183CHAPTER 8
Artifacts in Pediatric and Adult Magnetoencephalography
Christos Papadelis, Michel AlHilani, and Phillip L. Pearl
INTRODUCTION
Magnetoencephalography (MEG) records the magnetic brain activity that is measurable using extracranial sensors. The measured magnetic fields created by electric currents in the brain are extremely weak and prone to multiple artifacts. Artifacts can be generated by strong ambient magnetic fields (ambient artifacts) external to the patient’s body or by electromagnetic sources inside the patient’s body (biological artifacts). Both ambient and biological artifacts can obscure the MEG signal, rendering the electromagnetic activity generated by the human brain uninterpretable. Moreover, artifacts can be falsely attributed to cerebral sources, significantly altering the results and clinical interpretation of the MEG recording. There are artifacts that are unique to the MEG setup and there is limited literature that provides a detailed description of artifacts in MEG (1–3). In this chapter, we give examples of ambient and biological artifacts that are encountered in the clinical performance of MEG used for children and adults.
AMBIENT ARTIFACTS
Ambient sources include interference from power lines and electrical motors or from large, moving objects made of ferromagnetic materials. The interference from power lines contains the main frequency (50 Hz in Europe, Asia, and Australia; 60 Hz in the United States and most countries in North and South America) and its harmonics. Artifacts from electrical motors also have a constant frequency that corresponds to the rotating frequency of the motor. Both power mains and electrical motors generate artifacts that are almost always present in MEG recordings and can be easily recognizable. Common sources of moving objects in urban hospital settings include cars, commuter trains, subways, elevators, metallic furniture, and hospital beds. The electromagnetic signals generated by moving objects are characterized by temporal scales similar to the movement; the frequency is predominantly below 1 Hz (2). Although most of these artifactual sources are distant, they are still difficult to identify and eliminate because (a) they are not always present in the signal, (b) the location of the source is often not static, and (c) they often resemble pathological or normal variants of brain origin. For example, magnetic fields from a car travelling at a distance of 2 km are roughly equivalent to those produced by the human brain (4). Mechanical vibrations from the buildings transmitted to the magnetically shielded room (MSR) might also be another source of artifacts in the MEG recordings. Paramagnetic and ferromagnetic materials that might be contained in exogenous or endogenous sources can give rise to large-amplitude artifacts that obscure the brain’s MEG signal (1,5). Exogenous sources of artifacts include jewelry, watches, keys, glasses, dyes, spectacles, hearing aids, clothing (i.e., underwire bras and zippers), or even facial makeup (i.e., mascara and face glitter). Endogenous sources include dental work, fillings and braces, tattoos, and implanted medical devices that are either active (i.e., defibrillators, neurostimulators, cochlear implants, and drug pumps) or passive (i.e., hip/knee joint replacements, heart valves, aneurysm clips, coronary stents, and breast implants). These types of artifacts generally produce very large, but low-frequency signals in the MEG data. They increase when the patient is moving his or her torso, upper or lower extremities, or jaw and can be easily recognized by asking the patient to blink or move, thereby reproducing the artifact. Artifacts from dental work and facial makeup may be made worse if the patient was recently scanned in an MRI device because such materials may become magnetized.
184BIOLOGICAL ARTIFACTS
MEG recordings are more often confronted by interference from the patient rather than from the environment. Eyeblink, cardiac, respiratory, and muscle artifacts are common biological sources that disturb MEG recordings. Each of these artifacts has distinct spatial and temporal characteristics which the reader should become familiar with and be able to recognize. The human heart is one of the most insidious sources of magnetic noise in MEG. Cardiac artifact reflects either the magnetic counterpart of the QRS complex or pulsation of the ECG (6,7). Cardiac artifact in MEG is characterized by relatively brief pulses occurring at the same time as the patient’s heart rate. Normal cardiac function can also produce intermittent artifact by the ballistocardiographic effect. This can happen if the patient has any magnetic materials in his or her clothing that might fluctuate due to the mechanical rhythm of the cardiac muscular contraction causing an artifact in the MEG signal. Cardiac implantable devices (i.e., coronal stents) made of paramagnetic or ferromagnetic materials may also produce artifacts. Respiratory artifacts appear as slow, low-amplitude waves in MEG. Although they are usually not troublesome, they may be amplified by other factors. For example, magnetic lung contamination or magnetic material in clothing may cause slow shifts due to movement during respiration. Ocular artifacts (eye movements and blinks) are manifest as high-amplitude deflections less than a second in duration that appear predominately in the frontal channels. Other muscular artifacts can also contaminate the MEG signal. For example, tense neck muscles may emit continuous or bursting high-frequency noise, which becomes more prominent the closer the muscle is to the sensor array.
