Approaches to Extracranial and Intracranial Dissection
Pearls
Dissection of the intracranial and extracranial vasculature is deemed spontaneous if no evidence of preceding trauma exists.
Extracranial carotid artery dissection represents the etiology of stroke in less than 1% of all cases.1 , 2
Regardless of the mechanism of injury, the majority of arterial dissections will heal within 3 to 6 months of medical therapy. Luminal stenosis completely resolves in 90% of cases over this time.3
Ruptured intracranial pseudoaneurysms involving the vertebrobasilar system have a high rerupture rate in the acute period.
Intracranial dissecting pseudoaneurysms of the vertebral artery represent approximately 28% of posterior circulation aneurysms and 3.3% of all intracranial aneurysms.
Representing the etiology of stroke in less than 1% of all cases, extracranial carotid artery dissection is a relatively uncommon cause of cerebral infarction. However, dissections are a significant cause of stroke in the young, accounting for 10 to 25% of strokes in those aged 25 to 45 years.1 The yearly incidence of spontaneous carotid dissection is approximately 2.6 per 100,000, whereas that of vertebral artery dissection is 1 to 1.5 per 100,000.4 , 5 Untreated carotid artery dissections are associated with a mortality rate of 20 to 40% and a morbidity rate of approximately 40 to 80%.6 Dissection is deemed spontaneous if no evidence of preceding trauma exists, and fibromuscular dysplasia (FMD) is present in up to 15% of these patients.7 One percent to 5% of spontaneous dissections of the carotid or vertebral arteries are diagnosed in patients with other connective tissue diseases, such as Ehlers-Danlos syndrome type IV, Marfan’s syndrome, autosomal-dominant adult polycystic kidney disease, and osteogenesis imperfecta type I.8 , 9 When the cause of spontaneous dissection is unclear, the underlying etiology is usually a summation of multiple vascular risk factors, including, hypertension, diabetes mellitus, smoking, hyperlipidemia, and the use of oral contraceptives.10 – 12
In the setting of multisystem trauma, a dissection may be easily overlooked due to multiple factors contributing to a neurologic deficit on examination.13 Traumatic dissections may be secondary to either blunt or penetrating injury to the head or neck. Blunt trauma is the more common mechanism for both carotid and vertebral dissections. Sudden impact resulting in rapid deceleration and hyperextension, such as that seen in motor vehicle accidents, may stretch the internal carotid artery over the lateral masses of the cervical vertebrae, resulting in dissection. Hyperflexion of the cervical spine has also been implicated in carotid dissection, as the artery may be compressed between the mandible and spinal column.14 Vertebral artery dissections are associated with any mechanism that applies excessive rotational forces, distraction, or flexion-extension to the cervical spine. The vertebral arteries are placed at risk of dissection when trauma to the cervical spine results in fractures of the transverse foramina or facet dislocation. Arterial dissection may also be secondary to a relatively minor blunt force, such as that experienced in chiropractic manipulation of the neck.15 , 16
Penetrating injuries represent a less common cause of traumatic carotid and vertebral artery dissections and may be due to physical violence, such as stab and gunshot wounds to the head and neck. Additionally, iatrogenic dissections are well-known complications of neurosurgical procedures. Cerebral angiography may result in cervical or intracranial dissections, as the catheters and guidewires used to navigate the vasculature may disrupt the endothelium, leading to the formation of an intimal flap. Multiple spine procedures, including cervical lateral mass screw placement and occipital-cervical fusions, place the vertebral arteries at risk of injury and dissection.
♦ Pathophysiology and Natural History
Arterial dissections are the result of longitudinal division of the vessel wall by the entrance of blood into the tunica media. The inciting event is debatable, either being the result of an intimal tear that grants access to intraluminal blood or a direct bleed from the vasa vasorum, which forces blood into the vessel wall. Regardless of the inciting event, the expanding diameter of the vessel wall results in partial or complete occlusion of the true vessel lumen. The false lumen may remain patent and act as a conduit for continued blood flow.17 Exposure of the subendothelial layer also brings the prothrombotic components of the vessel wall into contact with intraluminal blood, resulting in the formation of thrombus and a source of potential embolization18 (Figs. 36.1 and 36.2).
