However, most of those studies have used a relatively short follow-up period (e.g. within five years of dementia onset), and this raises the possibility that weight loss in this context is an indicator of incipient dementia rather than a risk factor56.
The relationship between obesity and AD risk may operate through several pathways. First, obesity is a risk factor for a number of other adverse health outcomes including insulin resistance, diabetes and cardiovascular risk factors and conditions, and these in turn are risk factors for AD and vascular dementia53. Second, in the USA, obesity is associated with demographic characteristics that are also associated with AD. These include lower education and socioeconomic status and Hispanic or black race. Third, obesity is associated with higher consumption of dietary fat and calories and lower physical activity levels, which also have been associated with AD53.
Psychosocial Stress
Several studies have reported an association between psychosocial stress and dementia. A prospective, Swedish population-based cohort study of 70 year olds examined the effects of 18 stressful life events experienced in childhood, early adulthood and late adulthood in predicting incident dementia. Death of a parent in childhood and physical illness in a spouse or serious illness in a child in late life were each associated with a 6- to 7-fold increase in dementia risk. Experience of manual labour was associated with a 26-fold increase. The number of risk factors experienced was associated with increasing risk57. Similarly, the Cache County (UT) population study reported a 3-fold increase in dementia risk with early-life death of a father58. This effect was not observed with mother’s death, and it was hypothesized that the association reflected the effects of socioeconomic adversity, limited access to health care and other lost opportunities. Although the outcome examined was all-cause dementia, the predominant form of dementia in this population is AD10,11.
In a case-control study from a large health maintenance organization (HMO) in Washington state, number of siblings was associated with increased risk of AD. The risk increased with each additional child so that having five or more siblings was associated with a 39% increase in risk, seven to nine siblings with a 72% increase, and 10 or more siblings with more than a 2-fold increase in risk59. Residence in the suburbs was associated with a reduction in risk. Drawing from the same HMO database and linking to US Census records and birth certificates, Moceri and colleagues examined the subsequent risk of AD associated with a number of psychosocial factors. Father’s work in unskilled manual labour and number of persons in household were each associated with greater risk. Furthermore, these early life experiences interacted with APOE genotype. Among those with one or more e4 alleles, those whose fathers worked in manual labour had an odds ratio for AD of 2.35 (95% CI = 1.07-5.16), but those without e4 had an odds ratio of 1.40 (95% CI = 0.78-2.52). Compared to individuals with neither the early-life risk factors nor the e4 allele, having father’s work in manual labour, a household size exceeding six members and having an e4 allele was associated with an odds ratio of 14.80 (95% CI = 4.90-46) for AD60. A similar interaction between APOE genotype and early-life risk factors was reported in a large sample of Japanese Americans living in King County, Washington. Here, more than four children in household and smaller head circumference were associated with increased risk of AD among bearers of an APOE s4 allele61.
Other studies have examined the influence of experiences later in life such as loneliness or lack of social support, the latter of which can potentially mitigate the effects of stressful events. Individuals scoring at the 90th percentile of a loneliness questionnaire exhibited a 2-fold increase in risk for AD as compared to those reporting few feelings of loneliness. The elevated risk for loneliness was also associated with more rapid cognitive decline and persisted when controlling for the effects of social network and activity. Interestingly, these effects were independent of the degree of AD neuropathology62.
Additional work has examined personality traits, particularly distress proneness or neuroticism. In a sample of elderly Catholic clergy, distress proneness was associated with increased risk for AD. Because personality traits are considered to be relatively stable, the measure of distress proneness was thought to reflect cumulative, psychological distress experienced over the life span. Relative to those with a low level on this trait, individuals at the 90th percentile exhibited a 2-fold increase in AD risk. The effects remained after statistical control for depressive symptoms and engagement in cognitive activities. Distress proneness was also associated with more rapid cognitive decline, particularly in episodic memory. Again, baseline scores on distress proneness were not associated with degree of AD neuropathology at death63, suggesting that the relationship with dementia works through other mechanisms64.
Activation of the hypothalamic-pituitary-adrenal (HPA) axis has been suggested to underlie the association between psychosocial stress and AD. Broadly speaking, this process mobilizes resources in response to a ‘stressor’ by releasing glucocorticoids that promote energy metabolism and modulate immune functions65. However, with chronic and excessive activation of the HPA axis, high levels of glucocorticoids may lead to cell death in regions of the hippocampus, and this phenomenon in turn can diminish the normal inhibitory feedback loop, leading to further activation and release of gluco- corticoids. This ‘glucocorticoid cascade’ (see Sapolsky66) has been invoked to underlie the hippocampal damage and corresponding cognitive impairment observed with chronic stress in aged organisms66. In relation to AD risk, hippocampal damage from chronic glucocor- ticoid release may functionally reduce the brain’s capacity (reserve) to compensate for the neuronal degeneration that accompanies AD. Alternatively, animal studies suggest that glucocorticoids can directly promote the development of AD-type pathology. In transgenic mouse models of AD, the administration of an experiental stressor or direct glucocorticoid challenge increased beta-amyloid deposition67,68 and tau pathology. Clearly, more research examining the role of stress and physiological changes in the brain is warranted.
Early-life stressors may also have their effects through the limitation of financial resources. Early parental death, lower parental socioeconomic occupations and higher sibling density may limit the financial resources available during critical periods of brain development. Nutritional or environmental deprivation associated with low socioeconomic resources may limit brain growth and maturation, and therefore, neural reserve. Access to health care and educational and vocational opportunities may also be curtailed. In addition, the accessibility of emotional resources may be limited, particularly in the case of early parental death. Such non-normative loss may plausibly result in psychological growth and maturation under less than ideal circumstances, potentially leading to maladaptive coping strategies and stress reactivity, that affect brain development69. Later, in the face of AD pathology, less neural reserve and poor coping strategies may result in a lower threshold for the clinical expression of dementia.
