Tissue or fluid
X-ray absorption
Color
Hounsfield units
Air
None
Black
−1000
Fat
Slight
Black
−100 to −50
Water/CSF
Minimal
Black
0
Serum
Minimal
13–38
Unclotted blood
<WM < GM
Dark grey
45–68
White matter (WM)
<GM
20–30
Grey matter (GM)
37–45
Clotted blood
>GM
Light grey
40–90
Bone
White
300–1900
Digital angiography remains an important tool in stroke diagnosis and management, particularly in the imaging of vascular occlusions and direction of intravascular therapy.
For MRI, interpretation of image intensity is more complicated than for CT. The MRI signal is generated for standard proton MRI by hydrogen nuclei (protons) that are predominantly in water molecules, though fat and other biomolecules also contribute to the signal. The signal is proportional to the concentration of protons and the effect of T1 and T2 relaxation times with the MRI pulse sequence. There are also effects of motion and local magnetic field differences. Magnetic field differences may arise from the magnetic susceptibility of surrounding tissue or air and from local variation in the amount of paramagnetic substances, chiefly hemoglobin and its derivatives but also including other substances.
MR image intensities on different pulse sequences for tissues of neurological interest
Pulse sequence | Relative intensity (low to high) |
---|---|
T1-weighted | CSF < GM < WM < fat |
Infarcted brain < non-infarcted brain | |
CSF < GM < WM < or = hyperacute HEM | |
CSF < GM < WM; +edema = acute HEM | |
CSF < Acute HEM = GM < WM | |
CSF < GM < WM < early/late subacute HEM | |
CSF < or = chronic_HEM < GM < WM | |
T2-weighted | WM < GM < CSF |
Infarcted brain > non-infarcted brain | |
Non-edematous brain < edematous brain | |
Hemosiderin + <hemosiderin− | |
Flowing < non-flowing blood | |
WM < GM < CSF < or = hyperacute HEM | |
<WM < GM < CSF < or = acute/early/late subacute HEM | |
FLAIR∗∗ | CSF < white matter/grey matter |
Infarcted > infarcted brain | |
CSF < hyperacute HEM | |
CSF < acute HEM | |
GRE | Hemosiderin < WM < GM < CSF |
4 Acute Stroke
Cerebral blood flow is regulated by a complex mixture of autoregulatory and neurovascular coupling mechanisms mediated by various metabolites and excitatory neurotransmitters including H+, Ca2+, oxygen, adenosine, and excitatory amino acids such as glutamate [13, 14]. These are produced by and act on neurons, astroglia, and blood vessels.
