1. A normal healthy patient
2. A patient with mild systemic disease
3. A patient with severe systemic disease
4. A patient with severe systemic disease that is a constant threat to life
5. A moribund patient who is not expected to survive without the operation
6. A declared brain-dead patient whose organs are being removed for donor purposes
If Emergency – add “E” (e.g., 2E)
Other pre-procedure considerations include: assessment of the airway, positioning-related factors, intravenous access, pathophysiology of the surgical site, fluid management, and other issues which may impact on anesthesia medication choices for the procedure and management in the immediate recovery period (e.g., post-traumatic stress, trauma, elderly considerations , chronic alcoholism, or chronic opioid use are some examples). Airway issues, including anticipated difficult intubation or an unstable cervical spine, are extremely important and dictate an awake intubation or other alternative induction and advanced airway intubation methods. As such, the choice of muscle relaxants and sedative drugs may differ. As an example , the lack of intravenous access may necessitate an inhalational agent induction as is often used in children. Another example would be inducing anesthesia for a patient with increased intracranial pressure. In this case, the blood pressure must be maintained high enough to preserve adequate cerebral perfusion pressure. Hence, in general (unless central venous pressure is extremely elevated), the net perfusion of the brain (cerebral perfusion pressure) is the mean arterial pressure minus the intracranial pressure (discussed along with other physiological relationships later in the chapter).
In the preoperative period, the anesthesiologist will usually discuss the planned procedure with the surgeon or proceduralist. Positioning, anticipated blood loss, special procedures (such as deliberate hypotension or hypothermia), and anticipated procedure risks are discussed to plan for anesthesia management and the preparation of ancillary equipment. This is also a time when the IOM team should confer with the surgeon to insure the optimal choice of monitoring modalities. Additionally, a discussion between the entire team is warranted when surgical cases arise that require the IOM team. The anesthesiologist, the IOM team, and the surgeon should communicate before induction regarding optimal anesthetic choices in order to facilitate that monitoring and this communication is a standard of care to ensure the optimal patient outcome. In particular, the discussion between the IOM team and anesthesiologist should identify when the initial baselines need to be acquired, especially immediately post intubation or prepositioning, so that the induction can be planned with this consideration. It is important to recognize that the final choice of anesthetic medications may be a compromise between that which may appear optimal to the IOM team and that which may be dictated by medical and patient considerations identified preoperatively. Not infrequently, this discussion may result in a bargaining where the best compromise can be made . Once baselines are acquired, the anesthesia choice may be further adjusted based on how the patient is responding vis-à-vis hemodynamics and IOM.
Preoperative Discussion with the Anesthesiologist
The discussion with the anesthesiologist must take into consideration the experience and training of the specific practitioner since they may need to consult with a colleague. The anesthetic is usually delivered and monitored by either a solo practitioner or by an “anesthesia care team .” Solo practitioners include physician anesthesiologists or certified registered nurse anesthetists (CRNA) . The care team model includes an in-room practitioner such as a CRNA , anesthesia resident physician trainee, student registered nurse anesthetist (SRNA) , or an anesthesia assistant (AA) with anesthesiologist oversight. Physician anesthesiologists are individuals with a medical degree (MD or DO) who have completed a focused residency in anesthesiology and may have advanced training (fellowship) in subspecialty areas (e.g., neurosurgical, thoracic, cardiac, pediatric, or obstetric anesthesia). A CRNA is an advanced practice nurse with a master’s degree who has undergone specific training in anesthesia after nursing practice, typically in intensive care environments and may work with a physician anesthesiologist or, in some circumstances , as a solo practitioner. Some CRNAs may have advanced degrees such as a doctorate in nursing practice (DNP) . An AA has a masters level education with specific anesthesia training in the classroom and practical course work. They can act as physician extenders who always work under the direction of physician anesthesiologists. Since their background, training, and experience will vary, each of these individuals may be familiar with the anesthesia considerations for IOM so preoperative discussion, especially the day before the procedure if possible, will be important for their planning.
Types of Anesthesia
Important for discussion with the anesthesiologist is to understand the terms used with regard to the delivery of anesthesia (in particular the two ways the term “MAC” is used). First, although general anesthesia is usually used with IOM, anesthesia is also delivered in other ways. “Local MAC ” is used to describe the use of intravenous medications (e.g., propofol, midazolam, etc.) in patients where local anesthesia is used (often by the surgeon or proceduralist) to anesthetize a limited region of the body. In this case, the term “MAC” stands for “monitored anesthesia care ” and should not be confused with the similar term “MAC” used to describe the potency of inhalational anesthetics (see below). One of the goals of an anesthetic medication is sedation and usually amnesia. Blocking of noxious stimuli (such from the procedure) is primarily done by the use of local anesthesia. IOM is usually not utilized with monitored anesthesia care; however, electrocorticography and mapping of the motor and speech cortex is done by a specialized form of MAC when patients have “awake” craniotomies where local anesthesia is used on the scalp to allow a craniotomy (the exposed brain is insensitive) [1].
“Regional” anesthesia is used to describe the delivery of anesthesia where only a part of the body is made completely insensitive to the surgical stimulation. For example, the patient may have local anesthesia injected to anesthetize specific nerves or plexus (such as a brachial plexus block anesthetizing an arm) or injected into the spinal or epidural space (“neuraxial” anesthesia ). Similar to local MAC, sedation may be such that the patient becomes responsive to stimuli in a continuum from awake to unresponsive. Intravenous medications are used to produce sedation and usually amnesia. Blocking of noxious stimuli (such as from the procedure) is primarily done by the regional blockade. IOM is usually not possible with the regional blockade of major neural structures as the local anesthetics used usually render the neural structures unable to conduct IOM signals. However, when regional anesthesia is conducted to anesthetize regions allowing access to structures for a procedure and the neural structures being monitored are not anesthetized, IOM can be used as long as the IOM technique is not painful. A good example is the use of electroencephalography during carotid endarterectomy where a cervical plexus regional blockade is often used to allow surgical access to the carotid artery.