In Sandmann et al’s19 review of 200 patients with spontaneous cervical arterial dissections, the internal carotid artery was involved in 76% of patients. Of these patients, 62% experienced unilateral dissection, whereas 14% suffered bilateral dissections. The internal carotid and vertebral arteries were involved simultaneously in 6% of patients. Spontaneous internal carotid artery dissection most commonly occurs in the cervical segment 2 cm distal to the carotid bulb. The length of the dissected segment is variable, but the distal extent usually does not progress beyond the entry of the internal carotid into the petrous bone. The vertebral arteries most commonly dissect at the level of C1 and C2.
Cerebral infarction may occur at the time of dissection; however, approximately two thirds of strokes occur more than 24 hours after the initial injury. Stroke secondary to dissection is believed to occur for up to 1 month from the time of dissection. The majority of arterial dissections heal within 3 to 6 months, and luminal stenosis completely resolves in 90% of cases over this time. In cases of complete occlusion, 50% of the vessels recanalize within the first 2 to 3 months, resulting in the restoration of blood flow.3
When followed with serial imaging, dissecting aneurysms are found to be completely resolved (5–40%), decreased in size (15–30%), unchanged (50–65%), or, rarely, enlarged.20 , 21 Dissecting aneurysms of the vertebral arteries are more likely to resolve than those of the carotid arteries. Persistent aneurysms are followed with imaging and are associated with a low incidence of delayed embolic events.22


♦ Imaging of Cervical and Vertebral Artery Dissections
Cerebral angiography remains the gold standard in the diagnosis of dissection; however, magnetic resonance imaging (MRI) and magnetic resonance angiography (MRA) offer sensitive noninvasive means by which to reliably detect cervical and intracranial dissections. T1-weighted fat saturation sequences may demonstrate a widened vessel diameter and a narrowed lumen surrounded by a hyperintense signal, representing the false lumen and intramural hematoma. The MRA may reveal a tapered or narrow vessel lumen at the site of dissection. MRI and MRA also represent the imaging modality most commonly employed in the long-term follow-up of dissections.16 , 23
Although MRI and MRA are the preferred noninvasive modalities for dissection, their lack of widespread availability in the community and the lengthy amount of time required to capture an image limit their use when dissection presents as an emergency. Computed tomography (CT) and CT angiography (CTA) are readily available in most centers and are of significant benefit when dissection presents with subarachnoid hemorrhage or ischemia due to hypoperfusion. CT is able to confirm subarachnoid hemorrhage in most instances, and in many cases is sensitive enough to detect early signs of ischemia. CTA reliably identifies changes in vessel caliber, false lumina, vessel occlusion, and pseudoaneurysms caused by dissection.14 , 16
Prior to the advent of modern imaging techniques, cerebral angiography provided the only imaging modality by which to confirm dissection. Currently, its role as a diagnostic tool has largely been supplanted by the noninvasive tools discussed above. However, angiography continues to play a critical role in the treatment of dissections, and the appearance of a dissected vessel on angiographic films bears mentioning. Dissections, both cervical and intracranial, may display one or more of the following characteristics: segmental arterial narrowing (string sign), segmental dilation (pearl sign), frank aneurysmal dilation, a double lumen representative of an intimal flap, a free-floating thrombus, and a tapered occlusion.23
♦ The Cervical Artery Dissection in Ischemic Stroke Patients (CADISP) Study Group: Medical Management of Dissections
In 2007, the Cervical Artery Dissection in Ischemic Stroke Patients (CADISP) Study Group published the results of a systematic meta-analysis of the existing clinical data on antithrombotic therapy for the treatment of cervical arterial dissections.18 The study represents the most comprehensive review of the pathophysiologic and clinical considerations regarding the use of antiplatelet agents versus anticoagulation. The current medical management of dissections is largely derived from the practice guidelines put forth in this review, and an understanding of the presented data is useful in guiding clinical decision making.
