14
Autonomic Disorders in Children
In the human body, the autonomic nervous system (ANS) regulates fundamental, involuntary functions. The ANS is involved in temperature regulation, digestion, maintaining upright posture, control of breathing, and circulation. Practitioners of most medical specialties can benefit from understanding autonomic function and from the potential diagnostic insight provided by quantitative autonomic testing. Traditionally, care for children with ANS dysregulation (ANSD) has been distributed among a variety of subspecialists based on the primary affected organ system. As understanding of ANSD in children expands, so does the need for pediatric-focused autonomic specialists and centers.
Over the past several decades, objective and noninvasive techniques have been developed and standardized in adults to evaluate the functioning of cardiovagal, adrenergic, and sudomotor aspects of the ANS (1). With this review, we aim to provide an overview of existing experience with noninvasive testing of autonomic function in children and to outline areas of promising future development.
SPECIAL CONSIDERATIONS FOR AUTONOMIC TESTING IN CHILDREN
At different ages, children and adolescents are frequently unable or unwilling to provide a cogent description of their clinical symptoms. In the youngest children, the barrier is frequently limited by expressive language. In school-aged children, their primal need to not embarrass themselves and to avoid being “different” creates a barrier to their self-reporting. Adolescents tend to be extraordinarily self-absorbed, reporting all of the innate sensations occurring in their bodies making it difficult, given their different personalities, to know whether the reported symptoms are normal or alarming. It is in this context that laboratory testing of autonomic function in children and adolescents becomes important. Objective findings that can demonstrate normal or altered function in one of the autonomic pathways can be helpful diagnostically. This data can also serve to monitor changes in function over time. An understanding of the child’s age and developmental stage are key to anticipating a child’s ability to tolerate and cooperate with autonomic testing. For example, certain aspects of testing include lying completely still during the application of low currents to the skin (sudomotor testing) or maintaining sustained increase in intrathoracic pressure (Valsalva maneuver)—tasks that can sometimes be challenging for cooperative adults. Further, during analysis and interpretation of results, consideration should be given to the developmental and maturational changes in the nervous system from infancy through adolescence. The development of age- and gender-matched normative data has been and remains a central goal. The organ systems and types of autonomic testing pertinent to children and adolescents are listed in Table 14.1.
To be maximally useful in children, autonomic testing should be brief, have instructions that are easy to understand and carry out, and be nonintrusive/nonpainful. This is particularly important as there is much work to be accomplished establishing normal control values in healthy children under 10 years of age.
TABLE 14.1 ANS Regulation by System and Available Autonomic Testing
ORGAN SYSTEM | ANS COMPONENT | AUTONOMIC TEST |
Cardiovascular | Cardiovagal | – HRDB, HRST, HRSQ |
– VR | ||
– HRV | ||
Adrenergic | – Valsalva maneuver | |
– HUT | ||
– SSR | ||
– Biochemical (catecholamine levels) | ||
Vasomotor | – Digit wrinkle | |
Respiratory | Control of breathing including chemoreception | – Exogenous ventilatory challenges |
– Awake and asleep physiologic testing | ||
Skin | Sudomotor | – QSART |
– TST | ||
Ophthalmologic | Pupillary | – Pupillography |
Gastrointestinal | Enteric | – Gastric scintigraphy |
– Colonic transit | ||
Urologic | Sympathetic | – Urodynamics |
Parasympathetic | – Bladder US |
Abbreviations: ANS, autonomic nervous system; HRDB, heart rate response to deep breathing; HRSQ, heart rate response to squatting; HRST, heart rate response to standing (30:15 ratio); HRV, heart rate variability; HUT, head up tilt; QSART, quantitative sudomotor axon reflex test; SSR, sympathetic skin response; TST, thermoregulatory sweat test; US, ultrasound; VR, Valsalva ratio.
RATIONALE FOR AUTONOMIC TESTING IN PEDIATRICS
Quantitative ANS testing allows for the development of a deeper understanding of the interrelationship of multisystem features of ANSD and the associated clinical significance of dysfunction in these physiologically intertwined systems. Laboratory autonomic testing is most effective when it is paired with a clinical evaluation that includes ANS-focused questions. Based on the preliminary evaluation, the pediatric clinician may find that there are children in whom the diagnostic question is related to autonomic control over a specific organ system or that broad screening of autonomic function is appropriate in order to determine whether a symptom is due to isolated, widespread, and/or evolving autonomic dysfunction. Autonomic testing is indicated in congenital autonomic disorders as well as for the growing number of recognized diseases for which autonomic dysfunction is an acquired phenomenon (Table 14.2).
TABLE 14.2 Classification of Pediatric Autonomic Nervous System Dysregulation
CLASSIFICATION | EXAMPLES OF DISORDERS/DIAGNOSES |
Developmental/genetic | Alacrima, anhidrosis, adrenal insufficiency (AAAS) Congenital central hypoventilation syndrome (CCHS) Dopamine beta-hydroxylase (DBH) deficiency Fabry’s disease Hereditary sensory and autonomic neuropathies, including familial dysautonomia Hirschsprung’s disease Menkes syndrome Norepinephrine transporter (NET) deficiency syndrome Rett syndrome Shapiro’s syndrome |
Acquired | |
Autoimmune | Guillain-Barré syndrome Autoimmune neuropathy/ganglionopathy Postural orthostatic tachycardia syndrome |
Paroxysmal disorders | Autonomic storm (post central nervous system insult) Mast cell disorders Recurrent syncope Pheochromocytoma Postural orthostatic tachycardia syndrome Abdominal migraine, cyclic vomiting |
Disorders with associated autonomic dysregulation | |
Autism Chronic fatigue syndrome Cyclic vomiting syndrome Diabetes mellitus Migraine Mitochondrial cytopathies Rapid-onset obesity with hypothalamic dysfunction and autonomic dysregulation |
The development of noninvasive, child-friendly autonomic testing methods and obtaining normative data for children of both genders is ongoing. As this is accomplished, we need to acknowledge that the process of performing autonomic testing can change autonomic tone in children and adolescents and use care when selecting the test to be performed and the normal values to be used (2).
TEST DESCRIPTIONS
Cardiovagal
Cardiovagal function refers to the dynamic, coordinated impact of the parasympathetic component of the autonomic system in regulating heart rate (HR) and blood pressure (BP) in response to challenges. These physiologic challenges effect changes in cardiac output and venous return, while being affected by intravascular volume and posture. Standardized testing methods include measurement of HR and BP responses to deep breathing, Valsalva maneuver, and position change (sit to stand, stand to squat, and squat to stand).
