Endovascular Management of Intracranial Dural Arteriovenous Fistulas
Pearls
Dural arteriovenous fistulas (DAVFs) are both a result and a cause of dural sinus thrombosis.
The pattern of venous drainage determines lesion grade, and cortical venous drainage heralds an extremely aggressive course.
Transvenous occlusion of the common draining vein requires careful mapping of cerebral venous drainage to avoid inadvertent venous infarcts and hemorrhage.
Transarterial occlusion is now more feasible using the liquid embolic agent Onyx but requires a detailed understanding of dural arterial anatomy to avoid eloquent collaterals and cranial nerve injury.
Dural arteriovenous fistulas (DAVFs) account for 15% of all high-flow intracranial vascular lesions.1 Evaluation of the prognosis of a lesion and its potential therapies requires a detailed angiographic workup combined with a sound knowledge of dural arterial and venous anatomy. After reviewing this vital basis, we shall discuss the main transvenous and transarterial endovascular approaches to endovascular therapy as well as modified/combined techniques.
♦ Applied Dural Arterial Anatomy
Dural fistulas tend to recruit regional supply from adjacent arterial pedicles in a predictable fashion. Large shunts can eventually draw in supply from contralateral arterial pedicles (Fig. 38.1). Understanding the dural arterial anatomy and potential direct cranial nerve supply or anastomotic supply to the brain and orbit is of increased importance due to the increased application of transarterial liquid embolization since the availability of ethylene vinyl alcohol (Onyx, ev3, Irvine, CA) in 2005.2
The dura mater is supplied almost exclusively by extradural branches of the external carotid (ECA), internal carotid (ICA), and vertebral arteries (VA). A notable exception is the artery of Davidoff and Schechter, a dural branch arising from the posterior cerebral artery (PCA) that supplies the tentorial dura (Fig. 38.2).3 Normal intraaxial cerebral and posterior fossa brainstem/cerebellar arteries are delimited peripherally by the pia-arachnoid (leptomeninges) and subject to the blood-brain barrier. However, if there is a large and chronic shunt, these arteries may be recruited by a “sump effect” to supply the dural lesion (Fig. 38.3).
A brief account of pertinent dural arterial anatomy follows. The locations of potentially dangerous anastomoses among the ECA, ICA, and VA are summarized in Figs. 38.4 and 38.5 .
The middle meningeal artery (MMA) arises from the proximal internal maxillary artery (IMaxA) and takes a characteristic hairpin bend as it traverses the foramen spinosum. After becoming intracranial it gives off immediate branches to the dura of the cavernous sinus and petrous ridge (the latter constituting an important supply to the geniculate portion of the facial nerve, along with the stylomastoid branch of the posterior auricular artery). It then gives off the main squamosal and frontoparietal branches, which course posteriorly across the calvaria in a smooth anteriorly convex arc. The latter are distinguished from the overlapping superficial temporal artery (STA) on lateral angiograms by the marked tortuosity of the latter’s subcutaneous branches.
The accessory middle meningeal artery (aMMA) may arise from a common trunk with the MMA or arise independently and more distally along the IMaxA. After traversing the skull either with the mandibular nerve through the foramen ovale or the adjacent foramen of Vesalius, it gives off branches to the cavernous dura and can anastomose with the corresponding branches of the inferolateral trunk (ILT) of the ipsilateral cavernous ICA.

The most distal IMaxA supply arises from the artery of the foramen rotundum, which has a characteristic sawtooth pattern on a lateral angiogram as it travels superiorly and posteriorly along with the maxillary nerve to supply the cavernous dura (Fig. 38.6). The terminal IMaxA can anastomose with the ophthalmic artery via sphenopalatine to ethmoidal networks and with the accessory meningeal via the pterygovaginal artery, which supplies the roof of the pharynx and eustachian orifice. The vidian artery provides an extremely diminutive connection between the IMaxA and the lateral carotid segment, but can be enlarged in chronic collateralization states (Fig. 38.6).




The STA gives off a small transosseous supply to the dura, particularly over the vertex close to the superior sagittal sinus. A palpebral branch may anastomose with the ophthalmic artery at the lateral orbital margin.
The occipital artery (OccA) gives off transmastoid and transoccipital transosseous branches. As with the superficial temporal artery, these can be difficult to traverse, and necrosis of the scalp is a risk if the OccA or STA is embolized with liquid embolic agents.