In the first few moments of a stroke decreased blood flow may occur with or without detectable clinical deficits depending on the affected region of the brain. Acute stroke is usually painless, and even acute motor or sensory deficits may not be noticed by the subject because of impaired perception (termed anosagnosia or neglect). Anosagnosia is a feature of cortical lesions in general. Also, stroke in certain brain areas may not be noticed by the patient. The failure of patients to notice the effects of stroke is one reason that patients may not seek medical attention.
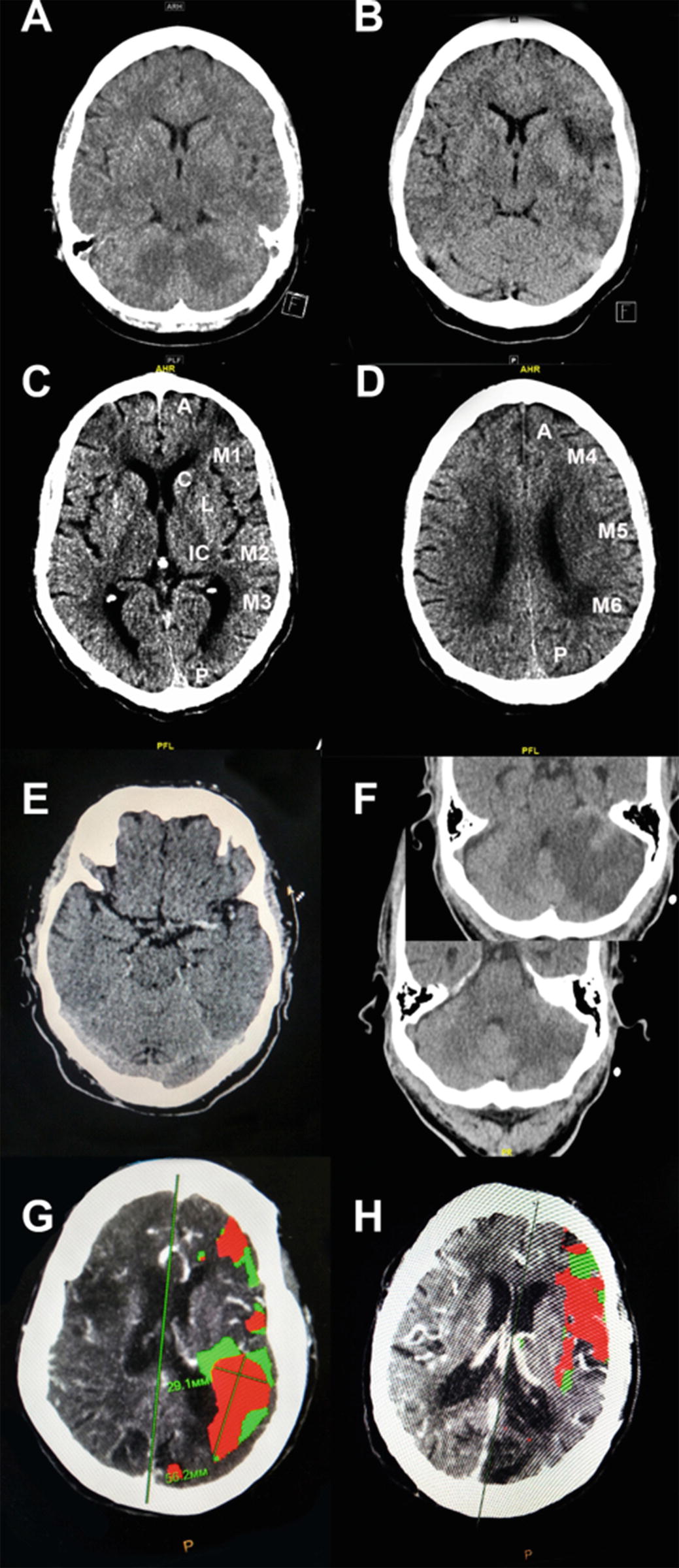
(a) CT of acute stroke with no changes. (b) CT of the same acute stroke hours later with decreased grey–white contrast. (c) The ASPECTS Scoring System applied to non-contrast CT. The affected hemisphere is divided into ten cortical and subcortical regions and one point is deducted for any evidence of acute stroke in each area for a score of 0–10. (d) Another brain slice in the same case with ASPECTS regions defined. (e) An example of the dense MCA sign in a patient with middle cerebral artery distribution stroke. (f) An example of the dense basilar artery sign in a patient with cerebellar stroke. Insert shows another level with large cerebellar infarction. (g) CT perfusion-weighted image showing core infarct (red) and ischemic penumbra (green)
The CT image of acute stroke may contain clues of the underlying pathology including the dense MCA sign correlating with middle cerebral artery occlusion, the insular dot sign [21], or a dense thrombosed basilar artery [22] (Fig. 1g) that may precede or give useful clues to tissue changes. Vessel pathology including calcium emboli, fat emboli, or vascular dissection may also be detected before tissue changes. CT perfusion imaging with contrast is more sensitive and reflects flow differences within minutes (Fig. 1, Panel g, h), though patient handling and other practical matters may delay detection. Rapidly obtained non-contrast CT imaging may be preferable to obtaining an advanced study at a later time [23].