Heart Rate Response to Deep Breathing
The HR response to deep breathing (HRDB) essentially provides a systematic and objective measure of the normal physiologic change in HR in response to breathing (“sinus arrhythmia”). With a combination of verbal coaching and visual pacing, subjects are asked to breathe slowly and deeply at 5 to 6 times per minute. HR during eight cycles (inspiration and expiration each taking 5 seconds) is recorded and the largest five consecutive responses are marked (Figure 14.1). HRDB is calculated as the mean of the consecutive differences between maximal and minimal HR. Normative values exist for ages greater than 9 years, with reported inverse relationship of HRDB with age (1,3). Specifically, normative values from 10 to 29 years of age in the Mayo Autonomic Lab are 14 to 41 (5th and 95th percentile, respectively) (1). HRDB has been used to identify children with presymptomatic trypanosomiasis infections in Peru (4).
FIGURE 14.1 shows an HRDB recording in a normal 7-year-old girl with respiration monitored on the top trace and heart rate on the lower trace.
Valsalva Ratio
A stereotyped Valsalva maneuver must be performed to calculate the Valsalva ratio (VR), which measures the vagal component of the baroreflex. For this test, the subject is resting and supine, with continuous HR and finger BP monitoring and is then asked to maintain a fixed expiratory pressure (40–50 mmHg) for 15 seconds by blowing into a mouthpiece with a partial air leak. The VR is the ratio between the maximum HR occurring during the maneuver and the lowest HR occurring within 30 seconds of the maximal HR. VR appears to be a less sensitive measure of cardiovagal dysfunction than HRDB in adults. Normal values are 1.5 to 2.9 (5th and 95th percentile, respectively) and do not vary with age or gender in adults (1). In children, normative data for healthy children appear to be similar to reported values in adults with Longin et al. finding the 5th and 95th percentile values to be 1.4 and 2.7, respectively, for 6- to 11-year-old children and 1.3 and 2.8, respectively, for 12- to 15-year-olds (5). Other pediatric investigators report slightly lower VR values with a mean of 1.65 in 6- to 15-year-olds (4) and a mean value of 1.5 in 10- to 17-year-olds (3). In pediatric practice, it is important to consider that small finger size may limit ability to obtain BP values and that children may have difficulty with maintaining the sustained intrathoracic pressure required to successfully complete this test.
HR Response to Standing
For this test, the subject has his/her HR continuously monitored in a supine position for a baseline interval, then gets to an upright position without assistance, followed by continued cardiac monitoring for 2 minutes. The HR response to standing (HRST or 30:15 ratio) is the ratio between the R-R interval at the 30th beat while standing and the 15th beat while standing. This has not been used as widely as the HRDB. Though children tend to stand up quickly and more uniformly (as compared to adults who have notable variability in time to stand), they also tend to be more fidgety, causing variability in response due to altered venous return from muscle contraction and to excessive movement causing recording artifact. Normative data (with some variation in protocols) has been published for children as young as 4 years of age (3–5).
HR Response to Squatting
The baroreflex involves a negative feedback response to changes in BP and can be tested noninvasively with continuous HR and finger BP monitoring while asking the patient to change position. With the heart rate response to squatting (HRSQ), the patient is asked to stand for 3 minutes and then squat for 1 minute, which causes an abrupt increase in BP. An attenuated baroreflex response with HRSQ has been shown in adult diabetic patients with autonomic neuropathy (6,7). Another maneuver, which involves starting in a squatting position, then standing for 1 minute can be used to evaluate the baroreflex response for initial orthostatic hypotension (8). There are no published normal values for children.
HR Variability
Measures of HR variability (HRV) are acquired noninvasively through monitoring of electrocardiogram (ECG) for various intervals (from 5 minutes to 24 hours) and analyzing R-R intervals. HRV can be reported as time domain or frequency parameters. HRV reflects the neural influences upon HR at the level of the cardiac pacemaker (sinoatrial node), which are due to naturally opposing sympathetic and parasympathetic signals. These measures are influenced by physiologic state; so, interpretation of HRV values should be made with consideration of behavioral state: active versus quiet sleep and awake versus asleep state. It has been shown that HRV varies with age (8,9), reinforcing the importance of broadly applicable age-matched normal reference values (2,5). HRV can also be evaluated through visualization of Poincaré plots (scatter plots of successive R-R intervals). Decreased HRV, a sign of autonomic dysfunction, would demonstrate a narrow scatter pattern as opposed to a normal “ice cream cone” pattern indicating increased variability at slower HRs with longer R-R intervals (Figure 14.2).
Adrenergic
Head-Up Tilt
Tilt table testing has been widely used by cardiologists and physiologists for the evaluation of orthostatic tolerance. All protocols require that the lower extremities be passively restrained with feet planted on a footboard so that no muscle tension is required to maintain the upright posture as this would affect the physiologic status. Generally with head-up tilt (HUT), the patient is initially at rest, supine for 10 to 30 minutes, then passively elevated (motorized tilt table) to 70° HUT. HR, BP, and clinical status are then monitored for another 10 to 30 minutes. HUT performed in autonomic laboratories tends to be of shorter duration and performed without intravenous (IV) pharmacologic challenges typically used in cardiology testing. Adolescents without history of orthostatic intolerance experience a higher incidence of near fainting during cardiac HUT than adults, particularly when IV lines are placed and IV infusions are used (10,65). Recent studies have demonstrated that the 95th percentile for HR increment was 43 bpm in 106 healthy children from 8 to 19 years of age. While the HR increment was mildly higher in children referred for orthostatic intolerance, there was considerable overlap between controls and patients (11). Galland and coworkers have published data on young infants who had simulated HUT testing while propped in HUT within their prams (12). They observed the effect of a 60° HUT on 60 full-term infants who were studied at 1 and 3 months of age. They noted that the HRV increased between 1 and 3 months of age, was reduced in prone as compared to supine sleeping position, and higher in active sleep compared to quiet sleep. There was no influence of a maternal history of cigarette smoking.
FIGURE 14.2 Poincaré plots. CCHS (left graph): narrow “cigar”-shaped plot (first reported by Marilyn Woo); normal child (middle graph): “ice cream cone”-shaped plot with labels indicating higher heart rates in circle to left, lower heart rates in circle to right, “line of identity” in red; ROHHAD (right graph): narrow plot, note decreased heart rate variability at lower heart rates.
Abbreviations: CCHS, congenital central hypoventilation syndrome; ROHHAD, rapid-onset obesity with hypothalamic dysfunction, hypoventilation, and autonomic dysregulation.
This data emphasizes the need for the development of normative data and unique diagnostic criteria in children and adolescents (11). Clearly, using adult norms (with 20-bpm increase in HR with upright posture) would create a significant pool of “false-positive” HUT in adolescent subjects.