EXAMPLES OF ARTIFACTS
Commuter Train
Large moving objects that are located quite far from the MSR can cause enormous technical artifacts in the MEG dominating the brain activity signal and rendering the recording unreadable. Figure 8.1 presents an example of ambient artifact in the MEG from a commuter train. The artifact has an amplitude composed of a few thousands pico tesla (pT) compared to the brain activity magnitude of femto tesla (fT) (~100 fT for a cerebral evoked response and ~1,000 fT for cerebral alpha rhythm) and dominates the brain MEG activity. Such large amplitude corresponds to thousands of microvolts (mV) in EEG.
Cardiac Pulse Artifact
MEG is more sensitive to cardiac artifacts than standard scalp EEG. The most common biological artifact in MEG is caused by the heartbeat. It appears as relatively brief pulses occurring at the same frequency as the heart rate. It may transiently overlap with interictal epileptiform discharges, or rarely the ictal onset during MEG, distorting the source localization of the epileptiform activity. It appears more prominent on magnetometers than gradiometers (Figure 8.2). It has a specific spatial distribution in the topographic plot of the MEG signal that is perpendicular to the topography of EEG. Simultaneous ECG recordings are essential to recognize the cardiac pulse artifact in the MEG signal.
Ocular Artifacts
Moving eyeballs act as two large magnets and generate relatively prominent artifacts. Ocular artifact can either be due to eye blinks contaminating particularly the lower frequencies or due to saccadic eye movements that interfere with the MEG signal at higher frequency ranges. Certain saccadic movements may produce spiky artifacts that reflect high-frequency myogenic artifact (i.e., lateral rectus spikes). The myogenic spikes generated by eye movement are an important artifactual signal because they can mimic abnormality. It is observed at the onset of even tiny saccadic eye movements and is characterized by strong electrical transients that are believed to reflect contraction of the extraocular muscles in the orbit (8). Figure 8.3 shows an example of a typical eyeblink artifact as it looks in MEG traces. It appears as a short (between ~100 and ~400 ms), large deflection with highest amplitude in the sensors of the frontal head regions. Simultaneously, recording electrooculography (EOG) is useful to recognize vertical eyeblink artifacts in the MEG signal. Figure 8.4 shows an example of artifact due to saccades, which are visible mostly in horizontal EOG. The deflection has the maximum amplitude at the frontal and occipitoparietal areas, and its topography is 90 degrees out of phase compared with the vertical eyeblink artifact.