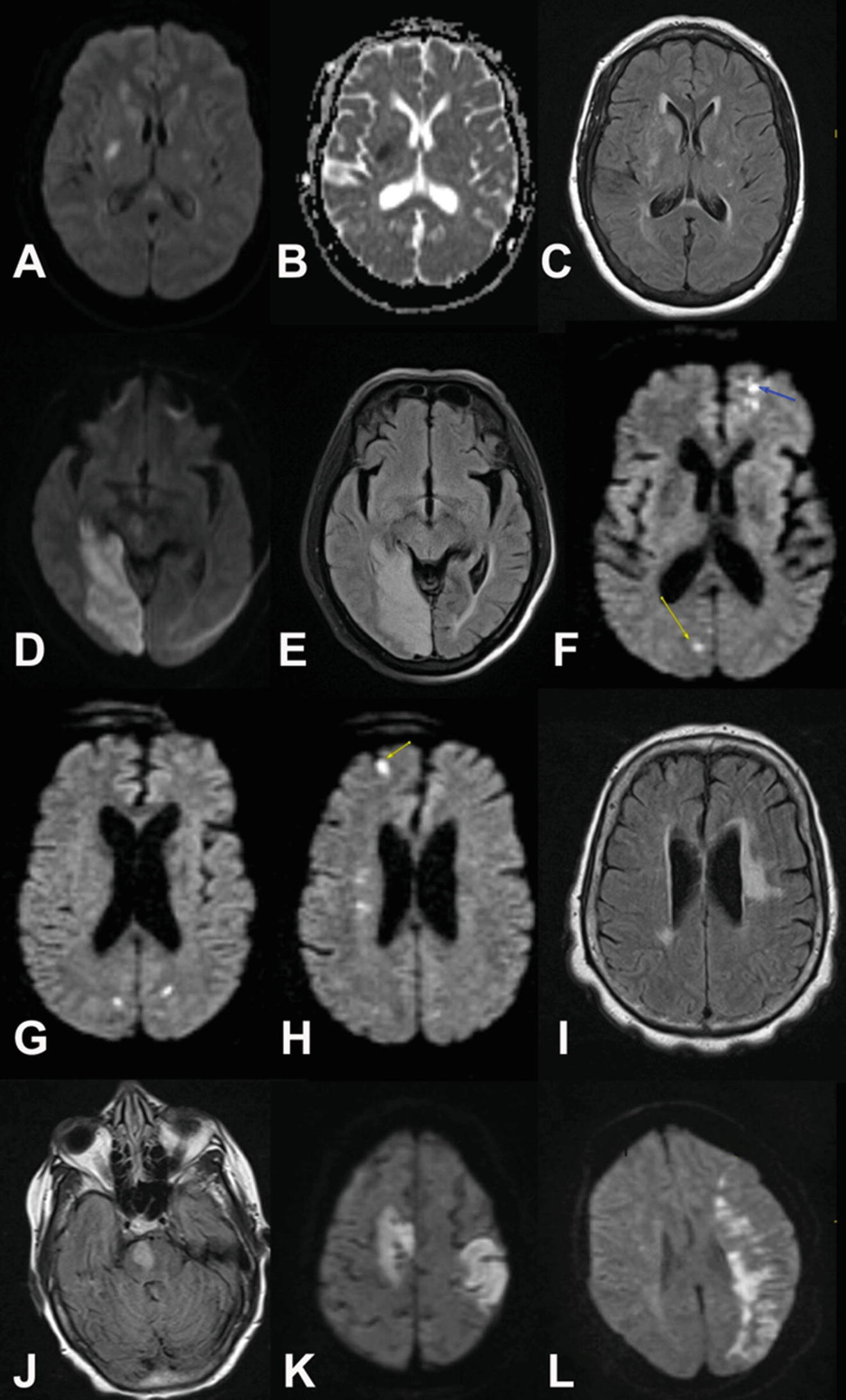
Acute stroke changes detected by MRI. (a) MRI of small acute subcortical basal ganglia region stroke with DWI changes (bright spot). (b) Same region showing correlating ADC dark spot. (c) Same region showing FLAIR image with subtler bright signal. Note that the FLAIR image is much sharper and with more anatomic detail than the ADC image but is not as sensitive to acute stroke. (d) Different case of subacute R occipital stroke showing high signal area in occipital cortex on DWI. (e) Corresponding FLAIR image to (d) showing more anatomic detail and edema with loss of detail in occipital sulci on R compared to L. (f) Another case showing multiple acute cardioembolic strokes in both hemispheres that are bright on DWI. (g) As above additional slices showing additional cardioembolic lesions. (h) As above additional slice showing additional cardioembolic lesions. (i) FLAIR image of same case not showing faint signal from acute emboli but showing large area of probable chronic microvascular change. (j) Patient with basilar thrombosis and acute R pontine infarction on FLAIR image. (k) Patient with multiple cardioembolic strokes with typical peripheral cortical location. (l) Patient with L MCA lesion with internal watershed infarction between deep and peripheral branches of L MCA
Imaging markers for acute ischemic stroke
X-ray computed tomography | Magnetic resonance imaging |
---|---|
Decreased attenuation (darker) | Decreased signal (T1-weighted) |
Increased signal (T2-weighted, diffusion and FLAIRa) | |
Edema (darker) | Edema (brighter) |
5 Embolic and Thrombotic Stroke
Embolic strokes occur when an embolus of blood clot, cholesterol plaque fragment, fat or other non-blood obstruction lodges in a brain blood vessel, blocking flow distally. Most emboli are composed of clotted blood. Emboli originate from the heart, great vessels, carotid or vertebral arteries, or proximal intracranial arteries. An embolic stroke is usually noticed when acute and there may be multiple emboli. Both large and small cerebral vessels may be occluded, and the size and location of the affected vessels will affect treatment options and determine the size of the stroke and reflect the embolic source. The infarcted brain may often have a distal peripheral cortical wedge shape (Fig. 2k), but emboli may occur in any location. Multiple embolic strokes in multiple vascular distributions are typical for cardioembolic stroke. A large embolus may block a large vessel and fragment into smaller more distal emboli within the same distribution.