STRATEGIES FOR THE PREVENTION OF AD
Any of the modifiable risk factors discussed above may present a potential target for intervention. The following sections will briefly summarize the literature regarding potential strategies to reduce the risk of AD. Some of these strategies involve pharmacological compounds that theoretically reduce AD-related neuropathology, whereas others are intended to build neural reserve or promote cardiovascular health. None of these potential prevention strategies has been proven efficacious, but a number are of sufficient promise to warrant discussion here. These include anti-inflammatory drugs, lipid-lowering agents, hormone replacement therapy, antioxidants and lifestyle factors including diet, social support and engagement in physical, cognitive and social activities.
Pharmacological Interventions
Non-steroidal anti-inflammatory drugs
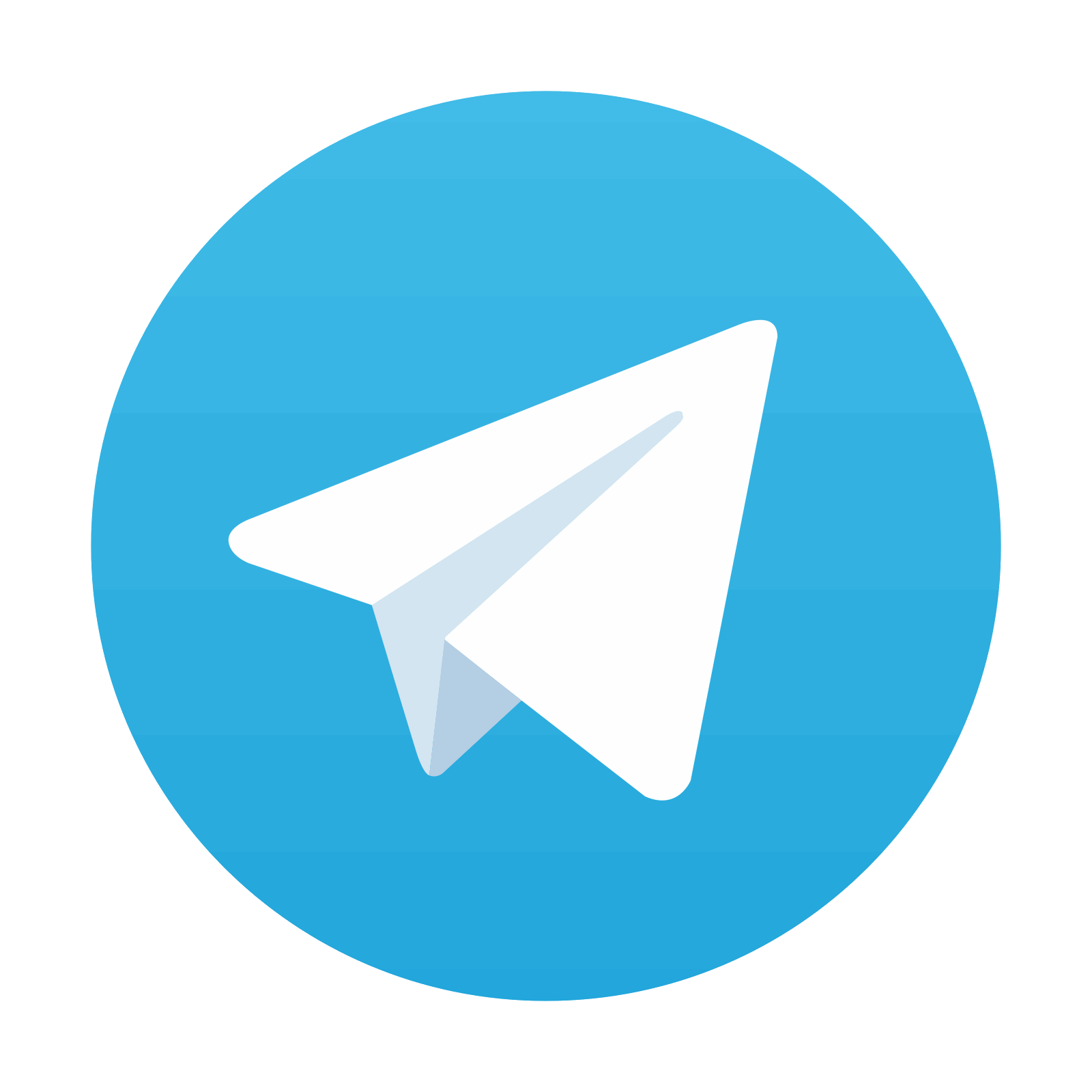
Stay updated, free articles. Join our Telegram channel

Full access? Get Clinical Tree
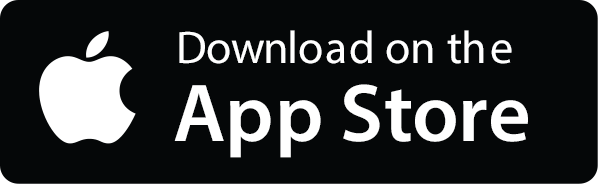
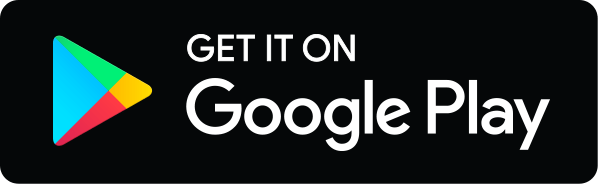