CHAPTER 91 BACTERIAL MENINGITIS
Meningitis has long been known as a clinical disorder. Unequivocal descriptions of meningitis date to the seventeenth century,1 and tuberculous meningitis and syphilitic meningitis have been known as discreet entities for nearly as long.2 In contrast, Lyme disease is a much more recently recognized entity. Prior to the advent of antibiotics, bacterial and tuberculous meningitis were, with very rare exceptions, fatal disorders, and even today, despite antibiotic therapy, both entities carry significant mortality and appreciable morbidity. The spirochetal meningitides, in contrast, usually pursue a more protracted course, and although both syphilitic or Lyme meningitis may result in significant neurological impairment, death from either condition is unusual.
BACTERIAL MENINGITIS
Epidemiology
Approximately 15,000 cases of acute bacterial meningitis occur in the United States each year.3 The overall incidence of acute bacterial meningitis has fallen over time, however, largely due to the sharp decline in early childhood cases of H. influenzae meningitis. Schlech,4 in a review of cases of meningitis in 27 states from 1978 through 1981, noted an incidence of three cases per 100,000 of population. In contrast, Shuchat and colleagues,1 in a study encompassing acute care hospitals in 22 counties of four states, found a decline in cases of meningitis from 2.9 cases per 100,000 population in 1986 to 0.2 case in 1995. In earlier studies, the most common organisms, in descending order, were H. influenzae, Neisseria meningitidis, and Streptococcus pneumoniae. By the time of Shuchat’s report, H. influenzae accounted for only 0.2% of cases. Prior to the advent of conjugated vaccines for H. influenzae type B (Hib), the median age of meningitis cases was 15 months, whereas by 1995 the median age had risen to 25 years.1 Meningitis in developed countries has thus changed from being a disease of infants to a disease of older adults. This is in sharp contrast to developing countries in which immunization against Hib has not been used: there, as in Western countries before the advent of Hib vaccination, meningitis remains a disease of early childhood, with the greatest number of cases occurring in children from 6 months to 2 years of age.5–7 A trend worldwide over this same period of time has been the emergence of clusters of meningococcal meningitis and meningococcemia, in particular within sub-Saharan Africa, with over 300,000 cases and 30,000 deaths.8 A key event in these outbreaks has been the introduction of virulent strains of meningococci into populations without strain-specific antibody.
Causative Agents
The agents causing bacterial meningitis vary with both the age of the patient (Table 91-1) and with the route by which infection is acquired (Table 91-2).
TABLE 91-1 Agents of Acute Bacterial Meningitis According to Patient Age
Age | Agent |
---|---|
Neonates | Streptococcus agalactiae: in particular type III |
E. coli and other gramnegative organisms (Proteus mirabilis, Klebsiella, Enterobacter sp., Pseudomonas aeruginosa, Citrobacter diversus, Salmonella sp.) | |
Listeria monocytogenes | |
Staphylococcus epidermidis | |
Staphylococcus aureus | |
S. pneumoniae | |
Childhood-early adulthood | N. meningitidis |
S. pneumoniae | |
S. aureus | |
Mid-adulthood | S. pneumoniae |
S. aureus | |
Old age | S. pneumoniae |
S. aureus | |
L. monocytogenes | |
Gram-negatives |
TABLE 91-2 Agents of Acute Bacterial Meningitis According to Route of Acquisition
Condition | Probable Organism(s) |
---|---|
Sinusitis or otitis | S. pneumoniae, H. influenzae, microaerophilic and anaerobic streptococci, Bacteroides, S. aureus |
Penetrating head trauma | S. aureus |
Shunt infections | S. epidermidis |
Complications of neurosurgery | Gramnegative bacteria (Klebsiella pneumonia, Acinetobacter calcoaceticus-baumannii complex, E. coli) |
Causative Agents of Meningitis by Patient Age
The most common cause of bacterial meningitis in neonates and infants is Streptococcus agalactiae (group B streptococci), followed in order of frequency by E. coli, other gram-negatives, and L. monocytogenes1,9 (see Table 91-1). Meningitis due to S. agalactiae occurs at two points in time: within the first 48 hours of the postnatal period or between 7 days and 6 weeks of age.10 Cases occurring in the immediate postnatal period represent acquisition of the agent from the mother at the time of birth, and meningitis often occurs as part of a systemic infection; cases in older infants more frequently occur as an isolated meningitis. Less common agents include Listeria monocytogenes, Staphylococcus epidermidis, Staphylococcus aureus, and S. pneumoniae. Listeria and S. aureus are discussed later. Prior to the development of an effective vaccine, H. influenzae type B was the most common cause of meningitis below the age of 6 and the most common organism overall in total number of cases. Today, H. influenzae is rarely associated with meningitis in the United States or western Europe.1,9 In areas where vaccination is not yet widely used, however, H. influenzae remains a major cause of disease. Meningitis in older children and young adults is usually caused by N. meningitidis or, less frequently, S. pneumoniae. In older adults, S. pneumoniae causes over 50% of cases, with the incidence of meningitis due to gram-negatives, Listeria, and S. aureus rising in later life.