Our autonomic laboratory monitors oxygen saturation, end-tidal CO2, respiratory rate, and regional cerebral perfusion (rSO2) via near-infrared spectroscopy (NIRS) as well as beat-to-beat HR and BP during our HUT. These data are very useful as changes in physiology and tendency to progress to syncope have been reported with hyperventilation. Martinon-Torres and colleagues (13) monitored end-tidal CO2 during HUT in 34 children (mean age 10 yrs) referred for evaluation of syncope and noted that the time from onset of tilt and from onset of clinical symptoms to syncope were both significantly longer in children who were noted to hyperventilate. It was felt that this might identify a subgroup of children who would respond differently to preventive measures. Rao (14) demonstrated failure of cerebral autoregulation in syncope by monitoring NIRS during HUT. In our laboratory, we have demonstrated poor cerebral perfusion during postural orthostatic tachycardia syndrome (POTS)-associated “foggy” mental status with preserved peripheral BP. Figure 14.3 demonstrates the HR, BP, and cerebral perfusion responses to HUT in several adolescents with orthostatic intolerance. Note the wide swings in monitored vital HR and BP in the first and second set of tracings, which are frequently noted in POTS. The third set of tracings demonstrates failure of cerebral autoregulation in an adolescent with POTS.
Valsalva Maneuver (BP Response During Phases II and IV)
A stereotyped Valsalva maneuver (see description under “Valsalva Ratio”) can also provide information regarding adrenergic function. In normal individuals, four phases of change in BP are observed: Late phase II and phase IV provide the most information about adrenergic function. Early phase II consists of a decline in BP followed by late phase II, which is an alpha-adrenergic–mediated arterial vasoconstriction leading to recovery of BP toward/above baseline levels. Phase IV consists of a BP overshoot of baseline (beta-adrenergic mediated) followed by gradual return of BP to baseline. With the Valsalva maneuver, BP is affected by a combination of mechanical factors such as intrathoracic and intra-abdominal pressure changes, changes in peripheral vascular resistance, as well as cardiovagal and adrenergic tone. Insight regarding cardiac adrenergic tone can be obtained from qualitative assessment of the arrest of the fall and subsequent increase in BP in late phase II and assessment of the increase in BP (with overshoot past baseline) seen in phase IV. There is limited experience in evaluating this phenomenon in children, as the youngest (and most ill) children have more difficulty in maintaining the required expiratory pressure for 15 continuous seconds. The two lower lines on Figure 14.4 outline systolic and diastolic BP during a Valsalva maneuver in a 7-year-old girl, with arrows noting late phase II and phase IV changes in BP demonstrating intact adrenergic function in this young girl.
Sympathetic Skin Response
The sympathetic skin response (SSR) reflects the degree of activity in the sympathetic nervous system and is based on skin conductance that can be recorded over the palm of the hand and the sole of the foot in response to sensory or electrical stimuli (see Figure 14.5). SSR has been used to establish hearing thresholds after cochlear implants (15) as well as to monitor response to enzyme replacement therapy in individuals with Fabry’s disease (16). Assessing SSR with different stimuli (electrical, thermal, inspiratory gasp, or auditory) has been used to objectively identify the preserved sensory fibers in familial dysautonomia (FD or HMSN III) (17) Many factors can affect the SSR such as skin temperature and stimulus strength as well as the patient’s emotional state and ability to be surprised (18). A limitation of this test is that the response habituates and tends to be “all or none” rather than a graded response.
Sudomotor
Quantitative Sudomotor Axon Reflex Test
Quantitative sudomotor axon reflex test (QSART) was developed in the Autonomic Laboratory at Mayo Clinic, Rochester, and provides information about sweat glands and postganglionic sympathetic nerve fibers. The QSWEAT is a commercially available, Food and Drug Administration (FDA)-approved device (based on QSART technique), which measures evoked sweat volumes. QSWEAT requires surface placement of quarter-sized disks through which a low-intensity constant current stimulus is passed for iontophoresis of acetylcholine through the skin and stimulation of sudomotor sympathetic axons. The impulse travels antidromically to an axon branch point and then orthodromically back down to synapse on the muscarinic receptor on an eccrine sweat gland. The same capsule is used to provide acetylcholine for iontophoresis, guide the constant current stimulus, and collect data regarding the volume of local sweat production. Capsules are typically placed at four sites (foot, distal leg, proximal leg, and forearm) and simultaneous stimulation provides information regarding sudomotor function along the extremities at various postganglionic axonal lengths (1). However, it is possible to have focal or regional sudomotor dysfunction that will not be identified with this technique. Sweat volumes are affected by age, gender, hydration status, and anticholinergic medication. These techniques have been used diagnostically in children down to 2 years of age (personal observation). However, normative data has not been systematically developed for individuals less than 20 years of age. Staiano et al. reported forearm QSART responses of 2.8 ± 1.3 mV amplitude in 11 healthy children between 4 and 10 years of age and 2.9 ± 1.2 mV amplitude in eight healthy children from 10 to 17 years of age (3).
FIGURE 14.3 (A) Vasovagal response. Ten-year-old male with progressive decline in HR, BP, and cerebral regional saturation values in association with abdominal pain, nausea, and poor color/perfusion of face and neck. (B) Postural orthostatic tachycardia syndrome (POTS). Ten-year-old female demonstrates a 45-bpm increase in HR from baseline with stable BP during HUT. (C) POTS with drop in cerebral perfusion. Fourteen-year-old female demonstrating a regional cerebral perfusion (rSO2) decrease in the first minute of HUT and then rebounding above baseline after return to the horizontal position.
Abbreviations: BP, blood pressure; HR, heart rate; HUT, head-up tilt; NIRS, near-infrared spectroscopy.
FIGURE 14.4 Upper tracing records heart rate and two lower tracings record beat-to-beat changes in blood pressure during voluntary Valsalva maneuver in a 7-year-old girl.
Thermoregulatory Sweat Test
The thermoregulatory sweat test (TST) is a sensitive, established autonomic test of central and peripheral sympathetic sudomotor function. By applying moisture-sensitive powder to the entire anterior aspect of a body, sweat production over the forehead, lower face, torso, and extremities can be evaluated (see Figure 14.6) (19). It has proven value in the diagnosis of small-fiber neuropathy, autonomic failure, focal and multifocal neuropathies, and ganglionopathies. The utility of this test in the evaluation of pediatric disorders has not been well studied. However, in 2011, Kuntz et al. reviewed the experience at Mayo Clinic, Rochester, relating to TST in over 200 children 2 to 16 years of age—demonstrating that TST was well tolerated in children and adolescents with valid, clinically relevant results obtained in 93.5% of the patients studied (20). TST, in children, was particularly useful in the evaluation of small-fiber peripheral neuropathies, myelopathies, multifocal or patchy peripheral nerve involvement, and disorders of sweating including anhidrosis and hyperhidrosis. In children and adolescents, TST was valuable for the assessment of small-nerve fiber function as it required less cooperation than quantitative sensory testing and was less invasive than multiple epidermal nerve fiber layer biopsies. Figure 14.7 demonstrates how TST can define abnormalities of sweating in children.