Typical embolic risk factors include mechanical and bioprosthetic replacement valves, atrial fibrillation, cardiac ventricular thrombi and valvular vegetations, dilated cardiomyopathy, myocardial infarction, congestive heart failure, ventricular hypokinesis, atrial myxoma, marantic endocarditis, infectious endocarditis, atrial septal aneurysm, patent foramen ovale, friable arterial atherosclerotic plaques, and hypercoagulable states (aka thrombophilia).
Thrombotic strokes occur primarily in smaller cerebral vessels and result from pathological changes in blood vessel walls due to atherosclerosis or lipohyalinosis. Thrombotic infarction occurs in areas of preexisting atherosclerotic disease and this is usually at branch points in the cerebral vascular tree or in small penetrating vessels in the brainstem, basal ganglia and deep white matter. The process may be acute or gradual and may, in the case of smaller strokes, be asymptomatic. The inciting event for thrombotic infarction can be disruption of the fibrous cap over a cholesterol plaque, exposing the blood within the vessel to thrombogenic materials and inducing clotting. Lipohyalinosis and fibrinoid necrosis of small cerebral vessels can also be associated with thrombotic stroke [26]. Risk factors for thrombotic strokes include hypertension, hyperlipidemia, diabetes, elevated homocysteine, smoking, cocaine use, oral contraceptives, hypercoagulable or thrombophilia states, inflammatory conditions, and vascular trauma.
The distinction between embolic and thrombotic stroke is of importance for secondary prevention of stroke (prevention of additional strokes following an initial hospitalization for stroke). Embolic strokes typically require anticoagulant (warfarin or direct thrombin inhibitor) therapy, whereas thrombotic strokes require antiplatelet therapy (aspirin or clopidogrel). In the present era of revascularization and clot retrieval therapy, large proximal cerebral infarctions may have a mixture of thrombotic and embolic components and the decision to choose between antiplatelet therapies and anticoagulants is not as immediately relevant.
In the case where images show multiple lesions, DWI and FLAIR images can “time stamp” lesions as acute and differentiate them from chronic lesions (Fig. 2f–i). Thrombotic and embolic strokes may overlap in appearance (Fig. 2j, k). The peripheral (often but not exclusively “wedge” shaped) appearance of embolic strokes (Fig. 2k) may be seen with “external” watershed infarcts (WI—see below) between the anterior and middle cerebral artery distributions and the middle and posterior cerebral artery distributions.
Markers for embolic vs. thrombotic strokea
Embolic | Thrombotic |
---|---|
Wedge shaped and cortical | Smaller and subcortical |
5.1 Watershed Infarctions (WI)
Watershed infarctions occur at the interface between arterial distributions within the brain. These infarctions may present in symmetrical fashion in association with hypotension or in asymmetrical fashion if there is unilateral large vessel occlusion or stenosis. Internal carotid artery stenosis may be associated with asymmetrical watershed infarction. WI are estimated to account for approximately 10% of all cerebral infarctions. The most common feature is an episode of hypotension or low blood flow that preferentially affects the peripheral areas between vascular distributions. In many cases such an episode is suspected but not documented. Thrombotic and embolic factors have also been proposed in the etiology of WI [27]. Either localized patchy or confluent patterns may be encountered, but WI are rarely “solid.” Internal WI may have a “rosary bead” linear appearance.
WI are classified according to the pair of vascular distributions that form the watershed and by whether they are external or cortical versus internal or subcortical. External WI occur between the anterior and middle and anterior and posterior cerebral artery distributions. Internal or subcortical WI occur between the cortical branches of the anterior, middle and posterior cerebral arteries and the much deeper lenticulostriate, Heubner, and anterior choroidal arteries (Fig. 2i, j). Purely hemodynamic factors contribute to all types of WI, but internal WI may preferentially derive from microemboli that fail to clear during hypotension [28].
