Craniovertebral junction (CVJ) is the region around the skull base and the upper cervical spine (atlas and axis), along with its neurovascular components, including the brain stem, spinal cord, vertebral artery (VA), and venous plexus. 1,2,3 The stability of CVJ is dependent on a strong ligamentous complex and the shape of the bony structures, which are also responsible for much of the axial rotation (C1–C2 joint) and flexion–extension movements (C0–C1 and C1–C2 joint). 4,5,6
CVJ deformity has various etiologies, such as congenital anomaly, tumor, infection, rheumatoid arthritis, and trauma. 7 One of the most common CVJ anomalies is basilar invagination (BI), either congenital or degenerative, as represented by the upward migration of the odontoid process into an already limited space of the foramen magnum causing compressive myelopathy around the cervicomedullary junction. 8,9 Many bony anomalies are associated with BI, such as clivus and condyle hypoplasia, occipital assimilation, os odontoideum, bifid C1 arch, and Klippel–Feil syndrome. 10,11 It is widely believed that the hypoplastic effect of normal bony structures results in BI, as seen in achondroplasia, clival hypoplasia, atlas hypoplasia, occipital condyle hypoplasia, or atlantooccipital assimilation. 12
Rheumatoid arthritis is the leading cause of secondary BI, which is also called basilar impression, atlantoaxial impaction, vertical settling, and cranial settling. 13 Symmetrical rheumatoid destruction of the C0–C1 and C1–C2 joints allows for the cranium to settle on the cervical spine and for the dens to enter the foramen magnum. However, an involvement of only one lateral mass may result in a fixed rotational tilt of the head. Secondary BI often results in a reduction of atlantodental interval (ADI) due to the conical shape of dens and reduced motion, producing a false impression of anatomic improvement known as “pseudostabilization.” 14
CVJ deformity results in sagittal and coronal imbalances, which causes significant pain due to arthritis, instability, and C2 foraminal stenosis. Moreover, malalignment can be a potentially life-threatening instability or cervicomedullary compressive myelopathy.
Patients presenting with intractable pain related to CVJ instability or myelopathy caused by the CVJ deformity usually require surgical treatment. Decompression of neural structures, sagittal and coronal spinal realignment, and stabilization are the primary goals of surgical intervention.
21.2 Craniovertebral Junction Alignment
During fetal development, the spine assumes the shape of the letter “C”, with the curved side facing toward the back, while the open side faces the front of the fetus. This C-shaped curve is the primary curve of the spine and is well suited to the cramped confines of the womb. Newborn infants retain this primary curve and, with growth and maturity, develop secondary curves, first in the neck and later in the lower back. Of the two secondary spinal curves, the cervical curve in the neck region develops first. Within the first few months of life, with sufficient development of strength and coordination to bear his or her own head, the cervical lordotic curvature begins to develop. This curve develops in the opposite direction from the primary spinal curvature. This important milestone allows the infant to have visual access to his surroundings. The enhanced visual perspective contributes to additional developments, such as reaching, grasping objects, and intentional crawling. Although there are so many factors affecting the normal cervical curvature, the aforementioned reason is why most people have lordotic cervical spine curvature. 15,16
This secondary cervical curvature is also found in quadrupedal animals as well as in humans. However, there is a significant difference between humans and animals with respect to this curvature: the upper cervical alignment compared to the cranium. It is because the location of the human foramen magnum is quite different from any other kind of animal.