Causative Agents According to Route of Infection
Here, meningitis is the result of extension or introduction of organisms into the subarachnoid space, by spread through emissary veins from infected sinuses or other cranial structures, penetrating trauma, or neurosurgical procedures (see Table 91-2). The most common agent associated with cases of sinusitis or otitis is S. pneumoniae. In this setting, H. influenzae may cause meningitis in adults as well as children, and infection may also be caused by anaerobic or microaerophilic organisms or by S. aureus.11 S. aureus is particularly associated with penetrating trauma. S. epidermidis tends to be associated with infection of ventricular shunts.12 S. aureus, S. epidermidis, and gram-negatives may cause meningitis in the setting of neurosurgical procedures.
Causative Agents in Immunocompromised Patients
The organisms associated with bacterial meningitis in immunocompromised patients vary with the type of immune deficiency. Individuals with defects of cell-mediated immunity, as in AIDS or Hodgkin disease, have an increased prevalence of meningitis due to L. monocytogenes.13–15 Patients with defects of humoral immune response—and patients who have undergone splenectomy—are prey to fulminant meningitis with S. pneumoniae, H. influenzae type B, and, less frequently N. meningitidis. Patients with neutropenia are susceptible to meningitis caused by Pseudomonas aeruginosa and by gramnegative enteric bacteria.16
Meningitis Due to L. monocytogenes and S. aureus
These agents deserve specific comment. L. monocytogenes differs from the other organisms previously listed in that it is an obligate intracellular parasite whose control depends on T cell–mediated immunity as well as opsonization. For this reason, L. monocytogenes appears as a cause of meningitis in three groups of patients: very young infants, especially in the setting of prematurity; patients who are immunocompromised; and the very elderly.17 S. aureus is the major agent associated with meningitis in patients with penetrating head trauma.16 In addition, however, S. aureus is a cause of meningitis in a minority of patients of all ages. Conditions in which S. aureus meningitis is particularly likely include known meningitis in the setting of endocarditis, intravenous drug abuse, burns, or superficial or deep abscesses or decubiti.
Pathogenesis and Pathology
The pathogenesis of bacterial meningitis involves three separate steps: colonization of the host, entry of bacteria across the blood-brain barrier, and development of infection within the subarachnoid space and ventricular system. Once established, bacteria may invade the central nervous system to cause meningitis via one of three routes. In the majority of cases of meningitis, colonization of the nasopharynx is a key initial event.18 Studies by Kim and coworkers19 indicate that entry of E. coli across the blood-brain barrier requires a high level of bacteremia, followed by entry of the organism into brain microvascular endothelial cells and movement across these cells into the subarachnoid space. Less frequently, meningitis may be caused by direct spread of agents from infected pericranial structures such as sinuses, middle ear, or mastoid via emissary veins. Bacteria may also enter the subarachnoid space directly, either through introduction of bacteria during penetrating trauma or, occasionally, via entry of organisms through congenital or acquired defects in the skull or spinal column.20–22
The ability of blood-borne bacteria to invade the meninges is determined to a considerable degree by properties of the bacterium itself, and many of the organisms associated with meningitis have a particular ability to adhere to mucous membranes, promoting their entry into the host’s bloodstream.20,23 Thus, most cases of S. agalactiae meningitis are associated with type III organisms; the majority of cases of E. coli meningitis are caused by those bearing the K1 capsular antigen24; virtually all cases of H. influenzae meningitis are caused by type b strains; and many cases of meningococcal meningitis are associated with those bearing groups B and C antigens. The reasons for the association of these serotypes with meningitis are not completely understood, but in each of these cases, the bacterial capsule contains sialic acid, making the organisms more closely resemble host cell surfaces; furthermore, there is antigenic cross-reactivity between the K100 antigen of E. coli, the capsular antigens of H. influenzae b, and those of group B N. meningitidis.20,23,25 Although more than 90 strains of S. pneumoniae are known to exist, only 18 of these are commonly associated with meningitis.