Markers for watershed stroke
External or cortical | Internal or subcortical |
---|---|
Wedge-shaped between ACAa and MCAb distributions | Linear or “Rosary bead” pattern between deep and superficial MCA distributions |
Wedge-shaped between PCAc and MCA distributions |
5.1.1 TIA
The definition of transient ischemic attack (TIA) is a sudden, focal neurologic deficit of presumed vascular origin that lasts less than 24 h. Deficits that last more than 24 h but resolve by 48 h were called reversible ischemic neurologic deficits (RINDs). It was noted in the 1980s with the development of clinically useful brain MRI that both TIA and RIND were often associated with imaging findings, most commonly focal hyperintense areas on T2-weighted images. With increasing availability of MRI in the 1990s, including innovations such as T2-FLAIR (Fluid Attenuated Inversion Recovery) and DWI (Diffusion Weighted Imaging), finding cerebral ischemic lesions on diffusion weighted imaging in patients with a clinical diagnosis of TIA became increasingly common. Therefore, the concept that no permanent ischemia occurred because the clinical symptoms had resolved had to be discarded.
ABCD2 vs. RRE-90 scales for stroke prediction after TIA
Clinical markers vs. imaging markers | |
---|---|
ABCD2 SCOREa | RRE-90 SCOREb |
Age ≥ 60 years | MRI within 72 h of stroke |
BP > 140/90 | History of TIA within month |
Unilateral weakness | Multiple acute infarcts |
Speech disturbance Without weakness? | Simultaneous Infarcts in different distributions |
Duration ≥ 60 min | Infarcts of different ages? |
Diabetes | Stroke subtype |
Recognizing that finding imaging abnormalities in TIA cases would potentially influence the urgency of diagnostics and treatment, The TIA Working Group was convened in 2000 and released its initial consensus statement in 2002 [39]. After further refinement and discussion, in 2009, the American Heart Association revised the definition for TIA and stroke as follows: “Transient ischemic attack (TIA) is a transient episode of neurological dysfunction caused by focal brain, spinal cord, or retinal ischemia, without acute infarction” [40]. Corollary to this, “An ischemic stroke is defined as an infarction of central nervous system tissue.” In terms of imaging features, a “true TIA” should have no imaging findings. A “false positive” TIA will look like an acute or subacute acute stroke (Fig. 2a).