General anesthesia (GA) is the anesthesia usually used during most procedures where IOM is used. Typically, several medications are used together to produce a more comprehensive anesthetic, which involves four major anesthetic goals. First, medications are given to produce a state of unconsciousness where the patient does not respond to stimuli . Second, medications are delivered to ensure that the patient has no awareness or recall (amnesia) during the procedure. Third, the anesthesia medications are used for analgesia and to block noxious stimuli from activating the nervous system. This goal is often termed anti-nociception and differs from Local MAC and regional anesthesia where local or regional anesthesia is primarily responsible for this aspect. Preventing awareness and recall is a major concern of anesthesiologists and is a major concern in designing an intravenous anesthetic.
Fourth, medications are used in GA to block movement (e.g., muscle relaxation) of the patient in response to stimuli. Although this can be accomplished using medications which block transmission at the neuromuscular junction, the anesthetics used for most cases where IOM is performed involve using agents to block the reflex transmission of sensory stimuli through the spinal cord producing peripheral motor activity. This reflex pathway involves afferent sensory input from the periphery, synaptic connections within the spinal gray matter, efferent motor pathways, and descending influences from the brainstem and cortex.
It is important to differentiate the reflex movement through the spinal cord pathways in the anesthetized, unconscious patient from the voluntary movement of an unanesthetized, awake patient. The comment “the patient is awake” when they move under anesthesia is usually incorrect since the motion is due to reflex activity in the inadequately anesthetized spinal cord from peripheral stimuli. This need for immobility is one of the major challenges and concerns for the anesthesiologist during procedures involving IOM when complete muscle relaxation must be avoided. The challenge for the anesthesiologist is that parts of the same pathway are used for reflex movement and for desired muscle activity from transcranial motor cortex stimulation (MEP) . This suggests that the titration of effect in the efferent components (spinal gray matter and peripheral nerve) must be a delicate balance to facilitate the MEP but inhibit the reflex activity. This also stresses the need for good antinociception at the spinal level to further block the afferent limb of the reflex movement pathway.
Each of these anesthetic goals can be accomplished using a mixture of agents acting at different neural sites. It is currently believed that anesthetic agents act through their interaction with specific synaptic receptors. The two major categories of action include facilitating the inhibitory effect of gamma-amino-butyric acid (GABA) by actions at the GABAa receptor , and the inhibition of the major excitatory synapse at the N-methyl-D-aspartate (NMDA) receptor [2]. Other synaptic targets include the neuronal acetylcholine receptor (nACh) , the mu opioid receptor , central alpha2 receptors , and the potassium , calcium and glycine channels [2]. Drugs differ by respective major synaptic targets as well as different subunits of the receptors and the distribution of these receptors throughout the central nervous system. As such, the anesthetic plan is usually developed taking into consideration these goals, the procedural goals (including IOM), and the specific medical issues for each patient. The plan is usually designed for the different phases of general anesthesia: premedication, induction, maintenance, emergence, and recovery.
Phases of Anesthesia
Premedication is the use of medications, usually prior to bringing the patient to the procedure room, to begin an anesthetic effect . Frequently, the patients may receive a small dose of midazolam which contributes to sedation and amnesia. Patients may also receive a short-acting analgesic, such as an opioid , if they are having pain and long-acting analgesics (often non-opioids) to provide postoperative pain reduction, especially in patients with chronic pain. Other medications may also be given to produce various pharmacologic effects such as anti-emesis or antibiotics to reduce perioperative infection. In general, these medications usually have minimal effects on IOM.
Induction is the phase of anesthesia after the patient is brought to the procedure room and basic anesthesia monitoring established (e.g., electrocardiogram, blood pressure, temperature, and pulse oxygen saturation). The doses of induction medications are rather large because of the needed initial cortical and brainstem drug effects, hence the loss of the cranial nerve-mediated blink reflex to eyelash stimulation is used to identify adequate brainstem drug effect. They also serve to load the patient with medications to start accomplishing the anesthesia goals and cover the gap until the maintenance medications are established. Unless an awake intubation or an inhalational mask induction is planned, induction usually includes a sedative drug like propofol , an opioid , and a muscle relaxant to facilitate intubation. These bolus doses would normally preclude IOM monitoring during induction; however, the time needed to establish IOM is such that most of the effects of the induction drugs will resolve except for muscle relaxant effects if longer acting agents are chosen.
If an awake intubation is chosen, the doses of sedative agents and opioids are usually smaller such that a clinical neurological assessment is often possible, and IOM can be established sooner. Generally, muscle relaxants are not needed for an awake intubation but topical application of local anesthetics to the airway may preclude assessment of electromyography of the vocalis muscle.
If an inhalational “mask” induction is chosen , the patient is asked to breathe sevoflurane with or without nitrous oxide. After the patient is asleep and an intravenous line established, the sevoflurane can be reduced and the anesthetic converted to the desired maintenance agents. Since the dose of sevoflurane is rather large, IOM will be affected until the agent has been reduced in the nervous system, which will be somewhat longer than the decrease of the concentration in the breathing circuit as shown on the anesthesia monitoring systems.