FIGURE 14.5 Sympathetic skin response from right hand and foot with left median nerve stimulation.
FIGURE 14.6 Fifteen-year-old female with distal anhidrosis suggestive of distal sudomotor or small-fiber nerve dysfunction.
Pupillography
Actions of the smooth muscles of the iris cause changes in pupil size. Pupillometry provides noninvasive, detailed measures of pupil size and response to light, with pupil dilation and constriction, respectively, indicating relative sympathetic and parasympathetic responsiveness (Figure 14.8). Daluwatte and colleagues (63,64) have published normative values for pupillary responses in children. Accordingly, quantitative pupil measurements have recently been used to evaluate ANSD in various conditions including autonomic neuropathies (21,22), enuresis (23), heart failure (24), diabetes (25,26), and pediatric autonomic disorders such as FD (27) and congenital central hypoventilation syndrome (CCHS) (28).
FIGURE 14.7 Examples of thermoregulatory sweat test in children with excessively sweaty hands and feet.
FIGURE 14.8 Parasympathetic and sympathetic pathways involved in pupillary control.
Source: Adapted from Patwari PP, Stewart TM, Rand CM, et al. Pupillometry in Congenital Central Hypoventilation Syndrome (CCHS): Quantitative evidence of autonomic nervous system dysregulation. Pediatr Res. 2012;71(3):280–285, copyright 2012 by International Pediatric Research Foundation, Inc. (IPRF).
Studies investigating the use of pupillometry have often used a 30- to 40-millisecond duration light flash (29,30); however, there is the need for a longer flash in some groups of patients. Patients with autoimmune autonomic ganglionopathy (AAG) have been found to have premature pupil redilation (31). These patients require a 2-second flash.
Use of pupillometry to measure autonomic regulation requires attention to ambient room light and ocular pathology or anterior visual pathway disease, which alter the afferent pathways. The patient must be cooperative (able to maintain stable eye position during the recording). Environmental triggers (loud noises or any type of stimulation that can cause anxiety or fear) can affect the sympathetic/parasympathetic balance.
Enteric
The process of eating (from perception of food through digestion to defecation) requires intact autonomic (sympathetic, parasympathetic, and enteric) function. Salivary secretion is under both sympathetic and parasympathetic control with inhibition and stimulation of salivary gland output, respectively. As the parasympathetic system is activated, gastric and pancreatic secretion increases. With elegant coordination, enteric motility is also enhanced. Most often, altered enteric autonomic function becomes apparent through altered gastrointestinal (GI) motility: delayed or enhanced stomach or intestinal transit time with symptoms of vomiting, early satiety, abdominal distention, abdominal pain, diarrhea, or constipation.
The options for the evaluation of GI transit are increasing as technology advances. For the purposes of this review, we will focus on GI emptying studies and colonic transit studies that can be performed in the pediatric patient. An excellent review of the adult clinical experience with autonomic testing of GI function has been recently published (32).
In children, differential diagnosis needs to include potential congenital anomalies. In children with constipation, GI dysmotility, or unexplained abdominal pain, Hirschsprung’s disease (HSCR) (absence of ganglion cells due to failure of migration) needs to be considered. Evaluation for HSCR starts with barium enema to determine the presence and location of a transition zone. If barium enema is suggestive of HSCR, then rectal biopsy is indicated to identify the absence of ganglion cells. A subset of children with HSCR have been found to have signs of ANSD relating to pupillary, cardiovascular, and sudomotor function (3). Further, HSCR can be found in pediatric autonomic disorders, such as in CCHS (33).
Gastric Scintigraphy
For scintigraphy, the patient is required to ingest a standardized amount of radiolabeled solid or liquid material (mixed with palatable items) with sequential imaging to determine the rate of emptying. Gastric scintigraphy would be indicated for the evaluation of unexplained vomiting, early satiety, or food intolerance suggestive of gastroparesis. Small-bowel and colonic scintigraphy can be performed but require longer duration of evaluation and lack standardization with limited normative data even in adults.
Colonic Transit With Radiopaque Markers
Ingestion of radiopaque markers is in use for the evaluation of colonic transit time by following the number of retained markers via abdominal radiograph over several days. Normative values are available for children (34–36).
Urologic
Urodynamic studies including the measurement of postvoid residual volume can be performed in children and adolescents to evaluate sympathetic and parasympathetic function. Recent articles outline the use of videourodynamic studies and uroflow/electromyography (EMG) for differentiating between common voiding disorders in children (37,38). Most interestingly for those interested in autonomic dysfunction in children and adolescents, the commonly observed voiding disorders, which occur without identifiable anatomic lesions of the spinal cord or spinal roots, are termed “nonneurogenic voiding disorders.” These disorders appear to involve poor coordination or balance between activation of the bladder smooth muscle, bladder neck, urinary sphincters, and pelvic floor. These would logically be disorders involving some combination of dysfunction in the central and peripheral autonomic pathways controlling voiding. Since “nonneurogenic” is a misleading term, these disorders could be considered autonomic disorders affecting voiding rather than nonneurogenic voiding disorders (personal observation).
Vascular
Skin Wrinkling
The clinical phenomenon of glabrous skin with sweat glands (finger tips and soles of the feet) wrinkling or “pruning” in response to prolonged immersion in water has been observed for decades—with the first report as a potential test of autonomic function in 1977 (39). Recent studies in adults have demonstrated that this is a sympathetically mediated vasoconstrictive phenomenon (40,41). Other studies have replicated the same phenomenon by placing a topical anesthetic such as EMLA™ under an occlusive dressing (42,43). None of these methods have been studied systematically in children or adolescents.
OTHER CLINICAL APPLICATIONS
Identification of Risk Factors for Autonomic Dysregulation
Johansson et al. (44) documented increased sympathoadrenal activity in childhood (fractionated urinary catecholamines, higher baseline HR and greater increment in HR after mental arithmetic/stress test) in healthy children with a history of preterm or small-for-gestational-age (SGA) birth.
EARLY DETECTION AND MONITORING OF COMPLICATIONS OF SYSTEMIC DISEASE
Diabetes
Varechova and colleagues (45) demonstrated a decreased threshold to capsaicin-induced cough reflex in diabetic children with subclinical autonomic neuropathy and have suggested this as a screening test for autonomic neuropathy in diabetic children.
Cahill and colleagues (46) studied pupillography and response to pilocarpine (0.1%) and cocaine (4%) in 72 children with type I diabetes, 60 children with type II diabetes, and 120 age-matched controls. Smaller dark-adapted pupil size and denervation hypersensitivity to pilocarpine were noted in the diabetic patients even when VR testing of cardiovagal function was normal. This was recommended as an inexpensive way to detect early diabetic autonomic neuropathy (DAN).