The predominant risk associated with cervical arterial dissection is that of stroke, occurring as the result of either thromboembolism or hemodynamic insufficiency. The vast majority of studies support thromboembolism as the primary etiology. Transcranial Doppler of the vasculature distal to a cervical dissection of the carotid or vertebral arteries shows a high incidence of microemboli in the intracranial distribution of the involved vessels.18 , 24 A review of CT and diffusionweighted MRI imaging has revealed predominant cortical, large subcortical, or multiterritorial infarcts consistent with thromboembolic events. Multiple studies have shown an incidence of only 3 to 16% of patients with a watershed distribution infarct suggestive of hypoperfusion. Angiographic data also support thromboembolism as the predominant cause of stroke in dissection, revealing branch occlusion of intracranial vessels in the distribution of the dissected vessel.18
Although the evidence supports thromboembolism as the primary cause of dissection-induced stroke, making anticoagulation the intuitive option, there is currently a lack of evidence from randomized prospective studies to support its routine use.18 Further complicating the decision-making process is a growing body of evidence that highlights the bleeding risks associated with anticoagulation. Whether the mechanism of dissection is an intimal tear or a direct bleed from the vasa vasorum, intramural blood accumulation is the common end point. When this phenomenon is taken into consideration, it is reasonable to assume that anticoagulation may lead to persistent or recurrent rehemorrhage, thereby propagating the dissection. Additionally, expansion of the mural thrombus can further narrow the true lumen, thus increasing the risk of hemodynamic compromise, delayed vessel occlusion, and possible stroke secondary to hypoperfusion. Indeed, multiple studies have shown delayed vessel occlusion in patients treated with anticoagulation, although mural thrombus rehemorrhage has not been proven.18
The existing data from clinical series were also found to be inconclusive, with conflicting findings of varying significance. Subarachnoid hemorrhage is believed to be a rare, yet devastating complication most commonly associated with intracranial extension of a dissection. Studies conducted with antiplatelet agents or anticoagulation failed to show a significant difference in the rate of subarachnoid hemorrhage associated with dissection, but the absolute risk of antithrombotic-associated subarachnoid hemorrhage has never been defined. Similarly, the rate of hemorrhagic conversion of an ischemic infarct is currently unknown and may represent a significant deterrent to anticoagulation. Stroke recurrence has been reported in patients treated with antiplatelets and in those treated with anticoagulation, but the literature fails to show the benefit of one intervention or define the true incidence of stroke recurrence.18 Specific clinical scenarios, such as recurrent thromboembolic events on antiplatelets, the presence of a free-floating thrombus, and occlusion of the dissected artery, may require further consideration of anticoagulation.
The findings of the CADISP study group meta-analysis highlight the need for a large randomized controlled trial comparing anticoagulation with antiplatelet agents.18 However, in the interim, the existing data establish a set of treatment recommendations that when paired with careful risk-benefit analysis, can be used to tailor treatment on a case-by-case basis. In all situations, the magnitude of the therapeutic benefit of long-term antithrombotic therapy must be weighed against the increased bleeding risk of anticoagulation.
♦ Surgical Intervention and the Management of Dissections
Although the vast majority of dissections may be treated with medical management, multiple scenarios, both ischemic and hemorrhagic, require the consideration of operative intervention. Hemodynamic insufficiency secondary to decreased luminal diameter, pseudoaneurysms, and subarachnoid hemorrhage represent commonly encountered pathology amenable to open or endovascular surgery. Traditional surgical intervention consists of craniotomy and proximal ligation of the dissected vessel, clipping or wrapping of associated pseudoaneurysms, and extracranial to intracranial bypass. Although effective in restoring blood flow and maintaining cerebral perfusion, the extended operative time required for the large exposures and delicate microsurgical technique limits the utility of these procedures in the setting of acute ischemia. Additionally, multiple studies demonstrate relatively high morbidity and mortality rates associated with such procedures, including an incidence of cranial nerve injury as high as 58% and a perioperative stroke rate of 10%.25 – 27
The limitations of these open approaches combined with advances in endovascular therapy have generated interest in stenting and stent-assisted coiling as a primary treatment modality. The relatively rapid nature of endovascular intervention dramatically reduces operative time and eliminates the need to prepare recipient and donor vessels for bypass. In contrast to open bypass procedures, which require temporary interruption of blood flow, stenting is able to reconstruct dissected vessel walls while maintaining continuous perfusion throughout the entirety of a case. In the setting of multisystem trauma, the ability to access the dissected vessel via a site remote from other injuries, such as the femoral artery, allows for effective treatment with minimal patient manipulation or positioning. Angiographic findings accurately define the collateral blood supply and determine the presence of additional vascular pathology within the same operative setting. The minimally invasive approach of endovascular intervention dramatically reduces the risk of further ischemic insult and cranial nerve injury frequently encountered in the meticulous dissections associated with open treatment.