Myogenic Artifacts
Myogenic artifact is the main barrier against obtaining high-quality MEG recordings. This artifact can be identified as high-frequency activity that is usually present bilaterally and is seen most prominently in temporal and frontal head regions. Muscular activity produces artifact that appears within the bandwidth of frequencies between 20 and 300 Hz with most of its 185power at the lower end of the frequency range (9). Some facial muscles with intricate function are smaller in size and have higher nerve–muscle innervation density capable of generating myogenic activity that are high frequency, reaching up to 600 Hz (9). Most relevant to the MEG are the myogenic artifacts involved in chewing (50–60 Hz) that is generated by the masseter muscle in addition to the frontalis muscle, which controls wrinkling of the brow (30–40 Hz). Muscular activity may overlap entirely with the spectral bandwidth of high-frequency oscillations generated by neural activity (gamma, ripples, and fast ripples), making their distinction from artifact almost impossible (3,10). Figure 8.5 shows an example of myogenic activity in the MEG signal due to contraction of the right frontalis muscles. The topography across different times helps to indicate the exact location of the muscle that is contracting. Figure 8.6 illustrates an example of chewing artifact contained within the MEG signal. Note the topography of the signal indicates a bilateral frontotemporal source for the artifact. Figure 8.7 is an example of myogenic artifact due to slight head movement that results from elbow flexion from arm movement. Myogenic artifacts can cause significant distortion in the topographic maps masking the spatial origin of pathological activity and affecting the reliability of the magnetic source imaging (MSI). Figures 8.8 and 8.9 show the distortion of EEG and MEG topographic maps due to myogenic artifact, which are concordant to an interictal epileptiform discharge (green arrows) in a pediatric patient with focal epilepsy. Figure 8.10 demonstrates the dislocation of an equivalent current dipole (ECD) at the peak of a spike due to the presence of a myogenic artifact in the MEG signal.
Movement Artifact
Patient movement artifacts are more likely to impact MEG than EEG due to the MEG sensors that are in fixed locations relative to the head compared to EEG sensors that move with the head.
Large movements of the patient’s extremities may cause a mixture of low- and high-frequency artifact to occur in the MEG signal (see Figure 8.7). Sometimes, the rhythmicity, polarity, and location of an artifact can look suspiciously like an electrographic seizure. This is especially true for neonates who are prone to focal seizures that may be seen in only one to two electrodes. Figure 8.11 (11) shows a patting artifact as seen on a MEG tracing that resembles an electrographic seizure. Use of concomitant video to identify movement patterns that parallel artifactual patterns is critical in these situations to differentiate extracerebral activity. Similarly, close observation of the MEG is important to assess patterns of evolution and propagation present in electrographic seizures, which are less likely to be seen in a purely artifactual pattern.
Medical Devices
On many occasions, technical artifacts from exogenous and endogenous medical devices, such as activated implantable medical devices, can generate nonphysiologic artifact rendering the MEG signal uninterpretable. Figure 8.12 demonstrates an artifact generated in the MEG due to transcranial electric stimulation. Figure 8.13 illustrates artifact during an MEG recording in an adult patient with a cochlear implant. Note that most magnetometers are saturated by the cochlear implant disturbance, becoming unable to record any brain activity from the patient. Figure 8.14 represents artifact present in the MEG signals in an adult person with epilepsy implanted with a vagus nerve stimulator (VNS). Note the precisely periodic deflections that overlap the background activity in the MEG. Figure 8.15 reflects device-related artifact during MEG acquired from an adult during electrocochleography.
ARTIFACT REDUCTION IN MEG
Artifact reduction is an important issue, not only for securing interpretable MEG recordings, but also for performing accurate source localization (12). This is particularly important during the presurgical evaluation of patients with epilepsy where the presence of artifacts in the MEG signal can alter the reliability of the MSI, providing inaccurate information about the exact location of the seizure onset zone (see Figure 8.10) and the eloquent cortex (Figure 8.16) (13). Countermeasures that are used to minimize artifacts consist of (a) precautions that are taken during the setup and connection of the MEG system, (b) precautions and intervention during the recording, and (c) usage of artifact reduction software for post hoc signal analysis of the recorded MEG signals. Some MEG devices use gradiometers that are less sensitive to interference from ambient artifact sources far away. Other MEG devices also incorporate an array of reference sensors situated at a greater distance from the head, which are used to subtract external artifacts. To prevent the occurrence of artifacts from mechanical vibrations from the building, the MSR should be isolated as much as possible. However, despite these precautions, most recordings are still subject to numerous artifacts. To improve the efficiency of recording, all magnetic materials should be avoided in the clothing of the patient. In case of dental implants, fillings, bridges, or braces, demagnetization using special equipment may prove helpful to reduce the high-amplitude magnetic signals produced by metals. A simple but effective way to minimize ocular and myogenic artifacts is to ask the patient to fixate on a single point to restrict eye movement or relax the muscles involved in producing artifact (e.g., jaw). Obviously, this approach may be impractical 186for some younger pediatric patients and patients with neurodevelopmental disabilities. Therefore, in these situations simultaneous recording of video, ECG, EOG, and electromyographic (EMG) signals are essential to recognize artifacts.