Guidelines for the management of acute stroke recommend against thrombolytic therapy or revascularization in patients that present with stroke and experience complete resolution of their deficits and have negative imaging before therapy can be started. Such cases would be considered TIA by the 2002 TIA Working Group definition. Patients that have certain persistent imaging abnormalities such as perfusion–diffusion mismatch might benefit from therapy on a case-by-case basis. Patients that improve but have moderate or severe residual clinical disability may benefit from thrombolytic therapy or revascularization [41].
5.1.2 The Time Course of Subacute to Chronic Ischemic Stroke
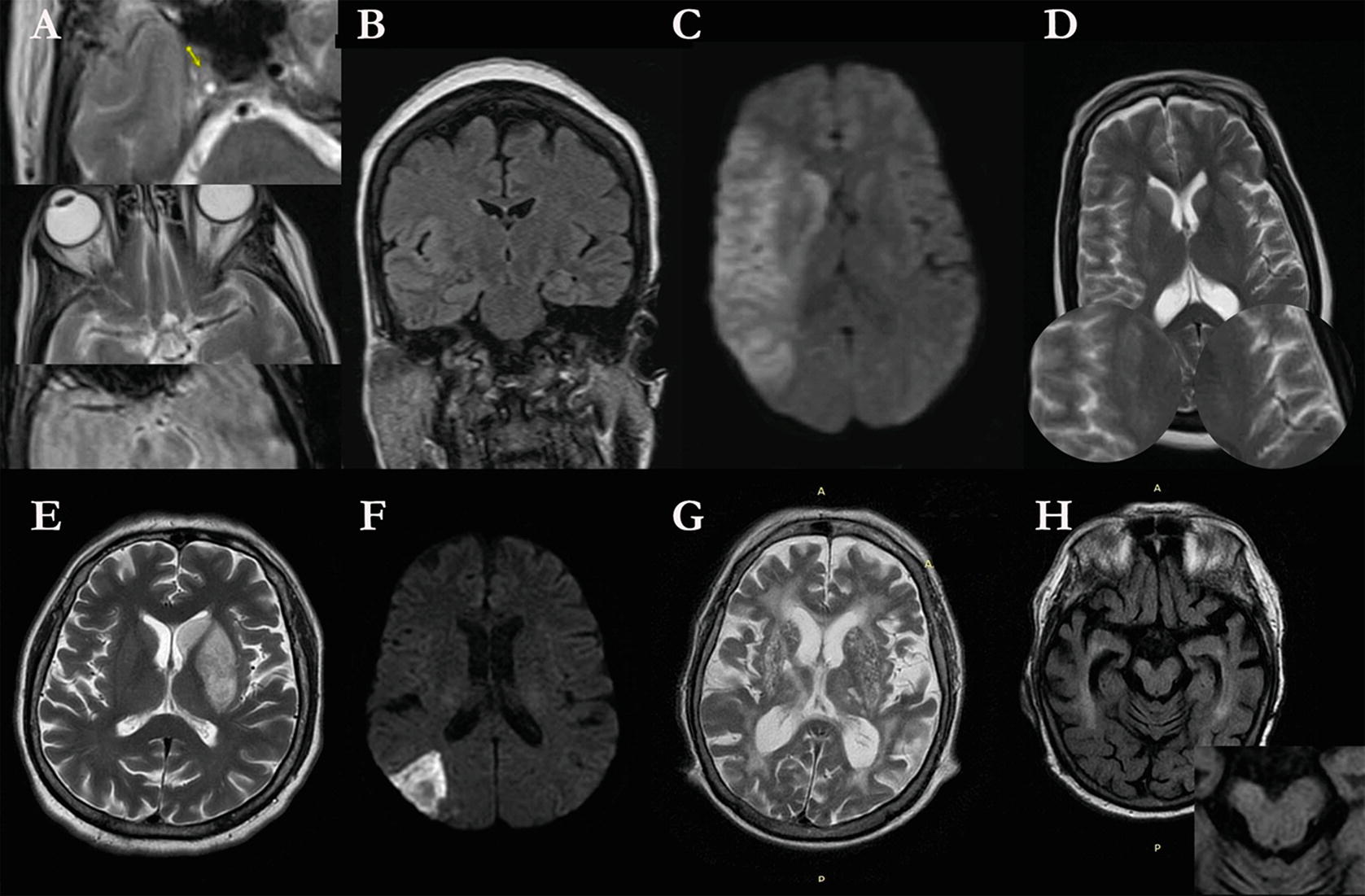
Time course of ischemic stroke. (a) cute Stroke secondary to R internal carotid occlusion (arrow 1) with decreased flow void in R MCA (arrow 2) and susceptibility artifact in R MCA (arrow 3). (b) Edema at site of infarction in R insula compared to L insula. (c) DWI change compatible with core stroke across MCA distribution. (d) T2-weighted image shows lack of flow void in distal R MCA distribution indicating poor collaterals. (e) Maximum edema in Day 3 infarction in L caudate and putamen. (f) Hemorrhagic transformation in embolic stroke on DWI image. (g) Wallerian degeneration in R internal capsule beneath area of encephalomalacia from stroke in R parietal area. (h) Atrophy of R cerebral peduncle beneath area of Wallerian degeneration
Imaging markers for chronic ischemic and chronic hemorrhagic stroke
Feature | Pathology | X-ray CT | MRI |
---|---|---|---|
Core infarct | Atrophy, replaced by CSF | Low attenuation: dark | T1-W/FLAIR: dark T2-W: bright |
Peripheral infarct | atrophy, glial cell scar | +/− Low attenuation: dark | T1-W: dark T2-W/FLAIR: bright |
Connected distant regions | Atrophy, Wallerian degeneration | +/− Low attenuation: dark | T1-W: dark T2-W/FLAIR: bright |
Remaining blood products | Hemosiderin | Isointense | T1-weighted: isointense T2-Weighted/FLAIR/SWI/GRE/DWI: hypointense, “blooming” |
6 Stroke Therapeutic Decision-Making and the Role of Imaging
The 2018 American heart Association (AHA) Guidelines for the early management of patients with acute ischemic stroke [41] reemphasize the “Time is Brain” concept of rapid evaluation and treatment. All patients with suspected acute stroke should have a non-contrast CT on arrival to the emergency room, preferably within 20 min. One major purpose of the rapid CT scan is to detect intracerebral hemorrhage and non-stroke entities such as brain tumors. The decision to admit to the hospital is based on a combination of clinical and imaging factors; even patients with symptoms that resolve (i.e., TIA) may be admitted for further evaluation and observation. Some patients with suspected stroke may turn out to have other non-stroke conditions including psychiatric conversion disorders and “stroke mimics” (see below).