Once induction is accomplished , anesthesia is converted to the maintenance phase. Two basic types of anesthetic agents are used for maintenance: inhalational and intravenous. Further, combinations of agents are often used in order to accomplish the four main anesthetic goals described above. The choice of these agents has a major impact on IOM such that the integration of patient needs, procedure needs , and IOM considerations must be balanced. During the maintenance phase, slow changes in IOM are typically observed. Although referred to as “anesthetic fade” this may relate to a variety of pharmacologic and other factors [3].
At the conclusion of the maintenance phase is emergence. This is the period when the anesthesia is reduced until the patient awakens and is extubated. This requires the resumption of spontaneous breathing and the return of protective airway reflexes. The goal is to have the patient safely awaken so they have a stable physiology and can be transferred to the care of a nurse in the recovery phase in the postoperative care unit. Aside from insuring adequate pain relief and minimizing the chance of nausea and vomiting , the major goal is having the patient awaken promptly to allow a neurological examination to identify problems. Since intravenous anesthetic agents may take longer to eliminate than inhalational agents, the intravenous agents may be reduced during the latter part of the maintenance phase, often after the conclusion of the procedure and during the closure of the wound, which may result is some associated IOM changes.
Specific Anesthesia Drugs Used for Maintenance
Based on the synaptic targets involved with individual agents, each anesthetic agent contributes differently to the four goals of anesthesia. For example, at clinically (or surgically) equivalent depths of anesthesia, some agents may produce a different spectrum of cortical or spinal cord evoked potential depression than other agents. The differences between drugs may be explained by differing profiles on receptor types (e.g., GABA, NMDA, etc.), differing location of drug action (i.e., pre- or post-synaptic effects), the effects on individual subtypes of these receptors, and the anatomic distribution of the receptors and subtypes . The differences between drugs also contribute to different profiles of drug action on monitored responses.
Halogenated Inhalational Agents
The halogenated inhalational anesthetic agents (isoflurane, sevoflurane, desflurane) are the most frequently utilized agents in GA when IOM considerations are not present. These drugs have a broad action on neural structures with excellent cortical effects on actions of unconsciousness and amnesia (action at the GABAa receptor), excellent spinal cord effects leading to immobility (effect at the glycine receptor), some contribution to antinociception (actions at the NMDA, nACh, sodium, and potassium channels), and some muscle relaxation effects (from action at the nACh receptor in the neuromuscular junction). These actions make them very versatile agents that provide excellent coverage for amnesia and immobility which are major concerns of anesthesiologists.
Properties of inhalational anesthetics
Agent | Adult MAC (vol%) | Blood: gas partition coefficient |
---|---|---|
Isoflurane (Forane®) | 1.15% | 1.4 |
Sevoflurane (Ultane®) | 2.1% | 0.68 |
Desflurane (Suprane®) | 6% | 0.42 |
Nitrous oxide | 105% | 0.46 |
In general, since the different agents act through similar neural mechanisms, the agents are thought to be equivalent when compared at concentrations of similar MAC value. Hence, 1 MAC of Desflurane is considered approximately equivalent to 1 MAC of Sevoflurane such that substituting one for the other (such as for pungency or solubility) should have similar effects.
The second property, solubility in tissues , is the basis for the time it takes to raise and to lower the anesthetic effect in the body. This is reflected in the blood: gas partition coefficient, where a higher coefficient is a more soluble agent (see Table 5.2). Although raising and lowering depends on the mechanics of the breathing pattern and cardiac output, a drug which is least soluble (e.g., desflurane) has its concentration in the brain rise the quickest and reduces the quickest when the delivery is stopped. For this reason, many anesthetists favor desflurane (quickest) or sevoflurane (second quickest) over isoflurane (least quick) when wanting to use an agent with IOM that might need to be eliminated because of excessive IOM effects.
Finally, pungency is the property of irritation of the lung when breathing the agent. Of these agents, sevoflurane and nitrous oxide are the least irritating so that if anesthesia needs to be induced by having the patient breathe the agent (i.e., there is no intravenous line), sevoflurane (with or without nitrous oxide) will be the choice. Isoflurane and desflurane are simply too irritating to be practical for mask induction.
Since the halogenated agents have broad actions at many synaptic targets, they provide a model for understanding the effect of anesthetic agents on IOM modalities. In summary, since the effects occur at synapses, the location of synapses in the IOM pathway will help explain the resultant effects [6].