Ischemic Presentation of Dissection and Surgical Intervention
When dissection results in acute severe stenosis or luminal occlusion, hemodynamic insufficiency and decreased perfusion, not thromboembolism, are the causative agents of cerebral infarction. Effective therapy consists of prompt recognition of hypoperfusion as the etiology of neurologic compromise and the initiation of medical therapy to stabilize the patient and optimize cerebral perfusion (see Figure 36.3 ). In the event of declining mental status or labored respiratory effort, immediate control of the airway is the first priority. Once respiratory stability has been established, and ideally in parallel, measures must be taken to maximize cerebral perfusion. High-volume fluid resuscitation and blood transfusion in the presence of anemia are utilized to at least maintain a normotensive state, with moderate hypertension most likely proving beneficial. An arterial line is useful in providing continuous monitoring of the mean arterial pressure. Likewise, routine insertion of a Foley catheter allows for strict monitoring of urinary output, with the goal of achieving aeuvolemic to slightly hypervolemic goal. When stable, surgical intervention, either open or endovascular, should be considered to restore normal luminal diameter and cerebral blood flow.

At our institution, acute hemodynamically significant cervical and intracranial dissections are routinely evaluated for possible angioplasty and stenting. Current stent technology enables reconstruction of the vessel wall, while maintaining cerebral perfusion throughout the entirety of the case. Stenting supports the true vessel lumen, reapproximates the edges of the intimal tear, and traps the intramural hematoma within the vessel wall, thereby restoring the original anatomy and decreasing the risk of thromboembolic events. As the dissection heals, the stent is incorporated into the vessel wall via endothelialization and the underlying hematoma is resorbed (Fig. 36.4).
Stenting has also found utility in the management of subacute and chronic symptomatic dissections refractory to medical therapy and in patients with contraindications to anticoagulation. Additionally, patients with asymptomatic dissections but persistent severe stenosis may also be considered for surgical intervention, as the incidence of thromboembolism is more than doubled (0.7% versus 0.3%) in this population. In these situations, stenting is usually the preferred modality, yet no guidelines exist to indicate the appropriate time interval over which to initiate treatment. Multiple studies cite the North American Symptomatic Carotid Endarterectomy Trial (NASCET) criteria, referring to the benefit of surgical intervention over medical therapy in symptomatic patients with 50 to 70% stenosis secondary to atherosclerotic disease.27 Clearly, prospective randomized studies are needed to better define the role of surgical management in this population.
Delivery of a stent to the extracranial or intracranial vasculature introduces a foreign body into the vessel lumen and further disrupts the antithrombogenic endothelial surface. Thus, in the acute setting, placement of an intravascular stent creates a prothrombotic environment, induces local platelet aggregation, and promotes thromboembolism. As a result, antiplatelet therapy is routinely initiated at the time of stenting and continued until re-endothelialization of the vessel wall is complete. Protocols differ between institutions, but most consist of a 600-mg load of clopidogrel with or without aspirin followed by daily dual therapy. Due to the need for antiplatelet therapy, intracranial hemorrhage, primarily subarachnoid hemorrhage due to intracranial extension of a cervical dissection, should be ruled out via head CT prior to stenting. The presence of subarachnoid hemorrhage may alter treatment protocols or entirely exclude stenting as a treatment option. In these situations, temporary balloon occlusion and sacrifice of the vessel with or without bypass may be the preferred intervention.

Close follow-up with angiographic or noninvasive imaging modalities is critical in patients who have been stented for cervical or intracranial dissections. Myointimal hyperplasia represents the chronic response of the vessel to the presence of a foreign body and results in the gradual thickening of the vessel wall. Over time, significant in-stent stenosis or occlusion may occur, resulting in delayed infarction remote from the original event. When discovered prior to additional neurologic insult, significant narrowing or occlusion may require further treatment with angioplasty, stenting, or open bypass.
Clinical Cases
Case 1 demonstrates endovascular stenting of a carotid dissection presenting with hemodynamic insufficiency (Figs. 36.5 and 36.6). Case 2 demonstrates dissection and complete occlusion of the cavernous carotid presenting with an acute stroke (Figs. 36.7 and 36.8).