The benefits of thrombolysis (IV alteplase or tenecteplase) and mechanical thrombectomy are highly time dependent so imaging, clinical assessment and alteplase reconstitution and administration must be performed promptly. The initial clinical experience with IV thrombolysis with streptokinase was a disappointment to a large part because patients with “wake-up” strokes were included [44]. Subsequent stroke trials have generally relied on the “last known normal” (LKN) time if the stoke onset is not witnessed and patients that wake up with stroke are assumed to have a time of onset as the time of going to bed as a default.
For IV thrombolysis, exclusion of any acute intracranial hemorrhage (ICH) is essential and remains a contraindication to intravenous thrombolysis. Widespread hypoattenuation (over 30% of the MCA distribution) on non-contrast CT is a relative contraindication to the use of thrombolysis [45]. On the other hand, several other imaging signs were felt to be relative contraindications years ago but post hoc analysis of clinical trial data initially showed that there was no benefit for their use as exclusion criteria when IV-rTPA was the only therapeutic option. These include the appearance of <33% MCA distribution CT hypodense areas associated with acute infarctions, loss of gray/white matter demarcation, Alberta Stroke Program Early Computed Tomography Score (ASPECTS), measures for WMH/leukoaraiosis [16, 17], MRI hyperintensities [46] and the CT hyperdense MCA sign [47]. The situation with microbleeds as detected on T2-weighted, diffusion-weighted or gradient echo MRI scans is more complicated with meta-analyses showing some increased risk [48, 49] particularly in cases with a large number of microbleeds. Patients who meet inclusion criteria for TPA but who have large strokes would benefit from TPA, but hemorrhage risk would be greater [50]. It should be noted that the therapy decision process has been evolving with the availability of intravascular therapy options.
6.1 The Role of Intra-arterial Thrombolysis
Current guidelines for management of acute stroke continue to emphasize rapid evaluation of patients for intravenous IV alteplase treatment, emphasizing telestroke consultation where necessary and transfer to facilities where endovascular therapy for stroke is available [41]. Intra-arterial alteplase use played a limited role in endovascular trials but is currently used as a rescue therapy when mechanical endovascular treatments do not achieve enough reperfusion.
6.1.1 Patient Selection for Endovascular Therapy
Current interventional stroke therapy beyond intravenous or intraarterial TPA is based on the 2015 updated AHA/ASA (American Heart Association/American Stroke Association) guidelines for the early management of patients with acute ischemic stroke. A central component is rapidly identifying patients with (1) large vessel occlusions accessible to currently available stent retrievers and who have (2) significant areas of therapeutically reversible ischemia, that is, ischemic penumbra or diffusion–perfusion mismatch. Such patients are the best candidates for revascularization/clot extraction.
The early attempts with intravascular revascularization therapy were successful in improving blood flow through larger arteries but clinical results were disappointing in part because of the performance of earlier devices [5] but also because of including patients that might benefit less because of large areas of unsalvageable “core infarct” or small areas of salvageable “ischemic penumbra [23].
There was accumulated evidence for improved outcome in stroke patients with greater amounts of pial-derived collateral blood supply who underwent intravenous and intra-arterial thrombolysis [51, 52], presumably because pial collaterals could extend viability beyond the 4.5 h window of intravenous therapy. Measures of collateral flow could be used to select appropriate patients.
These observations resurrect the concepts of core stroke and ischemic penumbra. Early non-imaging studies in animals established the ischemic threshold for core stroke as the reduced level of cerebral blood flow (CBF, usually expressed as ml blood flow per minute per 100 g of brain) below which brain tissue is not viable under the conditions of the experimental system. Above this there is a second threshold for ischemic penumbra where the brain is functionally impaired (perhaps with loss of measurable EEG activity) and will become necrotic with prolonged ischemia but it will resume normal function if blood flow is raised. Outside this is a region of benign oligemia where neurological function is impaired, but function will recover without treatment. Early studies established a core infarct ischemic threshold as <10 ml/min/100 g brain and an ischemic penumbra threshold in the range of 16–18 ml/min/100 g brain with normal flow approximately 50–54 ml/min/100 g [53]. Thresholds vary between different experimental brain models [54].