Despite pathogenesis studies extending back over 50 years, the actual routes by which bacteria penetrate the blood-brain barrier are still not fully defined. S. pneumoniae has been shown in vitro to bind to isolated human endothelial cells and to be detected in vacuoles within cells, suggesting that the organism may cross the blood-brain barrier via transcellular spread. Work in experimental animals with both E. coli and N. meningitidis suggests that binding of bacterial fimbriae is important in adherence of organisms to the luminal surfaces of brain microvascular endothelial cells. Transformation of organisms to nonfimbriated forms may then enhance transmural spread into the subarachnoid space.19,20,23 In vitro data suggest that neuroinvasion by E. coli involves interaction of bacterial proteins with cellular proteins present in brain but not systemic endothelia.19,26,27 In addition, certain brain regions, such as the choroid plexus, lack tight junctions, and meningitis of hematogenous origin might also begin with the entry of bacteria into brain ventricles across these structures. This hypothesis is supported both by morphological studies and by the frequent occurrence of ventriculitis as a concomitant of meningitis.28
Once within the subarachnoid space, bacteria multiply in an environment that is devoid of complement or leukocytes, and the initial stage of meningitis is essentially noninflammatory.28 Endotoxin, teichoic acid, and other products released from bacteria, however, elicit a brisk inflammatory response. This response includes not only leukocytes but also tumor necrosis factor and other chemical mediators of inflammation that alter blood-brain barrier permeability. Cerebrospinal fluid (CSF) protein concentrations rise and glucose characteristically falls, due not only to consumption of glucose by bacteria but also to altered transport of glucose across brain capillaries. Inflammation within the subarachnoid space is often accompanied by cortical encephalitis and ventriculitis, at times with hydrocephalus. Spread of inflammation into superficial areas of the brain may result in thrombosis of superficial cerebral vessels.29,30 This combination of factors results in vasogenic as well as cytotoxic cerebral edema, often complicated by arterial, venous, and capillary thrombosis, resulting in focal infarction, hemorrhage, or both. Death during the acute stages of bacterial meningitis is almost always due to brain herniation, resulting from cerebral edema and, in some cases, accompanying hydrocephalus. Delayed death or neurological disability in meningitis results from the combined effects of direct brain involvement, vascular compromise, and elevated intracranial pressure. In the process, any of several cranial nerves may be affected, in particular cranial nerve VIII. A major challenge in bacterial meningitis is that antibiotic therapy has no immediate effect on this cascade of events.
Meningitis is often viewed as a disease involving solely the meninges and, in some cases, the ventricular ependyma. The disease, however, is considerably more complex and destructive and may include communicating or obstructive hydrocephalus, cerebritis, vasculitis with axonal injury and/or arterial or venous infarcts, and actual myelitis.30–32 Actual cortical abscesses have been described in gramnegative meningitis and also may occur in cases of meningitis due to other disorders. Suppurative labyrinthitis has been described in experimental S. pneumoniae meningitis and may account for the hearing loss seen in meningitis. S. pneumoniae meningitis may also be accompanied by myelitis.30
Clinical Features
Bacterial meningitis typically presents in one of three ways.33,34 Most commonly, meningitis is preceded by 3 to 5 days of insidiously progressive symptoms of fever, malaise, irritability, or vomiting. In a smaller number of cases, meningitis develops over 1 to 2 days. In a minority of cases, bacterial meningitis begins fulminantly and may be so rapid that it remains one of the few neurological conditions capable of causing death within hours in an otherwise healthy young person. Typical symptoms are fever, headache, photophobia, and changes in mental status. Patients may or may not complain of neck stiffness, and some patients, in particular those with meningitis due to S. aureus, may complain of back pain. Seizures may occur early in meningitis in up to 40% of affected children and may also occur in adults. Presentation with focal seizures or focal neurological symptoms, however, should raise concern of brain abscess or other localized process.
Physical examination typically reveals fever, tachycardia, and nuchal rigidity.16,33 Many patients will also have altered mental status. Presentation in coma, seen in up to 12% of patients, is an ominous prognostic sign. Papilledema may be present in severe cases. Papilledema develops over time (often over 24 hours), however, and the rapid progression of meningeal infection may result in severely increased intracranial pressure before papilledema has had a chance to appear. In many patients, evidence of systemic infection is also present. Particular attention should be paid to identifying cutaneous rashes, petechiae, or purpura suggestive of meningococcemia, pulmonary consolidation, which may be present in S. pneumoniae meningitis, or cardiac murmurs suggesting endocarditis.