Boysen et al. (47) tested 20 patients with diabetes I between 10 and 19 years of age and report that HRV measures and baroreflex sensitivity (BRS) were more sensitive at detecting abnormalities in diabetic patients than HRDB or the 30:15 ratio. They speculated that incomplete cooperation or effort in some of the 136 control children studied may have led to the abnormalities noted with the later studies.
It remains to be seen whether early signs of autonomic dysregulation can be identified and used as a target for improved diabetic control in the pursuit of decreased long-term cardiac and renal complications of diabetes.
Screening for Other Systemic Illnesses
Trypanosomiasis
Bowman and colleagues (4) studied 38 children with confirmed but presymptomatic Trypanosoma cruzi infection in Peru and demonstrated blunted cardiovagal responses as compared to control children for the Valsalva maneuver (ratio of longest to shortest R-R interval), the cold pressor, and orthostatic tests. These simple, community-based tests requiring no expensive or high-technology equipment could serve well for screening programs.
Behcet’s Disease
Borman et al. (48) documented abnormalities in HR variability and SSR in 70 Behcet’s disease patients (primarily adults). While these abnormalities were primarily subclinical, there was a correlation between these changes and elevation of inflammatory markers, suggesting a relationship with disease activity.
Wilson’s Disease
Soni et al. (49) studied 30 patients (mean age 19 years, range 11–47) with Wilson’s disease and noted that 70% reported at least one autonomic symptom such as abnormal sweating, heat intolerance, early satiety, constipation, postural dizziness, bladder incontinence, palpitation, syncope, diarrhea, and dry mouth/dry skin. On testing, they noted increased HR at rest and decreased VRs and HRDB. The SSR latency was significantly higher, and 10% of this cohort had absent SSR in at least one extremity. Parasympathetic and sympathetic deficits were both observed, with the former more prominent.
Asthma
Emin et al. (50) demonstrated a direct relationship between the severity of atopic asthma and the degree of abnormality in HRV and SSR in 77 children from 7 to 12 years old. Autonomic testing was suggested as a cost-effective manner to assess disease severity in cases of pediatric asthma.
High BP
Fitzgibbon et al. (51) studied 11- to 14-year-old children with high BP (HBP) (1) and normal BP (85) and calculated high-frequency (HF) and low-frequency (LF) power spectrum and BRS from 5 minutes of beat-to-beat BP and HR data. Children with HBP (> 95th percentile but not clinically hypertensive) demonstrated reduced autonomic regulation.
Obstructive Sleep Apnea
Montesano et al. (52) evaluated 18 children between 6 and 16 years of age who were undergoing evaluation for obstructive sleep apnea (OSA) and compared their autonomic test results (VR, HRDB, HR 30:15, HUT BP response) with age-matched controls. The children with OSA demonstrated higher baseline BP and HR, greater increments in BP with HUT, and lower HR variability with deep breathing. These findings suggest an increase in sympathetic tone in children with OSA, and long-term implications need to be further evaluated.
IDENTIFYING UNDERLYING PATHOPHYSIOLOGY OF CLINICAL DISORDERS
Harlequin Syndrome/Flushing
Bremner and colleague (62) evaluated pupillary responses in 38 patients with Harlequin syndrome and noted Horner syndrome ipsilateral to the side with impaired facial sweating and flushing in two-thirds of the patients. Ten percent of the patients had a previously undetected mass lesion in the chest or neck presumably affecting the superior cervical ganglion or sympathetic chain.
Cyclic Vomiting Syndrome
Chelimsky et al. (53) studied six children between 6 and 16 years of age with classical cyclic vomiting syndrome and noted normal HRDB and Valsalva maneuver. Sudomotor (QSART) testing was abnormal in all patients. Response to HUT demonstrated greater than 30 bpm increase in HR in all six children and a vasodepressor change in BP in three who developed dizziness. Autonomic testing therefore demonstrated sudomotor and sympathetic abnormalities, but not parasympathetic abnormalities, in a small cohort of children with cyclic vomiting syndrome.
Syncope/Orthostatic Intolerance
Martinon-Torres and colleagues (13) monitored end-tidal CO2 during HUT in 34 children (mean age 10 yrs) referred for evaluation of syncope and noted that the time from onset of tilt and from onset of clinical symptoms to syncope were both significantly longer in children who were noted to hyperventilate. It was felt that this might identify a subgroup of children who would respond differently to preventive measures.
Singer et al. (11) performed an important study, which recruited 106 individuals under 20 years of age with good health and compared their HUT results (usually 5 minutes of tilt though a subset was tilted out to 10 minutes) with those of 654 patients of the same age who had been referred for testing due to clinical problems relating to orthostatic intolerance or lightheadedness. They observed that the HR increment in normal control children and adolescents was higher than previously estimated: The 95th percentile for the 5-minute orthostatic HR increment among normal controls was 43 bpm using HR averaged over 30 seconds. Further, an excessive orthostatic HR was considered to be 130 to 140 bpm in children 13 years of age or younger and greater than 120 bpm in adolescents 14 years and older. The authors suggested using the term “orthostatic symptoms without tachycardia” for symptomatic individuals who did not meet these criteria.
Antiel and colleagues (54) studied 31 patients between 12 and 17 years of age who presented with dyspepsia. A 10-minute HUT was performed as part of their clinical evaluation. Fifty percent of the 21 patients who demonstrated at least a 30-bpm increase in HR with HUT reported orthostatic dizziness as a daily complaint, while only 30% of the 10 patients with lower increases in HR with HUT reported orthostatic symptoms. The severity of the clinical GI symptoms and the results of gastric emptying did not relate to the amount of orthostatic tachycardia. While there is overlap between individuals with GI dysmotility and orthostatic intolerance, it is not universal and the magnitude of symptoms do not vary directly within an individual.
Longin et al. (55) studied 55 children with neurocardiogenic syncope and presyncope from 5 to 15 years of age and compared results of orthostatic testing in response to a 5-minute HUT and to 5 minutes of active standing with results from age-matched controls. HRV measures differed significantly from controls during tilt testing, with no overlap between LF power and total power calculated in control children and those with a history of neurocardiogenic syncope.
Characterization of Fiber-Type Involvement in Peripheral Neuropathies
Hilz (56) and colleagues demonstrated SSR changes in 13 patients with FD with different sensory stimuli used to evoke the responses. In patients with FD, preserved SSR in response to electrical stimuli with impaired or absent response to thermal stimuli confirmed small-fiber dysfunction.
Houlden et al. (57) studied 131 individuals with a wide spectrum of inherited peripheral neuropathies. Pupillary abnormalities were rare in hereditary neuropathy with liability to pressure palsy (HNPP) and Charcot-Marie-Tooth (CMT) I, but CMT II showed frequent and varied pupillary dysfunction. This was felt to be a differentiating finding between these two types of inherited neuropathy. Individuals with Refsum’s disease and hereditary sensory and autonomic neuropathies also had abnormalities on pupillary testing.