At the preprocessing stage of data analysis, filtering the data in the frequency range of interest (usually between 1 and 70 Hz) is fundamental for minimizing artifacts. This is particularly true for artifacts that lie outside the brain’s traditional frequency range. The main power line and video artifacts are typically reduced using notch or comb filters (at 50 or 60 Hz). Figure 8.17 shows MEG signals before and after filtering with a 60-Hz notch filter. These digital notch filters cannot be overly narrow because the main power line frequency is not absolutely constant at a single frequency. Narrow filtering may also introduce “ringing” in the time domain and may filter out MEG components at the notch filter frequency. Furthermore, filtering is not a solution when the frequency range of artifacts overlaps with the spectrum of cerebral activity (i.e., myogenic artifact). Elimination techniques to exclude selected sensors that contain continuous artifact are helpful to avoid undesired effects on source analysis though they may also exclude underlying MEG signals. More advanced signal-processing techniques may also be helpful to remove artifact composed of common sources as well as techniques that compensate for motion. For example, regression-based or blind source separation-based techniques (such as independent component analysis) may be useful for removing specific patterns of artifacts (e.g., heartbeat and ocular artifacts) (14–16). Some of these methods require the simultaneous recording of ECG, EOG, and EMG signals that are essential not only for the recognition of artifacts but also for their elimination. More recently, the signal source separation (SSS) method has been proposed (17), which decomposes the MEG signals into two components which are generated from sources inside and outside of the sensor space, and removes the latter component. The temporally extended SSS also considers temporal signal correlation (18,19) and is widely used in clinical practice. Finally, to deal with motion artifacts that distort the geographic information of the head and sensors, special compensatory methods have been developed that continuously track the head position during acquisition of the MEG signal. This technique is particularly useful for analyzing ictal data where head deviation sometimes occurs during seizures, introducing artifact (20,21).
CONCLUSIONS
Both ambient and biological artifacts can obscure the MEG signal, rendering the electromagnetic activity generated by the human brain uninterpretable. Moreover, artifacts may be falsely attributed to cerebral sources significantly affecting the clinical interpretation of MEG recordings. Accurate discrimination of artifact from cerebral sources is a critical skill for the MEG interpreter. The use of time-locked video together with the simultaneous recording of ECG, EOG, and EMG signals is indispensable for determining the source of some artifacts and differentiating them from interictal epileptiform discharges and electrographic seizures. Special precautions before and during the recording may help minimize interference from a variety of artifacts. Finally, the use of more advanced signal-processing tools can successfully address a plethora of persistent artifacts that occur during MEG recordings obtained in adult and pediatric patients.
187
FIGURE 8.1: Continuous sinusoidal artifact from a commuter train recorded with Neuromag Triux (Elekta Instrument AB Stockholm, Sweden) system in the MEG unit at the Centre for Cognitive Neuroscience of the University of Salzburg, Austria (Courtesy of Gianpaolo Demarchi). Please note the enormous signal amplitude (units are in the magnitude of pT).
MEG, magnetoencephalography.