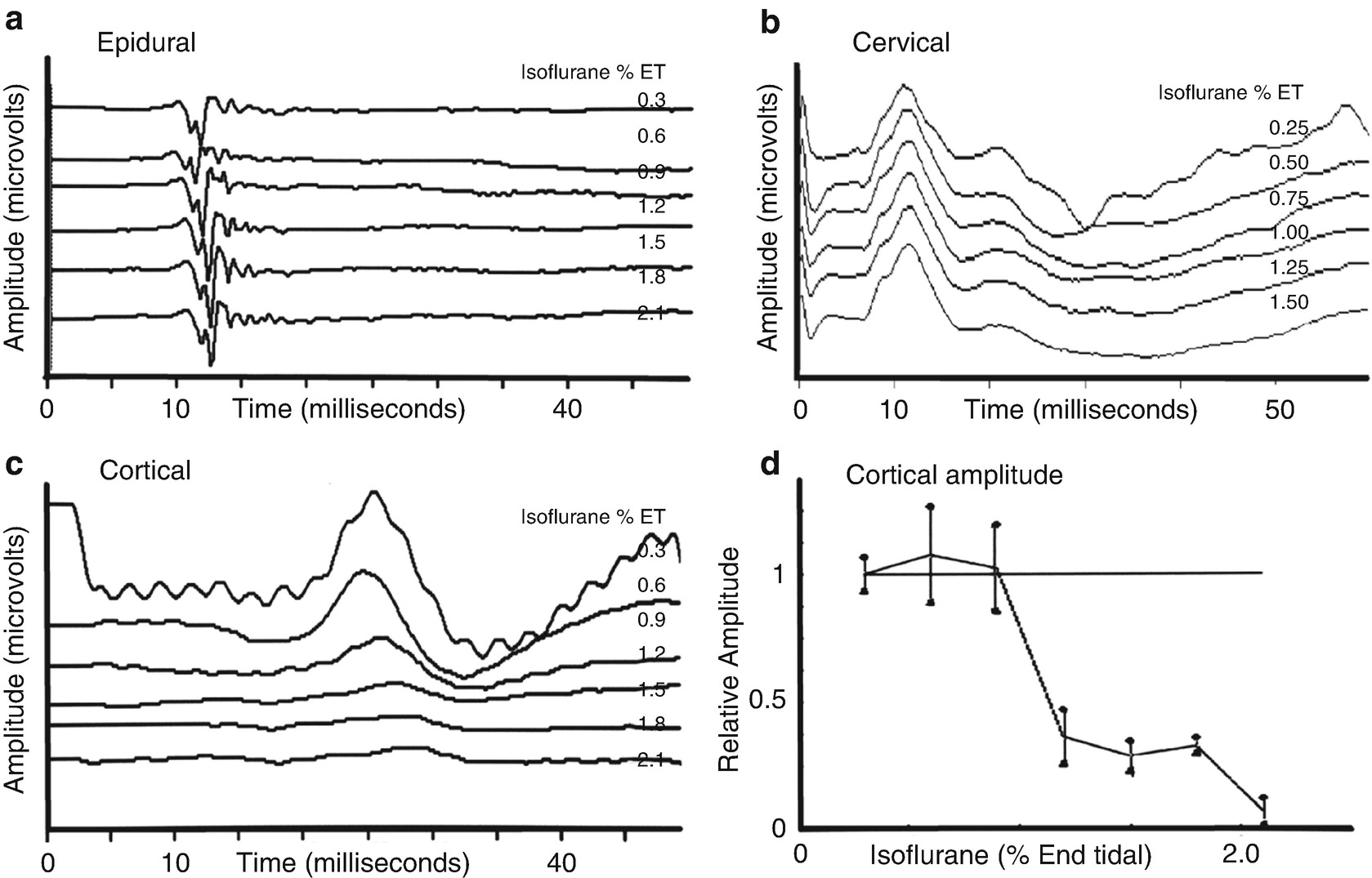
Effect of isoflurane on SSEP recordings. Changes in lower extremity somatosensory evoked potentials recorded at several locations with increasing concentrations of isoflurane in the baboon. (a) Shows recordings from the epidural space that indicates minimal effects. (b) Similarly shows minimal effects in the response recorded over the cervical spine. (c) Shows a prominent effect on the response recorded over the somatosensory cortex. (d) Shows a plot of the amplitude of the cortical response demonstrating a nonlinear amplitude reduction as the isoflurane concentration is increased. (Reproduced with permission from Sloan [6])
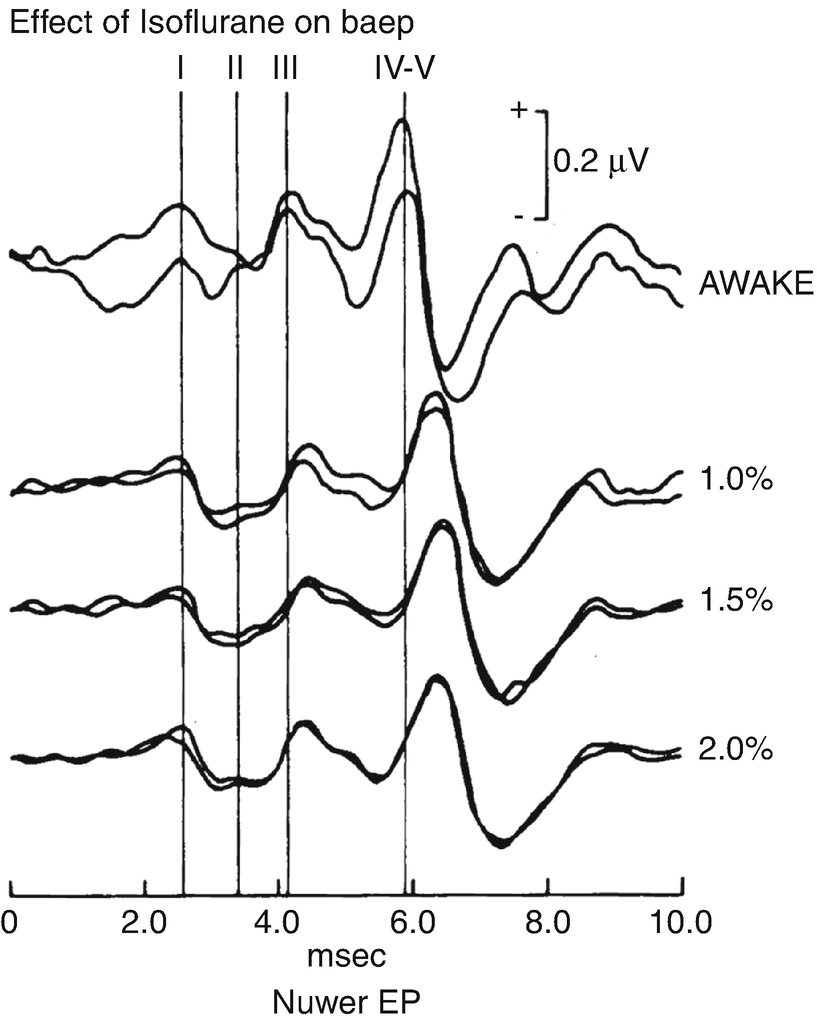
Effect of isoflurane on auditory brainstem response. Influence of isoflurane on auditory brainstem response (ABR) . Latency of peaks III and IV-V are progressively increased with increases in isoflurane . The effect on IV-V is more than on III. (Reproduced with permission from Manninen et al. [8])
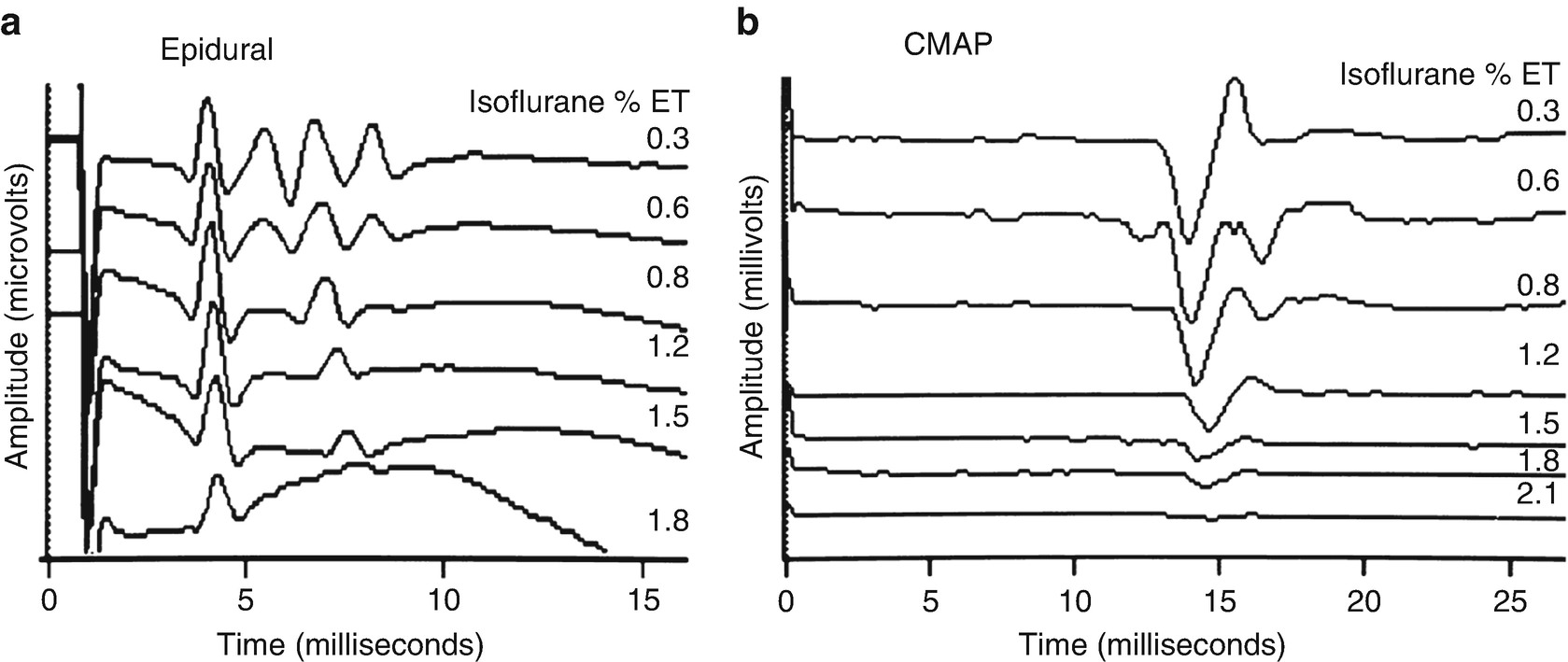
Effect of isoflurane on motor evoked potentials. Changes in transcranial motor evoked potentials recorded in the epidural space (a) and in compound muscle action potentials (CMAPs) in the hand (b) in the baboon. Shown is the maintenance of the single D wave (“D”) and loss of the multiple I waves (“I”) in the epidural recording and loss of the CMAP response with increasing concentrations of isoflurane . (Reproduced with permission from Sloan [9])
The second location of synapses in the motor pathway is in the spinal cord gray matter where descending motor pathways activate peripheral motor nerves. At this location the number of synapses varies with the specific muscles ; however, the more proximal muscles have more synapses suggesting why the more distal muscles in the limbs may provide the best-recording sites during anesthesia.
The production of peripheral motor responses results from the cumulative effect (temporal summation) of D and I waves and anterior horn cells. As such, the loss of I waves explains why the single pulse transcranial stimulation of the electrical or magnetic technique is so exquisitely sensitive to anesthesia and why the high-frequency multipulse technique is more successful since it produces multiple D waves that can summate more successfully. However, anesthetic effects at these spinal gray synapses can block the production of peripheral motor responses. In addition, a variety of other descending tracts (descending suprasegmental systems [corticospinal, rubrospinal, vestibulospinal, and reticulospinal systems] and propriospinal systems) influence the excitability of the anterior horn cells such that anesthetic effects on these pathways may also hamper the production of muscle responses . Thus, the cumulative effect of the cortical and spinal synaptic effects may explain why the muscle responses from transcranial stimulation are so easily affected by anesthetic agents.