In humans, the foramen magnum is anteriorly positioned, with its anterior portion lying on the bitympanic line (a line that connects the inferolateral points of the right and left tympanic plates) and is inferiorly oriented (opening directly downward). The occipital condyle that lies anterior to the foramen magnum (the basioccipital or basiocciput) is relatively short in humans. Contrastingly, in apes, the foramen magnum lies well behind the bitympanic line, posterior to a relatively long basiocciput. In addition to being more posteriorly positioned, the foramen magnum in apes is more vertically oriented (opening backward and downward, rather than directly downward). 17,18
Most researchers explained the anterior position of the human foramen magnum as an adaptation to maintain balance of the head atop the cervical vertebral column during bipedalism. 18,19,20,21
Hence, the human head is naturally in equilibrium upon the vertebral column in a standing position, without any strenuous effort. However, quadrupeds with posteriorly positioned foramina magna require well-developed nuchal musculature and ligaments to bear the weight of the head to maintain horizontal gaze. Therefore, the demands on the neck musculature differ between humans and quadrupedal mammals. Volume of the rectus capitis muscle is also quite different between humans and quadrupeds. In quadrupeds, the surface area of C1 posterior arch is quite broad for the rectus capitis muscle attachment, and V3 segment of VA is covered by the broad bony roof (▶ Fig. 21.1a), but in humans, the C1 arch is narrow and VA is not covered by the bony structure above the C1 arch in the general human population (▶ Fig. 21.1b). Posterior ponticulus is the bony bridge formed between the posterior portion of the superior articular process and the posterolateral portion of the C1 posterior arch (▶ Fig. 21.1b), which mimics the broad C1 arch of quadrupeds. 22,23,24 Its prevalence has been reported to be between 5.14 and 37.83%. 25,26,27,28,29,30 It could be a vestigial structure as humans have evolved, as upper cervical spine locates down below the head and strenuous rectus muscles become unnecessary to hold up the head.
Positional difference of the foramen magnum causes a difference in CVJ alignment between humans and other quadrupeds with respect to the C0–C1 segment.
When humans are at rest, the C0–C1 articulation is held rather flexibly, whereas the C1–C2 joints are held slightly in an extended position. This is because the human cervical spine is located inferior to the foramen magnum, and these characteristics ensure an energy-saving balance of the head and neck when at rest, allowing humans to maintain horizontal vision effectively without significant effort of the neck extensor muscle.
Radiographically, the C1 slope is backwardly slanted and kyphotic angulation of the C0–C1 segment allows some degree of freedom for neck extension as the space between the occiput and C1 posterior arch allows for upper cervical extension (▶ Fig. 21.2a).
When patients have kyphotic deformity in the lower cervical spine or thoracolumbar spine, C0–C1 segment can hold up the head to compensate for distal kyphosis, maintaining the sagittal balance and horizontal gaze (▶ Fig. 21.2b, c). Therefore, a normal kyphotic angulation of C0–C1 segment could be referred to as a “tertiary curvature” to characterize human CVJ alignment, to differentiate between upper cervical spine alignment and primary/secondary curvature of the human spine, as well as to differentiate between the cervical spine curvature of humans and that of quadrupeds.
Although asymptomatic population may have different types of cervical alignment, such as “straight” or “kyphotic” cervical spines ranging from 7 to 40%, it has been well accepted that cervical lordosis is a physiological posture in asymptomatic subjects, and a large percentage (about 75–80%) of cervical lordosis is localized to the C1–C2 segment. Only a small percentage (15%) of cervical lordosis exists in the lower cervical levels. On average, the C0–C1 segment is kyphotic. 31,32,33,34,35,36 The loss of subaxial lordosis has been reported in CVJ fixation in which excessive hyperlordosis is created at the C0–C2 segment. 37 Moreover, craniometric studies revealed that an excessive CVJ kyphosis can cause subaxial compensatory lordosis (▶ Fig. 21.2d). 38,39,40 The anatomy of the cervicothoracic junction (CTJ) has been shown to be the main determining factor for cervical lordosis. However, the angle of CVJ has also been shown to be significantly related to the angle of subaxial cervical spine. These findings suggest that reciprocal interaction may likely affect not only global balance but also regional balance. 41
Fig. 21.1 (a) Posterior view of dried quadruped’s specimen shows the craniovertebral junction and broad C1 posterior arch (arrow). (b) 3D reconstruction CTA shows normal C1 arch (black arrow) on the right side and posterior ponticulus (white arrow) covering the V3 segment of VA on the left side.
Fig. 21.2 Lateral X-ray shows normal alignment of the cervical spine. In most occasions, cervical lordosis occurs at the C1–C2 segment. C0–C1 segment is kyphotic and C1 slope is posteriorly slanted (a). C1 slope is reversely slanted and C0–C1 segment is hyperlordotic to maintain horizontal gaze in subaxial cervical kyphotic deformity patient (b) and ankylosing spondylitis patient (c). Lateral X-ray of CVJ deformity patient shows a kyphotic CVJ alignment and hyperlordotic compensation in the C0–C1 segment (reversed C1 slope) and subaxial cervical spine (d).