Tests of Meningeal Irritation
The classic tests for bacterial meningitis are resistance to passive flexion of the neck (nuchal rigidity), Kernig’s sign, and Brudzinski’s sign. Kernig’s sign represents resistance to passive extension of the leg at the knee. Brudzinski developed several tests of meningeal irritation, but the maneuver most commonly referred to as Brudzinski’s sign involves spontaneous flexion of the hips and knees when the neck is passively flexed. Both signs are strongly suggestive of meningeal irritation. Brudzinski’s sign is the more sensitive of the two, in particular where the observer takes pain to note even a slight degree of spontaneous flexion. Both signs, however, were developed long prior to the advent of antibiotic therapy, when meningitis was frequently advanced at the time of presentation, and both may be absent early in the course of illness. In awake patients, a more sensitive test is to ask the patient to put the chin on the chest with the mouth closed, because patients experiencing pain on flexion may hold the neck still but touch the chin to the chest by opening the jaw widely. Perhaps the most sensitive test of nuchal rigidity is a test developed during days of epidemic polio and involves asking the patient either to kiss the knee or to touch his or her forehead to the knee: this test will often detect meningeal irritation at a time when the other tests are negative. One should keep in mind that very elderly patients with extensive cervical spine disease may have neck stiffness, and occasional patients with influenza and severe myalgias may also complain of neck pain. In both cases, pain and resistance to movement occur not only on flexion but also on turning the head, whereas in meningitis, one can usually turn the head even if neck stiffness to flexion is present.
Atypical Presentations of Meningitis
Signs of meningeal irritation are often absent in four groups of patients: neonates, immunocompromised patients, the elderly, and patients with meningitis related to neurosurgical procedures.16 Neonates often do not exhibit nuchal rigidity, and the presence of meningitis may be signaled only by tachypnea, apneic spells, changes in heart rate, atypical seizures, or simply vague decline.10 The typical high-pitched “meningeal cry” may or may not be present. Similarly, the presence of a bulging fontanel is a late sign, indicating significantly increased intracranial pressure. Because the presentation of meningitis in neonates and infants may be so atypical, the threshold for lumbar puncture must be very low. Immunocompromised individuals, like neonates, may not develop fever or nuchal rigidity. Alcoholics, in particular those presenting in the setting of severe inebriation, may also have meningitis without clearly detectable signs. Meningitis may also be deceptively asymptomatic in the elderly, and the only sign of meningitis may be confusion in a previously alert older patient or altered responsiveness in a patient who is already demented.35,36 In these patients, threshold for lumbar puncture should also be low. However, alcoholics and elderly patients are also at risk for falls and subdural hematomas; and immunosuppressed patients may also have brain abscesses or other space-occupying lesions. In such patients, it may thus be prudent to begin appropriate antibiotics presumptively and obtain appropriate head imaging (magnetic resonance imaging [MRI] or head computed tomography [CT] scanning) before performing the lumbar puncture. The onset of bacterial meningitis following neurosurgical procedures may also be insidious, developing over hours or days. Patients in this setting are at increased risk, because alteration of consciousness or neck stiffness may be attributed to the preceding surgical intervention, and CSF cell count and chemistries are often altered by the preceding surgery.
Diagnosis
Bacterial meningitis is suspected on the basis of clinical presentation and physical findings. MRI may show cortical meningeal or meningovascular enhancement (Fig. 91-1). Definitive diagnosis, however, is almost always made on the basis of lumbar puncture. Bacterial meningitis usually produces diffuse meningeal involvement, so that relatively little brain shift may occur, even when intracranial pressure is significantly increased. Brain herniation may occur, however, if intracranial pressure is greatly increased, and the likelihood of fatal herniation cannot be predicted from CT.37,38 In severely ill patients, in whom very high intracranial pressure is suspected, the most prudent course may be to begin empirical treatment and wait until CSF pressure has been controlled before performing lumbar puncture.