It is of interest to note that the effects of the anesthetic agents on the spinal gray matter forms one of the anesthetic challenges for the choice of agents. Clearly, the ability of a descending motor pathway impulse to produce a motor nerve response or the muscle response follows the same pathway which needs to be blocked to prevent patient movement in response to peripheral noxious stimuli such as surgery. Thus, a balance of effects is needed and contributions of anesthetic agents, which block noxious stimuli coming into the spinal cord, are important to block the reflex pathway leading to immobility . Perhaps the profound effect of the halogenated agents on the reflex activity mediated through the glycine channels explains why this balance is difficult to achieve in their presence.
Similar to the SSEP, the MEP response also shows a nonlinear loss over a narrow anesthetic concentration. This means that some individuals will have muscle responses that can be acquired with 0.5 MAC of an inhalational agent , but most will require a careful titration of intravenous agents (TIVA). For these many reasons, the anesthetic effects on the motor pathway make the muscle responses of the transcranial motor evoked potential one of the most difficult monitoring techniques under anesthesia.
The anesthetic effect of the halogenated agents in the spinal cord accounts for the depression of the H reflex. Studies of the anesthetic effects on the H reflex show that it parallels the movement to noxious stimuli used to measure MAC of the halogenated agents. Thus the anesthetic effects on the motor evoked potentials will mirror the effects on the H reflex [10].
There is one additional synapse in the motor pathway located at the neuromuscular junction. Although the inhalational agents do have effects at this location, the effects do not appear to be clinically significant in the absence of neuromuscular blocking agents (NMBA) . However, the effect of the NMBAs is known to be amplified by halogenated inhalational agents such that the NMBA management must be carefully monitored in their presence such that the motor response is not excessively depressed. Fortunately, the only anesthetic agents that impact the muscle responses from peripheral nerve stimulation are neuromuscular blocking agents and local anesthetics blocking conduction in the nerve.
In summary, the halogenated inhalational agents have a broad spectrum of anesthetic effects (i.e., multiple synaptic targets) that provide excellent cortical effects on consciousness and amnesia, and excellent effects on the spinal gray matter producing immobility such that they are superb anesthetics when IOM is not being utilized. Since they have less profound antinociception, they are often supplemented with opioids creating what is often termed a “balanced” anesthetic. However, because of the profound depression of the SSEP and MEP, these halogenated agents must often be restricted or avoided during IOM and other agents utilized.
Nitrous Oxide
Nitrous oxide is also an inhaled agent. Nitrous oxide (N2O) is different from the halogenated agents consistent with anesthetic action at different synapses. N2O is particularly effective in antinociception due to its action at the NMDA receptor with additional actions at the mu opioid, nACh, and potassium channels. In addition, it contributes to unconsciousness and amnesia through minor actions at the GABAa and central alpha2 receptors and contributes to immobility through minor actions at the glycine receptor [11]. In summary, it has excellent qualities in blocking noxious stimuli but weak properties in producing cortical effects (unconsciousness and amnesia) and immobility. This makes it a nice complement to the halogenated agents and explains the logical combination of the two classes in general anesthesia.
The effects of nitrous oxide on the SSEP and MEP are similar to the halogenated agents. However, the potency of N2O (MAC >100%) limits this effect. When compared at equi-MAC anesthetic concentrations, nitrous oxide produces more profound changes in the muscle recordings of motor evoked potentials than the halogenated inhalational anesthetic agents [12]. Some studies have suggested that similar to low concentrations of the halogenated agents, nitrous oxide may be acceptable for MEP monitoring with multipulse stimulation techniques; however, the other anesthetics used with it make a difference in the degree of depression [13–15]. As such, if only one inhaled agent is to be used many anesthesiologists would prefer the contribution of the halogenated agents to the cortical and immobility effects rather than the contribution of nitrous oxide to antinociception (which could be accomplished using opioids with less impact on the responses).
Unfortunately for IOM, the combination is synergistic such that the depression of the combination is more profound than would be predicted by the effect of the individual agents [16]. Thus, it is not recommended to use a combination of halogenated agents and nitrous oxide with IOM.
Intravenous Anesthetic Agents
Since the inhaled agents may need to be reduced in concentration (or avoided) in some patients during some IOM modalities (notably transcranial motor evoked potentials and cortical somatosensory evoked potentials), anesthesia maintenance may require the use of intravenous anesthetic agents since these are often more compatible with accomplishing the four anesthesia goals and facilitating IOM. The intravenous agents are usually chosen such that the mixture of agents accomplishes the goals of anesthesia while minimizing the impact on the IOM responses. When general anesthesia is provided solely by intravenous agents it is termed total intravenous anesthesia (TIVA) .
Agents Used to Produce Unconsciousness and Amnesia
Commonly used sedative, hypnotic, and amnestic intravenous agents
Agent | Trade name |
---|---|
Propofol | Diprovan® |
Etomidate | Amidate® |
Dexmedetomidine | Precedex® |
Midazolam | Versed® |
Propofol
Of these agents, propofol is currently the most commonly utilized sedative and amnestic agent. Propofol has potent effects via actions at the GABAa receptor which increases the inhibitory effects of GABA, acts at extrasynaptic GABA receptors and has some action at neuronal nACh receptors. This action at the GABAa and minor effects at the glycine receptor in the spinal cord contributes to immobility during anesthesia. Finally, it makes minor contributions to antinociception through minor actions at the glycine and nACh receptors. At the spinal cord level, the dose -response curve for reflex movement is substantially flattened compared to the halogenated agents such that a dose can usually be found that provides adequate suppression of movement without the depression of the MEP seen at higher doses [17].