Another interesting finding is that neck flexion and extension movement are initiated primarily by motions between the head and C1. 42 As the head approaches full flexion and extension, contribution from the upper cervical segments decreases, whereas contribution from the lower cervical segments increases. Understanding the normal CVJ alignment and segmental motion is paramount to better understand CVJ deformity and to decide on a more appropriate surgical strategy according to the pathologies of CVJ.
21.3 Clinical Symptom and Sign
There are various presentations for CVJ deformity, with many of them being a direct result of compression, and it may be associated with Chiari malformation. The degree of upward migration of the dens determines the neurologic sequelae, as this creates significant crowding at the foramen magnum and at the medulla oblongata. Moreover, this crowding could obstruct cerebrospinal fluid (CSF) flow leading to syringomyelia. In the cases with cervicomedullary dysfunction, patients may have ataxia, dysmetria, nystagmus, dysphagia, or cranial nerve palsies, and report weakness, loss of endurance, loss of dexterity, gait disturbance, and paresthesia. 43,44
Neck pain is the most common complaint of CVJ deformity. It is usually associated with occipital headache. Occipital neuralgia, facial pain, and periauricular pain can occur from compression of the greater occipital nerve (C2 root), nucleus of the spinal trigeminal tract, and greater auricular nerve, respectively. Exertional cough headache is a well-documented symptom in cases with syringomyelia. 44
Difficulty swallowing may be associated with CVJ deformity, especially in cases of CVJ kyphosis. 45,46 In cases with severe-degree CVJ kyphosis, dysphagia may be inevitable, not only because the oropharyngeal space becomes narrow but also because the angle change and movement of C0–C1 segment are not possible to compensate for the mechanical restriction in fixed kyphosis. 46,47 Hence, it is important to correct CVJ kyphosis to relieve dysphagia. In addition, sparing C0–C1 segment could provide a certain degree of freedom as a compensatory movement and angle change to avoid dysphagia when treating CVJ pathologies if C0–C1 segment is not the primary pathology for treatment.
21.4 Preoperative Radiologic Assessment
Irreducibility was defined as a nonalignment of C1–C2 after neck extension (determined on lateral X-ray) or after cervical traction. Lateral radiography with dynamic study is necessary to evaluate ADI, degree of vertical subluxation, and reducibility. Furthermore, bony abnormalities, such as atlas occipitalization, os odontoideum, bifid C1 arch, and C2–C3 fusion can be evaluated on lateral radiography.
Traction can be a useful tool in evaluating the reducibility and predicting intraoperative neurological worsening by surgical position. Preoperative cervical traction may correct the atlantoaxial dislocation (AAD) and vertical subluxation in some reducible deformity cases. This could especially be important for the management of fragile patients because they can be treated with only a stabilization procedure postreduction, reducing the level of fixation. Gardner cervical traction was applied, depending on age and weight, starting with 2 to 5 kg for 1 or 2 days. The head of the bed was elevated to provide a countertraction. Serial radiographs were assessed for reduction. These patients who demonstrated a reduction were classified as reducible AAD or BI.
MRI is critical to evaluate cord compression, T2 signal change, Chiari malformation, and syringomyelia. CT scans can confirm bony abnormalities, exact location of the dens, C1–C2 joint destruction, and abnormal C1–C2 facet angle. CT scans are also used to determine the extent of facet fusion and osteophytic bridging to assess the need for C1–C2 osteotomy.