Detecting Small-Fiber Dysfunction—Particularly as Cause of Pain Syndromes in Children and Adolescents
Oaklander and Klein (58) studied 41 consecutive children and adolescents with debilitating, unexplained widespread pain syndromes. A high fraction of autonomic tests were abnormal in this group (27% with decreased HRDB, 42% with abnormal Valsalva maneuver, 75% with abnormal HUT, and 82% with abnormal QSART). Only two patients had a sural nerve biopsy; both (100%) were abnormal, and 11 of 37 (30%) who underwent skin biopsies for determination of nerve fiber density were abnormal. The noninvasiveness of the autonomic tests as well as the ability to repeat testing to assess treatment response strongly recommended consideration of autonomic testing for the evaluation of significant, unexplained widespread pain syndromes.
Abdominal Pain or GI Dysmotility
One recently described syndrome, the “4A” syndrome (adrenocortical insufficiency associated with achalasia, alacrima, and autonomic symptoms) can present with either abdominal pain/gastroesophageal motility problems or orthostatic intolerance (59). This can present from preschool years through adult life and has been associated with mutations in AAAS, a GTP-binding protein-linked hormone receptor. In addition to the symptomatic GI and orthostatic issues, patients have been demonstrated to lack normal HRV on HRDB and VR testing and to have abnormalities of pupillary responsiveness.
Safder and colleagues (60) evaluated 76 children with functional abdominal pain on tilt table testing. They noted that the group of children whose symptoms were reproducible on HUT benefited more from treatment with fluids and fludrocortisone than those whose symptoms were not reproduced on the tilt test.
Safder et al. (61) recorded surface EMG over the gastric region (electrogastrography) in 49 adolescents undergoing HUT testing (25 meeting criteria for POTS and an age-matched group that did not meet POTS criteria). The two groups did not differ with respect to supine gastric activity. However, the adolescents meeting POTS criteria had significantly increased gastric activity in the upright HUT position. This data suggests an etiologic mechanism for the abdominal symptoms in children with POTS.
FUTURE DIRECTIONS
Using new technologies to develop faster, less intrusive methods of measuring autonomic function will be very useful in infants, children, and adolescents. This clearly has to be followed by collection of data from adequate cohorts of healthy children to allow precision in the interpretation of developmental changes over growth and aging.
SUMMARY
Many clinical disorders involve changes in the autonomic nervous system. It is important that pediatricians and pediatric specialists have the support available to evaluate, diagnose, and monitor autonomic involvement in the myriad of appropriate clinical problems that occur in children. While autonomic testing has an important role in detecting, diagnosing, and monitoring treatment, it is critical that it be performed using well-established protocols with adequate normative controls to facilitate interpretation.
REFERENCES
1. Low PA, Sleeten DM. Laboratory evaluation of autonomic failure. In: Low PA, Benarroch EE, eds. Clinical Autonomic Disorders. 3rd ed. Philadelphia, PA: Lippincott-Raven;2008:130–160.
2. Longin E, Dimitriadis C, Shazi S, et al. Autonomic nervous system function in infants and adolescents: impact of autonomic tests on heart rate variability. Pediatr Cardiol. 2009;30:311–324.
3. Staiano A, Santoro L, DeMarco R, et al. Autonomic dysfunction in children with Hirschsprung’s disease. Digestive Dis Sci. 1999;44(5):960–965.
4. Bowman NM, Kawai V, Gilman RH, et al. Autonomic dysfunction and risk factors associated with Trpanosoma cruzi infection among children in Arequipa, Peru. Am J Trop Med Hyg. 2011;84(1):85–90.
5. Longin E, Schaible T, Lenz T, König S. Short term heart rate variability in healthy neonates: Normative data and physiologic observations. Early Hum Dev. 2005;81(8):663–671.
6. Marfella R, Giugliano D, diMaro G, et al. The squatting test. A useful tool to assess both para-sympathetic and sympathetic involvement of the cardiovascular autonomic neuropathy in diabetes. Diabetes. 1994;43:607–612.
7. Nakagawa M, Shinohara T, Anan F, et al. New squatting test indices are useful for assessing baroreflex sensitivity in diabetes mellitus. Diabet Med. 2008;25(11):1309–1315.
8. Weiling W, Krediet CTP, van Dijk N, et al. Initial orthostatic hypotension: review of a forgotten condition. Clin Sci(Lond). 2007;112:157–165.
9. Silvetti MS, Drago F, Ragonese P. Heart rate variability in healthy children and adolescents is partially related to age and gender. Int J Cardiol. 2001;81(2–3):169–174.
10. deJong-deVos van Steenwijk CC, Wieling W, Johannes HM, et al. Incidence and hemodynamic characteristics of near-fainting in healthy 6–16 year old subjects. J Am Coll Cardiol. 1995;25(7):1615–1621.
11. Singer W, Sletten DM, Opfer-Gehrking TL, et al. Postural tachycardia in children and adolescents—what is abnormal? J Pediatr. 2012;160(2):222–226.
12. Galland BC, Hayman RM, Taylor BJ, et al. Factors affecting heart rate variability and heart rate responses to tilting in infants aged 1 and 3 months. Pediatr Res. 2000;48(3):360–368.
13. Martinon-Torres F, Rodriguez-Nunez A, Fernandez-Cebrian S, et al. The relation between hyperventilation and pediatric syncope. J Pediatr. 2001;138(6):894–897.
15. Péréon Y, Laplaud D, Nguyen TT, Radafy E. A new application for the sympathetic skin response: the evaluation of auditory thresholds in cochlear implant patients. Clin Neurophysiol. 2001;112(2):314–318.
16. Jardim LB, Gomes I, Netto CB, et al. Improvement of sympathetic skin responses under enzyme replacement therapy in Fabry disease. J Inherit Metab Dis. 2006;29:653–659
17. Hilz MJ, Axelrod FB, Schweibold G, Kolodny EH. Sympathetic skin response following thermal, electrical, acoustic, and inspiratory gasp stimulation in familial dysautonomia patients and healthy persons. Clin Auton Res. 1999;9(4):165–177.
18. Vertugno, R, Liguori, R, Montagna, P, Cortelli, P. Sympathetic skin response: Basic mechanisms and clinical applications. Clin Auton Res. 2003;13:256–270.
19. Fealey RD. Thermorgulatory Sweat Test. In: Low PA, Benarroch EE, eds. Clinical Autonomic Disorders. 3rd ed. Philadelphia, PA: Lippincott-Raven;2008:245–257.