Propofol does have depressant effects similar to the halogenated inhalational agents. For this reason (as with all anesthetic agents) a constant infusion is required to prevent sudden changes in drug concentration that depress the IOM responses simulating neural compromise. Usually, a dose can be chosen which produces the desired anesthetic effects without excessive depression of the IOM responses.
At higher doses it will block the responses such that if they are needed for an individual patient, other agents may need to be added to accomplish the cortical effects and reduce the propofol dosage . One of these agents is ketamine which is discussed below [18]. A second, less commonly used agent is an infusion of lidocaine which also reduces the dose of agents used for antinociception [19, 20]. A newer version of propofol named fospropofol does not appear to have any advantages over propofol [21].
Propofol is used as a common induction agent for anesthesia (usually in doses of 1–2 mg/kg). With TIVA, the initial bolus dose is needed to get the blood level up to the needed dose so that a subsequent infusion can keep the needed effect level. That infusion is designed to match the metabolism or clearance of the drug so that a constant drug level is maintained to provide the needed level of anesthesia and a constant level of drug effect on the monitoring. In general, this infusion rate will vary with the patient needs but is usually 120–180 micrograms per kilogram per minute when it is used solely with an opioid in TIVA. The infusion may be higher in patients who need a higher effective blood level because of drug tolerance from preoperative medication usage, or maybe lower when other medications are added to the TIVA that also provide some sedative-hypnotic effects (e.g., inhalational agents or lidocaine infusions). As with all of the anesthetic agents, a constant level is important to minimize acute changes in the monitoring. Slow awakening can often be seen with prolonged infusions due to increasing context-sensitive half-time so decreasing the infusion rate near the end of the procedure may be used.
Etomidate
An alternative sedative/hypnotic to propofol is etomidate, which also has potent effects on unconsciousness and amnesia via actions at the GABAa receptor. An advantage is that etomidate has limited cardiopulmonary depressant effects and has a role during induction in selected patients. It contributes to immobility via actions at the GABAa and glycine receptors and has some minor contributions to antinociception through actions on the potassium channels. Many practitioners have avoided etomidate in infusion over time because of concerns of worsened outcome in patients with sepsis secondary to the depression of corticosteroid production in the adrenal gland [22, 23].
Etomidate is unusual because at clinically useful doses it enhances the EEG and increases the amplitude of both sensory and motor evoked responses [24–29]. This enhancement may produce seizures in patients with epilepsy; the combined effect of enhanced activity of epileptic foci and transcranial electrical stimulation is unknown [30].
Etomidate is delivered like propofol in bolus doses for induction and by infusions for TIVA. Its use for induction as a bolus (typically 0.2–0.3 mg/kg) is often favored in patients who may be dehydrated, in the elderly, have poor myocardial function, or who may be hemodynamically unstable because it has less depressant effects on the heart . Like propofol, the induction bolus can achieve effective blood concentration so that a subsequent infusion can maintain the effective level for anesthesia. At present, infusions of etomidate in TIVA are limited as indicated related to adrenal suppression . Current research with chemical relatives (e.g., methyl-carbonyl etomidate) may hold promise for an alternative without adrenal depression in the future [21].
Benzodiazepines
Prior to the introduction of propofol (and prior to MEP monitoring), an infusion of midazolam was used with TIVA [25, 31]. The benzodiazepines , notably midazolam, also act at the GABAa receptor producing amnesia at doses that are not associated with unconsciousness . This produces a mild depression of cortical SSEP and as a premedicant or occasional supplement in anesthesia allows MEP recording [28, 31–36]. In addition to possible cortical locations for the benzodiazepine effect, an effect at the spinal cord has been described as antinociceptive through actions at the GABA receptors in lamina I and II in the dorsal horn [37, 38].
The superb anxiolytic and amnestic qualities make midazolam an excellent addition to anesthesia but a prolonged drug half-life makes it less favorable than propofol for a TIVA infusion -based anesthetic. As such midazolam is frequently given preoperatively for reduction of anxiety (anxiolotic) and as a method to help insure amnesia when concerns are raised about the possibility of awareness. These small doses, given intermittently, do not appear to have a detrimental effect on monitoring; however, higher doses have been associated with MEP depression . It is also an excellent agent to add at the end of a procedure if delirium on awakening is anticipated or to mitigate hallucinatory effects of ketamine (see below).
Dexmedetomidine
One of the newer additions to the sedative agents is dexmedetomidine which acts as a central, selective alpha2 adrenoreceptor agonist drug. It has been shown to reduce the amount of propofol , opioids, and halogenated inhalational agents needed during maintenance [39]. The sedative drug effect is primarily due to action in the brainstem (locus coeruleus) which decreases cortical arousal influences producing sleep similar to normal sleep. Although not FDA approved at present for use in general anesthesia, it is approved for sedation in the intensive care unit where patient awakening allows a less effected neurological exam. Of note, it does not appear to have amnestic action. Side effects of hypotension and bradycardia occur because of effects on the brainstem limit the drug to a role as a supplement to other anesthetic agents.
Dexmedetomidine has “opioid-sparing” properties and appears to be an excellent supplement in the opioid-tolerant patient. The effects on SSEP recordings are minimal but, as with propofol, higher blood levels of dexmedetomidine (or when moderate dosages of other agents such as propofol are used with dexmedetomidine) inhibit MEP monitoring making its use challenging [40, 41].