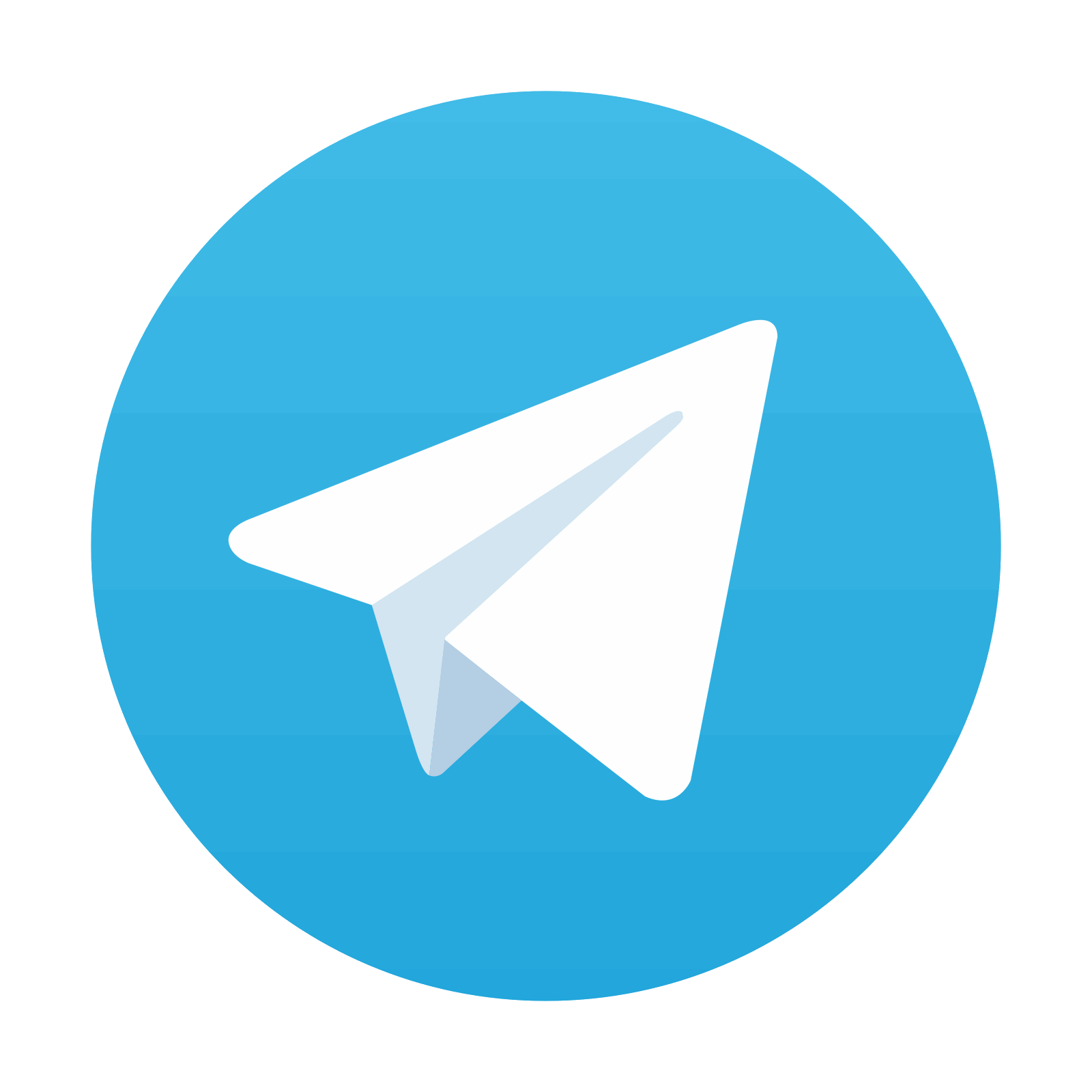
Stay updated, free articles. Join our Telegram channel

Full access? Get Clinical Tree
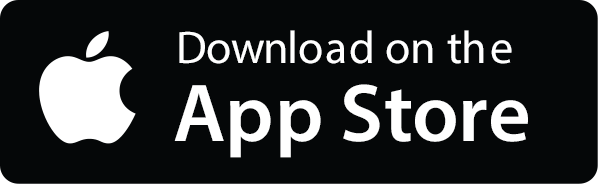
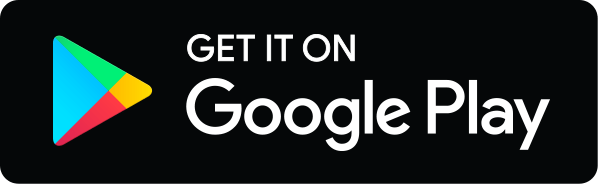