The ascending pharyngeal artery (APA) gives off neuromeningeal branches that traverse the hypoglossal and jugular foramina with a variable meningeal supply that is balanced with the posterior meningeal branch of the vertebral artery. It gives an important supply to the cranial nerves IX to XII. A branch to the periodontoid arcade can anastomose with the C3 vertebral segmental artery. The superior pharyngeal branch gives off a carotid branch that traverses the foramen lacerum to anastomose with the ICA.
The meningohypophyseal trunk (MHT) of the posterior cavernous ICA gives off the marginal tentorial branch of Bernasconi-Cassinari (Fig. 38.5) and also supplies the cavernous dura along with branches of the ILT, which arises from the anterior cavernous ICA. The latter may anastomose with the artery of foramen rotundum and the ophthalmic artery via a recurrent branch. Medial and lateral clival branches course inferiorly from the MHT and ILT, respectively, where they anastomose with corresponding hypoglossal and neuromeningeal branches of the ascending pharyngeal artery. There are no normal dural branches arising from the intradural ICA. In extreme cases of high-flow shunting, the dural territory may recruit supply from the adjacent pial branches of the cerebral arterial territories (Fig. 38.7).
The ethmoidal branches of the ophthalmic artery (OA) traverse the floor of the anterior cranial fossa at the foramen cecum and give off an anterior falcine branch. They are almost always the main supply to fistulas in this region, often with the bilateral OAs involved.4 The MMA may also contribute to their supply but is usually secondary.
♦ Applied Dural and Cortical Venous Anatomy
Dural arteriovenous fistulas are defined and graded by their means of dural or cortical venous drainage (see below). Dural sinuses are venous channels within the dura itself and tend to have a triangular cross-sectional profile with flattened sides. Cortical veins and the perimedullary veins around the brainstem traverse the subarachnoid space and are rounded in cross sections and more tortuous as they pass over adjacent anatomy such as cortical gyri. Figures 38.8 and 38.9 demonstrate the lateral projection venous phases, from ICA and VA injections, respectively, superimposed on the same patient’s sagittal magnetic resonance (MR) anatomy.
Dural Venous Anatomy
The superior sagittal sinus (SSS) has a variable size anteriorly and gradually enlarges as it passes posteriorly to accommodate the draining paramedian medial and lateral convexity hemispheric (frontal, parietal, and occipital) cortical veins. Due to the small size of the SSS anteriorly, DAVFs of the floor of the anterior cranial fossa tend to drain into a cortical vein before reaching the SSS (Fig. 38.10).5 The anatomic arrangement of the torcular herophili varies widely but it usually receives the SSS and the straight and occipital sinuses, and divides into the left and dominant right transverse sinuses (TSs). In addition to the deep venous drainage though the vein of Galen, the straight sinus receives the inferior sagittal sinus, which is normally a tiny structure in the inferior margin of the falx cerebri. A vestigial embryonic posterior falcine sinus can be recanalized in pathologic states and extends from the vein of Galen to the SSS several centimeters above the torcular (Fig. 38.11).5 The transverse sinuses receive the adjacent occipital and temporal cortical veins, either directly or via inconstant tentorial sinuses. A midline occipital sinus usually drains up into the torcular but may drain inferiorly around the foramen magnum via a marginal sinus into the jugular or condylar-suboccipital vein. The sigmoid sinus is applied to the inner surface of the petrous bone as it descends from the TS to the jugular fossa, and turbulent flow may be audible to the patient in this location even in the absence of an arteriovenous malformation (essential vascular tinnitus).6 It is also prone to involvement by inflammatory diseases of the middle ear and mastoid sinus.
The paired cavernous sinuses are located directly behind the superior orbital fissures on each side. They receive drainage from the superficial middle cerebral vein, sphenoparietal sinus, and superior and inferior ophthalmic veins, the latter often joining before draining into the sinus. Drainage is usually predominantly via the superior and inferior petrosal sinuses into the transverse sinus and jugular bulb, respectively, and with variable drainage via the basilar plexus and transforaminal emissary veins mainly through the foramen ovale to the pterygoid plexus. As with other large dural sinuses, the cavernous sinus may be compartmentalized by fibrous trabeculae (Willis cords) complicating transvenous treatment. Further confusion is added by the inconstant presence of a laterocavernous sinus, a separate entity in the lateral wall of the cavernous sinus that commonly drains the superficial middle cerebral vein (SMCV) to the pterygoid veins or petrosal sinus, but which only communicates with the cavernous sinus in about a third of cases.7 Intercavernous sinuses communicate between the two cavernous sinuses under the diaphragma sella, together creating a “circular sinus” around the pituitary infundibulum. There may also be intercavernous communication through part of the clival plexus over the back of the dorsum sellae.