Dexmedetomidine is not used as a sole agent in TIVA for two reasons. First, its dose is limited because it reduces the sympathetic influences from the brainstem which results in bradycardia and hypotension. Second, at acceptable doses, it does not produce adequate antinociception and amnesia, so these effects must be provided by other medications. Hence, TIVA can be accomplished by dexmedetomidine infusion supplemented by low dose propofol for amnesia and an opioid infusion for antinociception. Alternatively , a low-dose inhalational agent may be used instead of the propofol to provide the amnestic action. Often these combinations will be acceptable for SSEP recording but, if higher doses of dexmedetomidine or propofol are used , MEP monitoring may not be possible.
Barbiturates
Although not fully explored , infusions of methohexital (Brevitol®) have been used during IOM [42]. This may be a viable alternative if the other agents are not usable.
Intravenous Agents Used to Block Noxious Sensory: Antinociception
Commonly used intravenous agents for antinociception
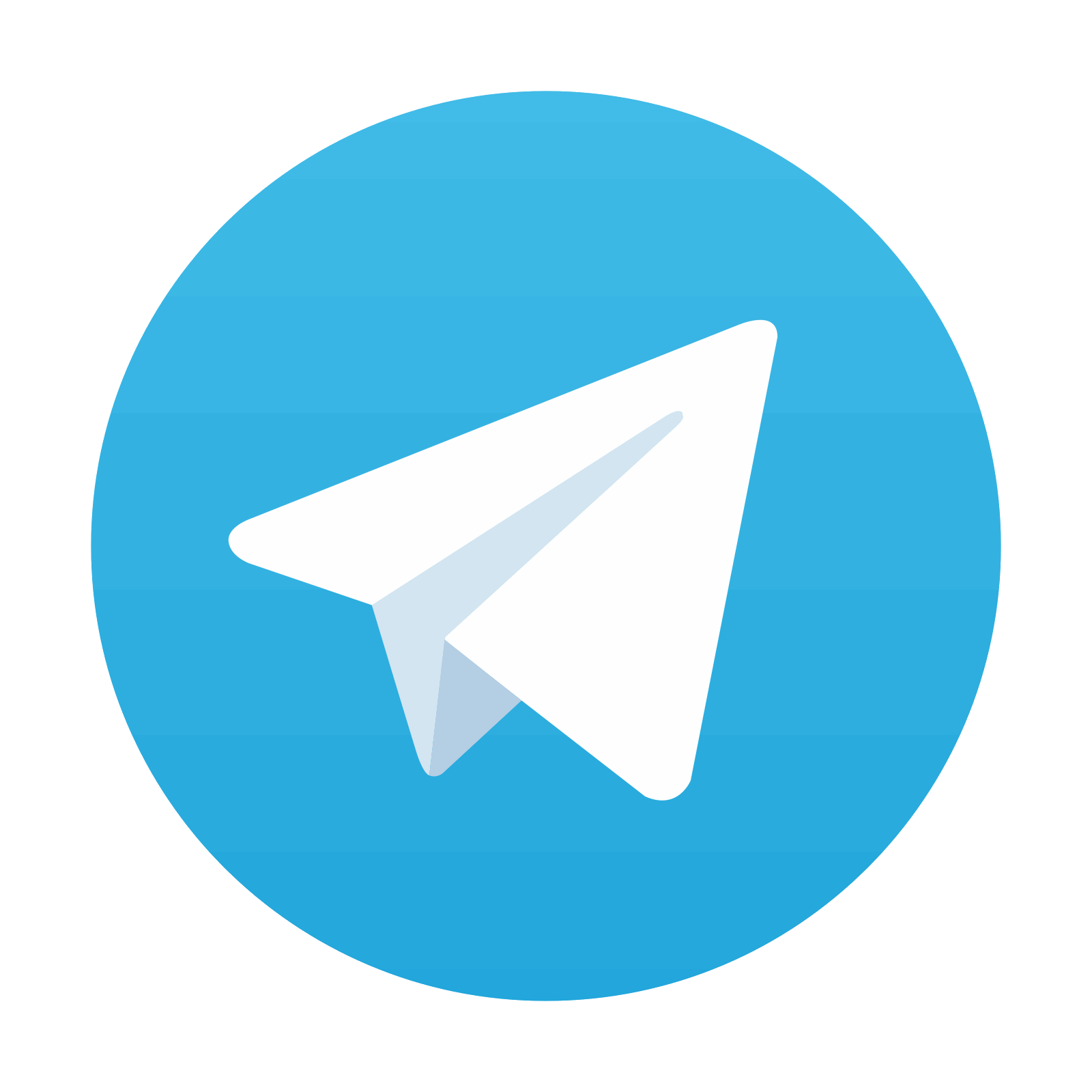
Stay updated, free articles. Join our Telegram channel

Full access? Get Clinical Tree
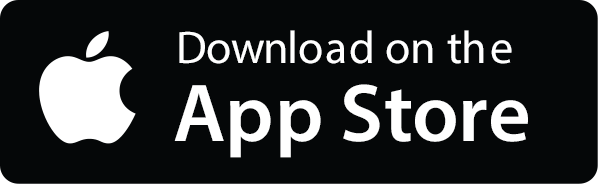
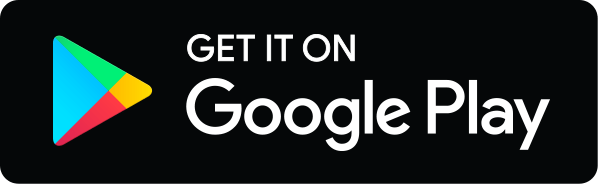