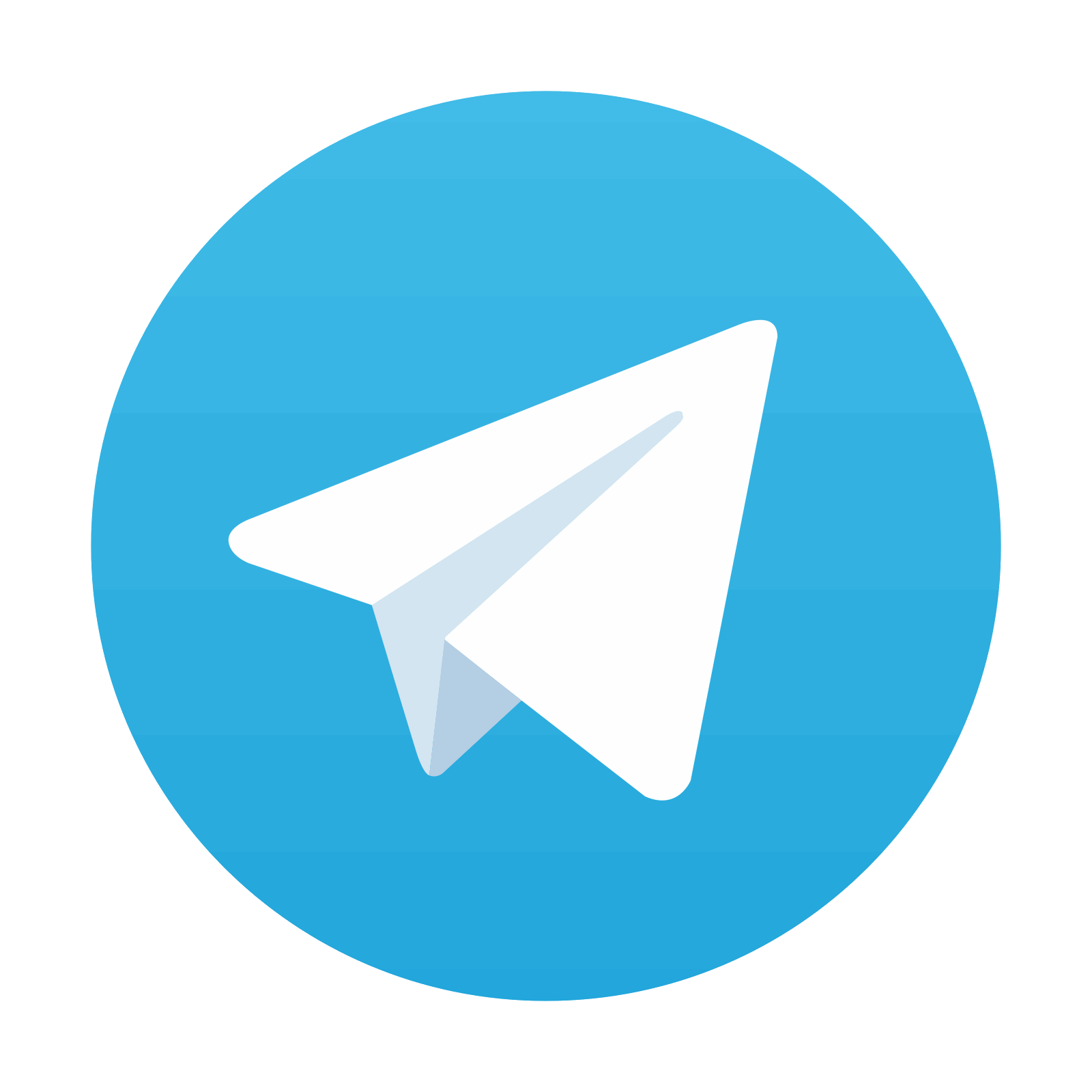
Stay updated, free articles. Join our Telegram channel

Full access? Get Clinical Tree
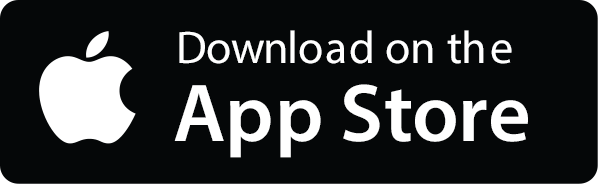
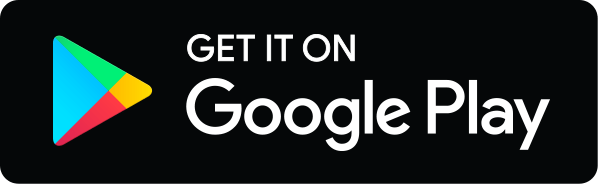