Superficial Intradural Veins
The sylvian vein (superficial middle cerebral vein) receives the superficial perisylvian cortical venous drainage and passes anteriorly and medially over the lesser sphenoidal wing to drain into either the cavernous sinus or the laterocavernous sinus, directly via emissary veins through the foramen ovale into the pterygoid plexus, or extending back over the floor of the middle cranial fossa to the transverse sinus. The term sphenoparietal sinus is often used as a synonym for the sylvian vein, but anatomic studies have shown that the former is a separate structure, paralleling the sylvian vein at the lesser sphenoidal wing, and it receives dural venous drainage alone.8 Perisylvian drainage may also pass to the posterior transverse sinus and the superior sagittal sinus via the anastomotic veins of Labbé and Trolard, respectively. Any of these three perisylvian routes can dominate over the others. Although the remaining superficial cortical veins drain relatively directly into the nearest sinus, they do so at an angle (Fig. 38.8) and can run a variable distance adjacent and parallel to the sinus before joining it. The junction between the cortical vein and sinus is relatively inelastic and can appear focally (pseudo)-stenosed if receiving a pathologically dilated vein.





Posterior and lateral cerebellar hemispheric cortical veins drain either directly into the torcular and transverse sinuses, or enter them via sinuses in the tentorium. The vermian veins and more anterior cerebellar hemispheric veins drain into the galenic or petrosal routes.
Deep Intradural Veins
The deep system drains the periventricular medullary/transependymal, septal, caudate, thalamostriate and thalamic veins via the paired internal cerebral veins, vein of Galen, and straight sinus. The bilateral basal veins of Rosenthal traverse the ambient cisterns above the posterior cerebral arteries and receive a variable contribution from the deep middle cerebral, orbitofrontal, inferior thalamic, and lateral mesencephalic veins.
The perimedullary veins of the brainstem run longitudinally (anterior pontomesencephalic, anterior medullary, lateral mesencephalic, and lateral pontomesencephalic) and transversely (posterior mesencephalic, pontomesencephalic sulcal, transverse pontine, and pontomedullary sulcal veins). They are closely applied to the surface of the brainstem (Fig. 38.9). Drainage can predominate superiorly via the basal vein of Rosenthal, superiorly via the precentral vein to the vein of Galen, laterally via the petrosal vein to the superior petrosal sinus, or inferiorly to the spinal venous system.9 , 10
♦ Pathogenesis
Although simple, direct arteriovenous fistulas are often seen incidentally on angiograms performed soon after severe head trauma or surgical craniotomy, these are usually small, self-limiting, and not clinically significant. In cases of trauma and intracranial hemorrhage where there is uncertainty whether the trauma may have occurred secondary to collapse after an initial hemorrhagic event, traumatic fistulas may be recognized by their proximity to skull fractures or extradural hematomas, the clean appearance of a single irregular artery and parallel draining dural vein, the lack of recruitment, and the dilatation of feeding vessels (Fig. 38.12). In contrast, the “idiopathic acquired” DAVFs that constitute the subject matter of this chapter usually have established multiple feeders with a “leash” or network centered on the fistula itself (Fig. 38.13).
As many DAVFs recruit a prominent leash of dural vessels, it is not surprising that until about 30 years ago these were mistakenly considered to be congenital dural-based arteriovenous malformations (AVMs), analogous to pial AVMs. The realization of their acquired nature gained momentum after several case series demonstrated the de-novo angiographic evolution of complex fistulas after previous sinus thrombosis11 or trauma.12 Terada et al13 were later able to generate a DAVF by inducing venous hypertension in a rat model. The finer details of normal dural vascularization, which are grossly underappreciated by clinical angiography, were elucidated by histologic analysis, which can distinguish normal arteries and veins in the wall of the dura.14 These same networks (vasa venorum) are responsible for the “empty delta sign” of enhancement evident in the wall of a thrombosed sinus on contrast-enhanced computed tomography (CT) or magnetic resonance imaging (MRI) (Fig. 38.14).15 Small dural arteries (<200 μm) and veins are intimately related in the dural subintima, concentrated at points of dural fusion along the margins of the sinuses as well as at junctions between the sinus and cortical veins14—the latter arrangement explaining the prevalence of high-grade lesions draining primarily into cortical veins (see below). Direct pathologic communication between these vessels has been documented histopathologically in cases of DAVF. These arterialized veins then traverse the intima to empty into the sinus. Sinus thrombosis, usually occult, is considered to be the initial insult leading to the genesis of a DAVF in the majority of cases. During the period of sinus occlusion, fistulas are created due to elevated back pressure leading to dilatation of preexisting microscopic anastomoses (which explains the frequent finding of “satellite” DAVFs), local ischemia leading to neovascularization by pathologic vessels, or inflammatory injury to the intervening capillary network during the period of thrombosis with subsequent fibrinolysis. To add confusion, sinus thrombosis may itself be a result of a preexisting DAVF due to turbulent flow and an occlusive venopathy that can occur due to intimal hyperplasia and intimal swelling consequent to the dilated vasa venorum. The potential for subsequent occlusion or recanalization of venous drainage explains the possible spontaneous evolution of a fistula that can upgrade due to thrombosis of a previously benign drainage route. However, as described in the following section, low-grade fistulas tend to have a benign natural history. Venous thrombosis can also lead to spontaneous improvement and even complete obliteration of a fistula.16 , 17

♦ Grading
The two established grading systems were published in quick succession by Borden et al18 and then Cognard et al19 in 1995. The Cognard classification is illustrated in Fig. 38.15 . Both systems relate the pattern of venous drainage of a DAVF to the risk of having an aggressive outcome, such as intracranial hemorrhage or nonhemorrhagic neurologic deficit (NHND) due to venous hypertension.