20. Kuntz NL, Fealey RE. Utility of diagnostic thermoregulatory sweat test in children. Neurol. 2010;76(9 Suppl 4):A 575.
21. Bremner F, Smith S. Pupil findings in a consecutive series of 150 patients with generalised autonomic neuropathy. J Neurol Neurosurg Psychiatry. 2006;77(10):1163–1168
22. Bremner F. Pupil evaluation as a test for autonomic disorders. Clin Auton Res. 2009;19(2):88–101.
23. Dundaroz R, Turkbay T, Erdem U, et al. Pupillometric assessment of autonomic nervous system in children with functional enuresis. Int Urol Nephrol. 2009;41(2):231–235.
24. Keivanidou A, Fotiou D, Arnaoutoglou C, et al. Evaluation of autonomic imbalance in patients with heart failure: a preliminary study of pupillomotor function. Cardiol J. 2010;17(1):65–72.
25. Maguire AM, Craig ME, Craighead A, et al. Autonomic nerve testing predicts development of complications; A 12 year followupstudy. Diabetes Care. 2007;30(11):77–82.
26. Karavanaki-Karanassiou K. Autonomic neuropathy in children and adolescents with Diabetes Mellitus. J Pediatr Endocrinol Metab. 2011;14 Suppl 5:1579–1586.
27. Dutsch M, Hilz MJ, Rauhut U, et al. Sympathetic and parasympathetic pupillary dysfunction in familial dysautonomia. J Neurol Sci. 2002;195(1):77–83.
28. Patwari PP, Stewart TM, Rand CM, et al. Pupillometry in Congenital Central Hypoventilation Syndrome (CCHS): Quantitative evidence of autonomic nervous system dysregulation. Pediatr Res. 2012;71(3):280–285.
29. Muppidi S, Adams-Hunt B, Tajzoy E, et al. Dynamic pupillometry as an autonomic testing tool. Clin Auton Res. 2013;23:297–303.
30. Boev A, Fountas K, Karampelas I, et al. Quantitative pupillometry: normative data in healthy pediatric volunteers. J Neurosurg. 2005;103(6 Suppl):496–500.
31. Muppidi S, Schribner M, Gibbons C, et al. A Unique manifestation of pupillary fatique in autoimmune autonomic ganglionopathy. Arch Neurol. 2012;69(5):644–648.
32. Rao SS, Camilleri M, Hasler WL, et al. Evaluation of gastrointestinal transit in clinical practice: position paper of the American and European Neurogastroenterology and Motility Societies. Neurogastroenterol Motil. 2011;23(1):8–23.
33. Weese-Mayer DE, Berry-Kravis EM, Ceccherini I, et al. An official ATS Clinical Policy Statement: Congenital central hypoventilation syndrome: Genetic basis, diagnosis, and management. Am J Respir Crit Care Med. 2010;181(6):626–644.
34. Casasnovas AB, Cives RV, Jeremias AV, et al. Measurement of colonic transit time in children. J Pediatr Gastroenterol Nutr. 1991;13(1):42–45.
35. Sutcliffe JR, King S, Hutson JM, Southwell B. What is new in radiology and pathology of motility disorders in children? Semin Pediatr Surg. 2010;19(2):81–85.
36. Wagener S, Shankar KR, Turnock RR, et al. Colonic transit time—what is normal? J Pediatr Surg. 2004;39(2):166–169.
37. Glassberg K, Combs AJ, Horowitz M. Non-neurogenic voiding disorders in children and adolescents: Clinical and Videourodynamic findings in 4 specific conditions. J Urol. 2010;184(5):2123–2127.
38. Van Batavia JP, Comb AJ, Hyun G, et al. Simplifying the Diagnosis of four common voiding conditions using uroflow/electromyography, electromyography lag time and voiding history. J Urol. 186(4 Suppl):1721–1726.
39. Bull C, Henry JA. Finger wrinkling as a test of autonomic function. Br Med J. 1997;1(6060):551–552.
40. Wilder-Smith EPV, Chow A. Water-immersion wrinkling is due to vasoconstriction. Muscle Nerve. 2003a;27:307–311.
41. Van Barneveld S, van der Palen J, van Putten MJ. Evaluation of the finger wrinkling test: a pilot study. Clin Auton Res. 2010;20(4):249–253.
42. Wilder-Smith E, Chow A. Water immersion and EMLA cause similar digit skin wrinkling and vaso-constriction. J Microvasc Res. 2003b;66:68–72.
43. Wilder-Smith EP, Guo Y, Chow A. Stimulated skin wrinkling for predicting intraepidermal nerve fibre density. ClinNeurophysiol. 2009;120(5):953–958.
44. Johansson S, Norman M, Legnevall L, et al. Increased catecholamines and heart rate in children with low birth weight: perinatal contributions to sympathoadrenal overactivity. J Intern Med. 2007;261(5):480–487.
45. Varechova S, Durdik P, Cervenkova V, et al. He influence of autonomic neuropathy on couge reflex sensitivity in children with diabetes mellitus type 1. J Physiol and Pharmacol. 2007;58 Suppl 5:705–715.
46. Cahill M, Eustace P, deJesus V. Pupillary autonomic denervation with increasing duration of diabetes mellitus. Br J Ophthalmol. 2001;85(10):1225–1230.
47. Boysen A, Lewin MA, Hecker W, et al. Autonomic function testing in children and adolescents with diabetes mellitus. Pediatr Diabetes. 2007;8(5):261–264.
48. Borman P, Tuncay F, Kocaoglu S, et al. The subclinic autonomic dysfunction in patients with Behcet disease: an electrophysiological study. Clin Rheumatol. 2012;31(1):41–47.
49. Soni D, Shukla G, Singh S, et al. Cardiovascular and sudomotor autonomic dysfunction in Wilson’s disease—Limited correlation with clinical severity. Auton Neurosci. 2009;151:154–158.
50. Emin O, Esra G, Aysegul D, et al. Autonomic nervous system dysfunction and their relationship with disease severity in children with atopic asthma. Respir Physiol Neurobiol. 2012;183(3):206–210.
51. Fitzgibbon LK, Coverdale NSS, Phillips AA, et al. The association between baroreflex sensitivity and blood pressure in children. Appl Physiol Nutr Metab. 2012;37(2):301–307.
52. Montesano M, Siano S, chiari Paolino M, et al. Autonomic cardiovascular tests in children with obstructive sleep apnea syndrome. Sleep. 2010;33(10):1349–1355.
53. Chelimsky TC, Chelimsky GG. Autonomic abnormalities in cyclic vomiting syndrome. J Pediatr Gastroenterol Nutr. 2007;44(3):326–330.
54. Antiel RM, Risma JM, Grothe RM, et al. Orthostatic intolerance and gastrointestinal motility in adolescents with nausea and abdominal pain. J Pediatr Gastroenterol Nutr. 2008;46:285–288.