6.1.2 Clinical Scales of Suitability and Success in Intravascular Treatment
Grading of intravascular occlusion
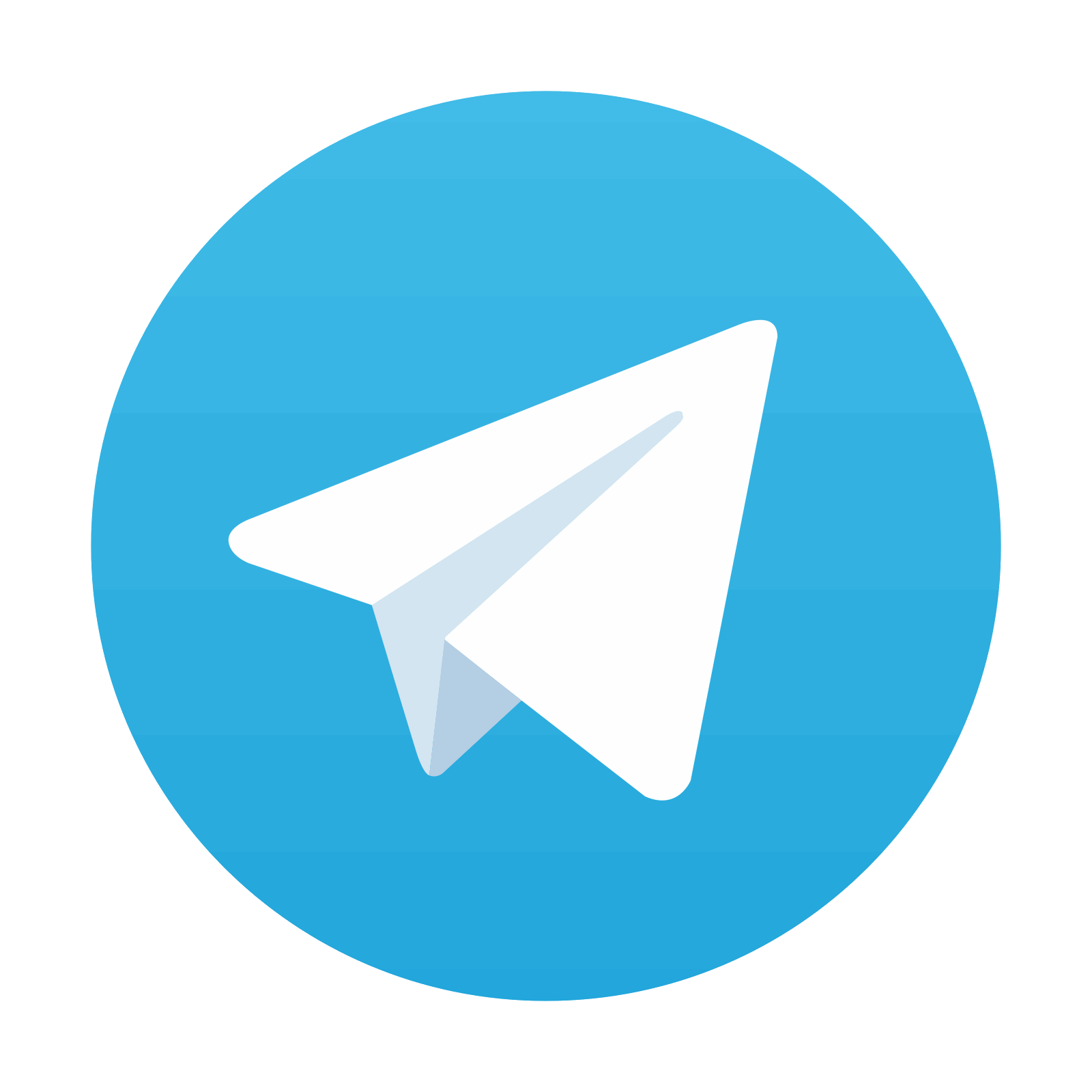
Stay updated, free articles. Join our Telegram channel

Full access? Get Clinical Tree
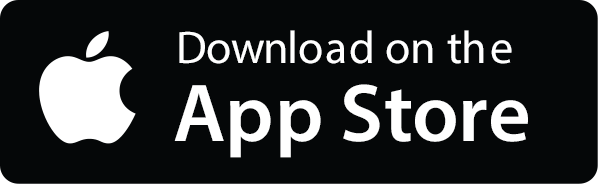
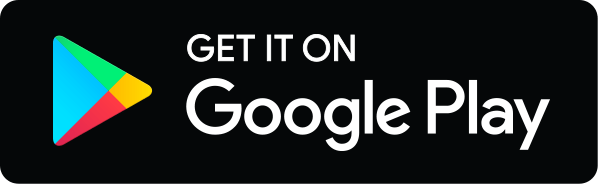