Cognard’s data were based on a retrospective single center experience of 205 patients over an 18-year period. As an example of the difficulty in subanalyzing such rare disorders, there were only 10 patients in the CG2b group. Also, of the 205 patients, only 120 received follow-up with a range of 6 months to 23 years (mean 52 months), and, apart from the CG1 lesions, Cognard’s data do not distinguish between symptoms occurring at presentation versus during follow-up. However their data remain the benchmark for estimating the risk of a particular lesion having an adverse outcome. In brief, in patients without any cortical drainage (CG1 and 2a) there were no cases of intracranial hemorrhage, whereas hemorrhage occurred in 10% of CG2b, 6% of CG2a+b, 40% of CG3, and 65% of CG4. Nonhemorrhagic aggressive symptoms were found in less than 2% of CG1, 37% of CG2a, 10% of CG2b, 61% of CG2a+b, 36% of CG3, and 31% of CG4. Intracranial hemorrhage is usually parenchymal, in the territory of an involved cortical vein, with possible subarachnoid breakthrough or subdural hematoma. Cognard found that fisulas in the anterior fossa, tentorium, and torcular had higher rates of aggressive symptoms (88%, 92%, and 100%, respectively), but this correlated with the likelihood of cortical venous drainage and, allowing for this, location itself was not an independent arbiter of risk. Of 12 patients with CG5 lesions draining from an intracranial fistula into perimedullary spinal veins, six suffered a progressive myelopathy, five had subarachnoid hemorrhage, and one had a focal neurologic deficit.
Borden’s classification is as follows: BG1, drainage into a sinus only (antegrade or retrograde); BG2, reflux from a sinus into a cortical vein; and BG3, drainage into a cortical/subarachnoid vein only. Borden et al illustrated their system with cases but did not publish data to quantify the outcomes associated with their different grades, as did Cognard et al. Instead, they had two subclassifications: type a, single fistulas; and type b, multiple fistulas. They considered the spinal epidural veins to be equivalent to intracranial sinuses and classified the majority of spinal DAVFs as type 3 (dural lesion draining directly into the subarachnoid coronal venous plexus).



As mentioned, apart from CG1 lesions, neither analysis quantifies the risk of a future adverse event from the time a patient has been diagnosed with a DAVF. Most high-grade lesions are treated and cured urgently. The Toronto group followed over a mean of 4.3 years 20 patients with DAVF and cortical venous reflux (CVR) who had either failed or refused treatment. This cohort had an annual event rate of 15% (8.1% intracranial hemorrhage and 6.9% NHND) and a 10.4% annual mortality rate.20 However, it appears that not all patients with cortical venous reflux are at such a high risk; Strom et al21 analyzed a cohort of DAVFs with CVD in 17 patients presenting with aggressive symptoms versus 11 patients without aggressive symptoms, and found respective annual rates of hemorrhage or NHND of 19% versus 1.4%.
Barrow et al22 devised a classification of cavernous sinus fistulas in 1985 that includes the type A, direct ICA to cavernous fistula, usually due to a traumatic tear of the ICA or a ruptured aneurysm; and types B to D, which are dural fistulas subclassified by their arterial supply.22 Type B receives dural supply from the cavernous branches (ILT and MHT) of the ICA alone, type C is supplied only by dural branches of the ECA, and type D is supplied by both the ICA and ECA branches.