55. Longin E, Reinhard J, vonBuch D, et al. Autonomic function in children and adolescents with neurocardiogenic syncope. Pediatr Cardiol. 2008;29(4):763–770.
56. Hilz MJ. Assessment and evaluation of hereditary sensory and autonomic neuropathies with autonomic and neurophysiological examinations. Clin Auton Res. 2002;12 Suppl 1:133–143.
57. Houlden H, Reilly MM, Smith S. Pupil abnormalities in 131 cases of genetically defined inherited peripheral neuropathy. Eye (Lond). 2009;23(4):966–974.
58. Oaklander Al, Klein MM. Evidence of small-fiber polyneuropathy in unexplained, juvenile-onset, widespread pain syndromes. Pediatrics. 2013;131(4):1091–1100.
59. Gazarian M, Cowell CT, Bonney M, Grigor WG. The “4A” syndrome: adrenocortical insufficiency associated with achalasia, alacrima, autonomic and other neurological abnormalities. Eur J Pediatr. 1995;154(1):18–23.
60. Safder S, Chelimsky TC, O’Riordan MA, Chelimsky G. Autonomic testing in functional gastrointestinal disorders: implications of reproducible gastrointestinal complaints during tilt table testing. Gastroenterol Res Pract. 2009;2009:1–6.
61. Safder S, Chelimsky TC, O’Riordan MA, Chelimsky G. Gastric electrical activity becomes abnormal in the upright position in patients with postural tachycardia syndrome. J Pediatr Gastroenterol Nutr. 2010;51(3):314–318.
62. Bremner F, Smith S. Pupillographic findings in 39 consecutive cases of harlequin syndrome. J Neuroophthalmol. 2008;28(3):171–177.
63. Daluwatte C, Miles JH, Christ SE, et al. Age-dependent pupillary light reflex parameters in children. Conf Proc IEEE Eng Med Biol Soc. 2012;2012:3776–3779.
65. Grubb BP, Orecchio E, Kurcyzynski TW. Head-upright tilt table testing in evaluation of recurrent, unexplained syncope. Pediatr Neurol. 1992;8(6):423–427.
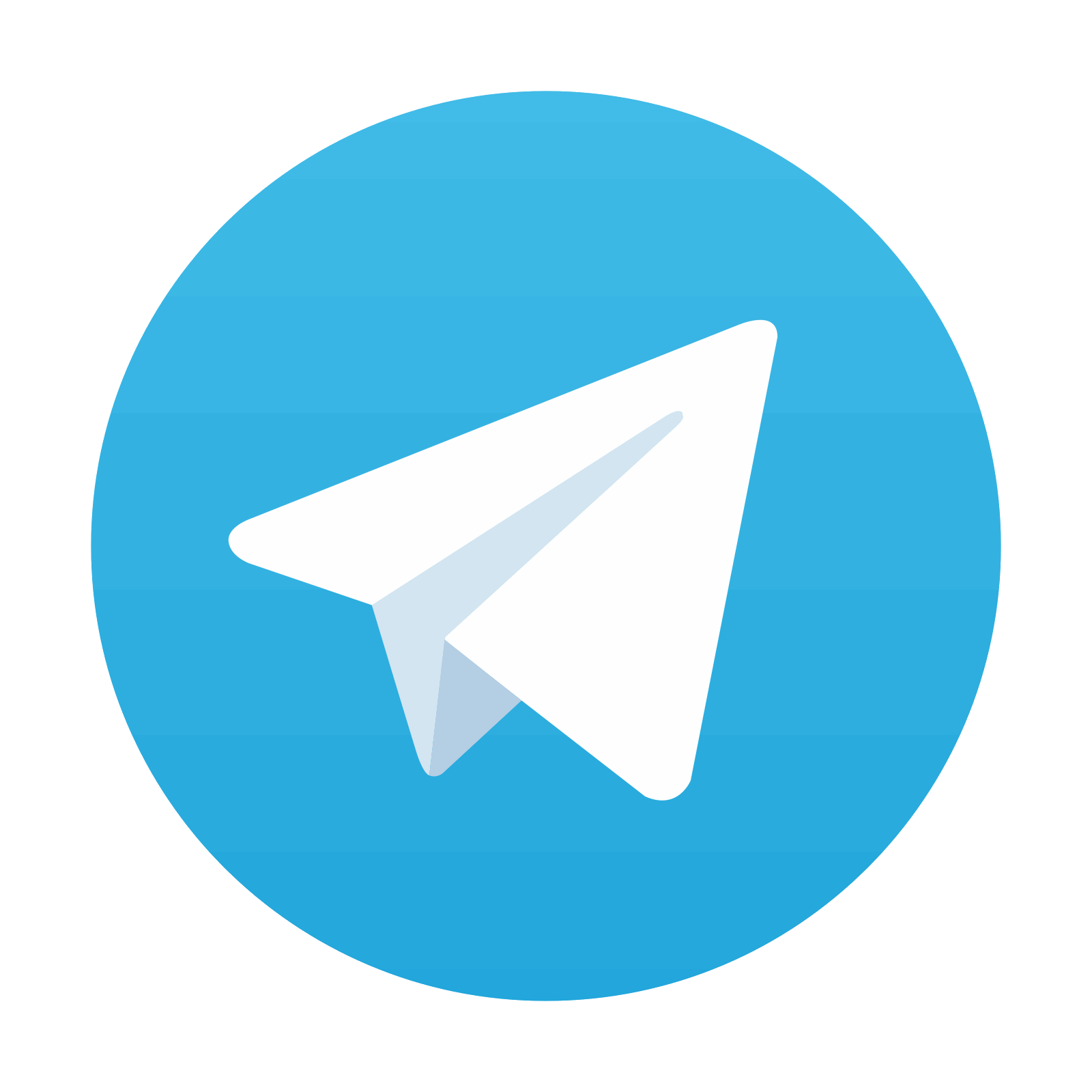
Stay updated, free articles. Join our Telegram channel

Full access? Get Clinical Tree
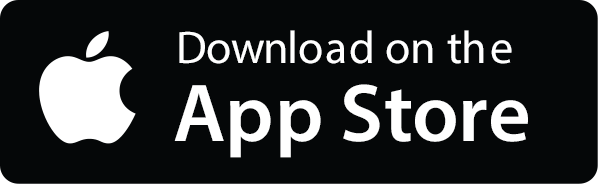
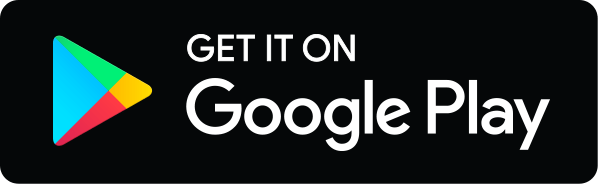