Typical CSF findings are shown in Table 91-3. These include elevated pressure, fluid which is often turbid, elevated protein, depressed glucose, and elevated white blood cell count, consisting predominantly of polymorphonuclear leukocytes.39 The degree to which glucose is depressed in bacterial meningitis has been a matter of debate. Silver and Todd, in a study of 181 pediatric patients with CSF glucose levels under 50 mg/dL identified 35 patients with bacterial meningitis.40 Of these, 22 (77%) had glucose levels of 20 or below, and of 37 patients with glucose levels under 20 mg/dL, 22 (73%) had bacterial meningitis. A CSF : blood glucose ratio of less than 0.3 was highly correlated with bacterial meningitis. Bacterial meningitis was less likely if the CSF glucose levels of 20 to 50 or if the CSF/blood glucose ratio was greater than 0.30. Spanos and colleagues41 found that a CSF glucose of 18 mg/dL or less or a CSF/blood glucose ratio of less than 0.23 was associated with bacterial rather than viral meningitis in 99% of patients studied. It should be remembered, in evaluating blood glucose, that CSF glucose values will be higher in severely hyperglycemic patients and that changes in CSF glucose levels may lag 30 to 120 minutes behind those in blood. Protein levels in meningitis are a reflection of blood-brain barrier injury and usually range between 100 and 500 mg/dL.39
TABLE 91-3 Typical Cerebrospinal Fluid Findings in Bacterial Meningitis
Opening pressure | Usually elevated |
Fluid | Turbid |
Cells | >100/mm3; often >1000 cells/mm2 |
Cell type | Polymorphonuclear leukocytes |
Protein | Elevated: usually >100 |
Glucose | Depressed: usually <50% of blood glucose |
Gram’s stain | Positive in 60-80% of cases |
Not all patients with bacterial meningitis exhibit the characteristic findings listed earlier.39 CSF, especially early in the course of meningitis, may have normal cell count and chemistries yet contain bacteria. Severely immunosuppressed patients may also fail to develop CSF leukocytosis. Approximately 14% of patients with bacterial meningitis will have a CSF cellular response that is predominantly lymphocytic; CSF lymphocytosis is particularly likely in neonatal meningitis and in infections caused by L. monocytogenes.39 Although a high CSF cell count, in particular, with a large percentage of polymorphonuclear leukocytes, suggests a bacterial infection, similar CSF leukocytosis with polymorphonuclear predominance may be seen in tuberculous or fungal meningitis, spirochetal meningitis, or viral meningitis. Approximately 9% of patients with bacterial meningitis have normal CSF glucose. Prior antibiotic treatment of bacterial meningitis may have little effect on CSF cell count, glucose, and protein within the first 2 to 3 days but will reduce the yield on Gram’s stain and culture.39 In some instances, prior antibiotic treatment will cause a shift from a polymorphonuclear to a lymphocytic CSF pleocytosis.
Specific identification of the infecting organism has traditionally involved Gram’s stain and bacterial culture.39 Gram’s stain provides the most rapid initial identification of the organism. Gram’s stain will be positive in approximately 25% of cases in which the CSF contains 103 colony-forming units (CFU)/mL and 97% of cases with greater than 105CFU. Errors in Gram’s stain may result from inadequate efforts to resuspend bacteria if CSF has been allowed to settle and errors in decolorization or reading of the slide. Bacterial culture and determination of antibiotic sensitivity are routine in virtually all hospital laboratories. It may be useful, however, to review culture requirements with the laboratory in advance if anaerobic infection or other unusual organisms or culture requirements are anticipated. Yield on culture can be reduced by prior antibiotic therapy.