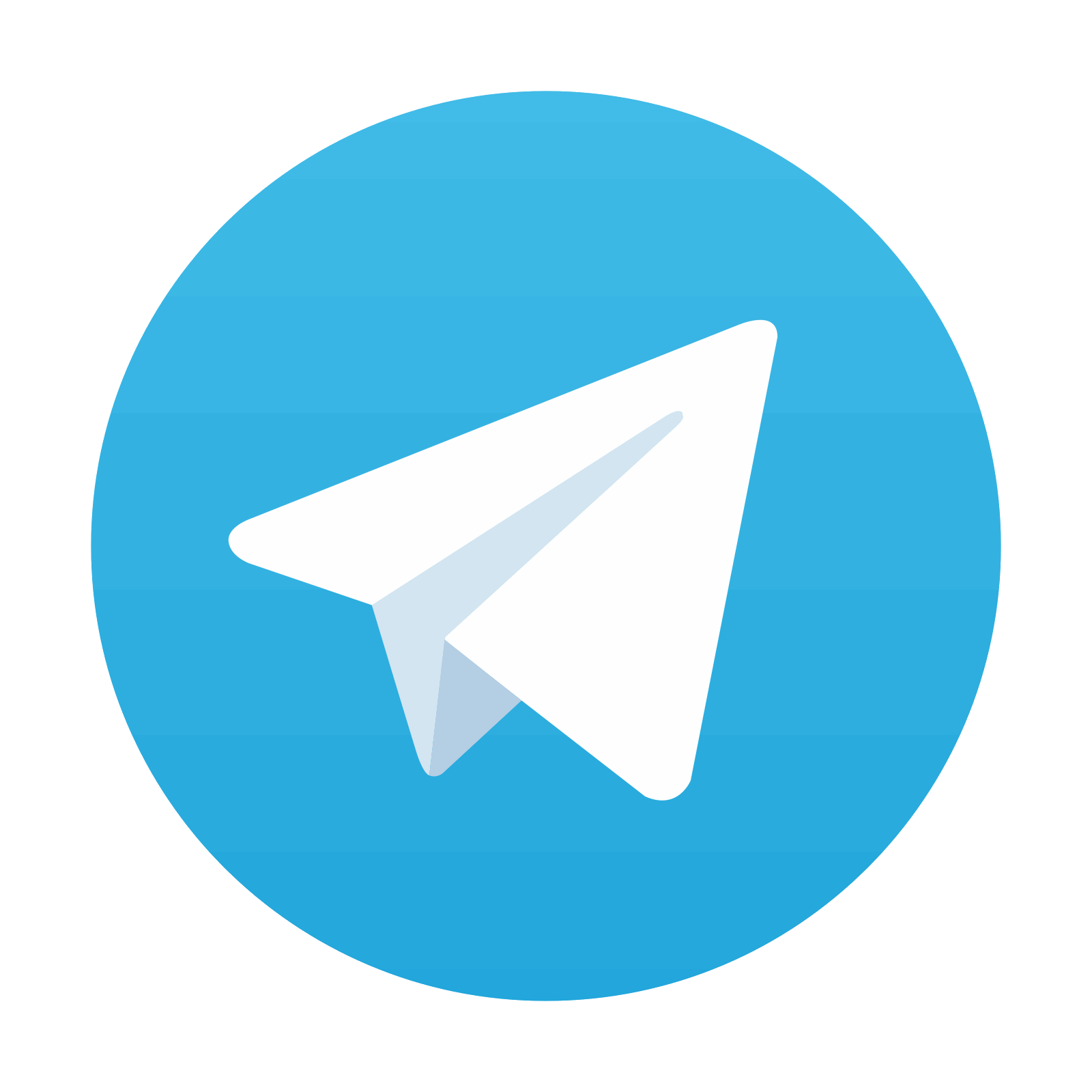
Stay updated, free articles. Join our Telegram channel

Full access? Get Clinical Tree
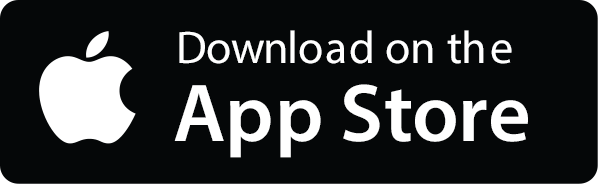
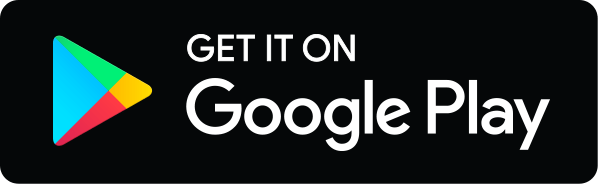