Several adjunctive tests exist for the diagnosis of bacterial meningitis.39 Determination of lactic acid levels, in particular, D-lactate, and C-reactive protein may help differentiate bacterial from viral meningitis. Tests for bacterial antigens have proved disappointing as diagnostic tools and have been abandoned by many laboratories. Polymerase chain reaction is a promising tool in the diagnosis of meningitis.39,42 This test, which involves amplification of bacterial DNA sequences from CSF, has far greater sensitivity than do conventional culture methods and is also much less readily affected by prior antibiotic treatment. To date, the major use of polymerase chain reaction has been in the diagnosis of viral meningoencephalitides (e.g., herpes simplex encephalitis, enterovirus meningitis, or polymorphonuclear leukocytes) or of tuberculous meningitis. Several promising reports, however, have demonstrated rapid and successful use of polymerase chain reaction in meningitis due to N. meningitis, S. pneumoniae, β-hemolytic streptococci, and E. coli, including detection of penicillin-resistant strains of S. pneumoniae.42
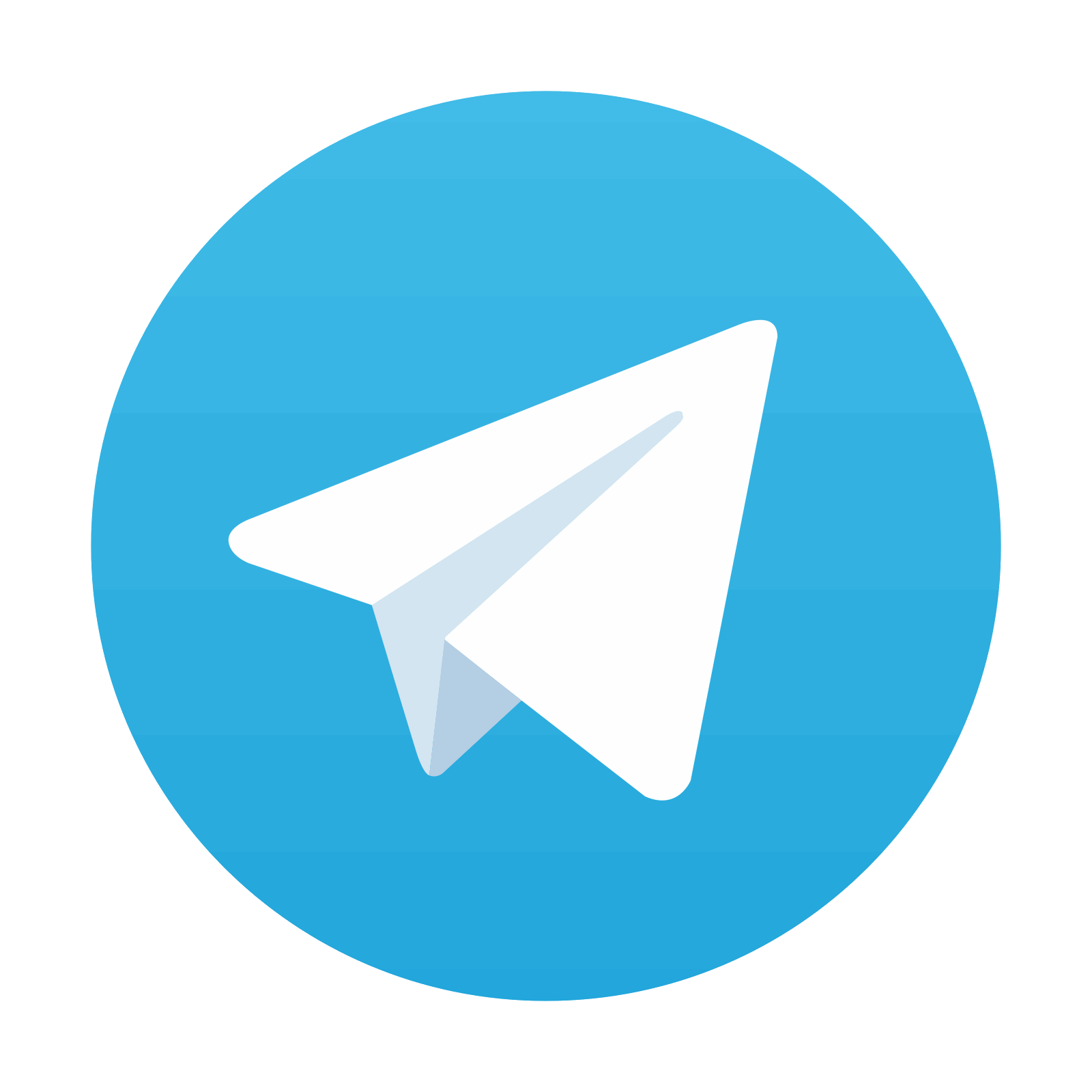
Stay updated, free articles. Join our Telegram channel

Full access? Get Clinical Tree
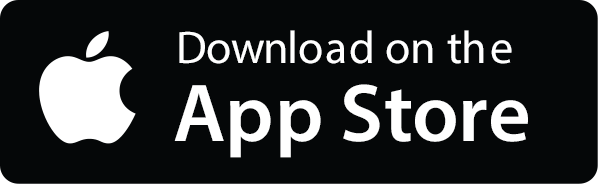
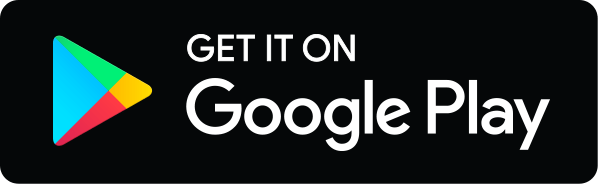