CTA screening is a useful tool for getting a three-dimensional information of complex deformity and for identifying VA anomaly around CVJ. 26,48 The incidence of V3 segment anomalies has been reported to be as high as 10% ( ▶ Fig. 21.3). 26,49 In V3 segment anomalies, since the VA or its major branch courses inferiorly to the C1 arch, these anomalies pose a surgical challenge. These anomalous vessels may likely be injured during drilling, tapping, and insertion of the lateral mass screws. Therefore, when a V3 segment anomaly is detected, a more optimal entry point for C1 fixation should be selected to avoid significant morbidities associated with VA injury. In these cases, five alternative techniques can be utilized to avoid V3 segment injury. The first technique is to choose the superior lateral mass as an alternative starting point for C1 posterior screw placement. It could be useful especially in cases of persistent first intersegmental artery, which is the most common anomalous V3 segment. The second is to choose the C1 dorsal arch as the entry point for C1 screw placement. The C1 dorsal arch might be the best entry point in cases with fenestrated VA or posterior inferior cerebellar artery early branch. The third method involves C1–C2 transarticular screw fixation to avoid causing an injury to the abnormal VA below the C1 arch. The fourth technique is to skip the C1 screw fixation and extend the level of fixation proximally or distally. The last technique is to mobilize the VA inferiorly together with the C2 nerve root before screw placement at the C1 inferior lateral mass. Although there could be a racial difference in the incidence of V3 segment anomaly, existing literature indicates that V3 segment anomaly is more common in the group with congenital bony anomaly. Therefore, we suggest that preoperative CTA might be informative for deciding on a surgical technique, especially in high-risk group of the V3 segment anomaly, such as Asians and those with CVJ deformity with congenital bony anomaly. 49
Fig. 21.3 3D CTA images show three different types of V3 segment anomaly. (a) 3D CTA demonstrates the persistent intersegmental artery on the left side (white arrow), the VA course abnormally below the C1 arch after leaving the transverse foramen of the C2 and enter the spinal canal not passing through the C1 transverse foramen. Right side V3 segment (black arrow) is normal. (b) 3D CTA demonstrates the main V3 segment was normal, but the right side posterior inferior cerebellar artery (white arrow) originated from the VA between C1 and C2. (c) 3D CTA shows the fenestrated VA on the left side; one courses as usual, while the other entered spinal canal below the C1 arch and joins the former above the C1 arch.
21.5 Treatment of Craniovertebral Junction Deformity
A surgical treatment of CVJ abnormalities was first proposed in 1977. 50 It was further advanced by the introduction of posterior segmental fixation. Factors that influence specific treatment of CVJ deformity are as follows: (1) the reducibility of deformity (i.e., restoring anatomic alignment, thereby relieving neural compression); (2) the direction of mechanical compression; (3) the presence of abnormal craniocervical angle and alignment; and (4) the presence of hindbrain herniation, syrinx, and vascular abnormalities.
The primary goal of treatment is to relieve compression at the cervicomedullary junction. Stabilization is critical to treat reducible deformity and to maintain neural decompression. Irreducible lesions may require decompression at the site of compression or may require an osteotomy procedure to make the lesion reducible and relieve neural compression. 41
Irreducible lesions can be divided into two types: the ventral compressive lesion and the dorsal compressive lesion. Dorsal compression cases need either a posterior or posterolateral decompression procedure, and if there is instability after decompression, posterior fixation is mandatory to gain stability.
Transoral surgery is a procedure carried out through the oral cavity to gain access to ventral compressive lesion of CVJ. Under normal conditions, surgeons should be able to expose the area from the lower rim of clivus cranially to the level of C23 disc space caudally. Transoral surgery is often necessary in case of irreducible CVJ deformity, where ventral compression to neutral structures is present. 51 Other indications for transoral surgery include CVJ tumor and infection, in which the anterior compression cannot be decompressed by a simple posterior reduction. 52 Although the combined anterior-posterior approach may provide satisfactory neural decompression and fusion, it poses several disadvantages, such as higher risk of surgical morbidities, prolonged postoperative intubation/nasogastric feeding, phonation difficulty, and potential infection. 53,54,55 The risk of infection is a big concern particularly in cases where the subarachnoid space had to be opened, which may cause catastrophic sequelae. Another issue is that transoral decompression can induce significant instability of CVJ, inevitably requiring a subsequent posterior stabilization procedure. These issues are reasons why the initial enthusiasm vanished and indication of transoral decompression has become less clear in recent literature.
A C1–C2 joint distraction technique, as introduced by Goel, has been gaining popularity recently as a possible treatment modality for selected cases of AAD and BI. 56,57,58 This technique seems to have several advantages over conventional transoral surgery and occipitocervical (OC) fixation. First, direct reduction and fixation are possible posteriorly for cervicomedullary decompression. Hence, a transoral surgery and its related complications could be avoided. Second, the dimension of the fusion bed could substantially be increased because C1–C2 facet surface is rather large. Third, it can avoid occipital fixation and conserve C0–C1 segmental motion. Fourth, C1–C2 joint manipulation can make a fixed subluxation or deformity reducible, minimizing the need for a head traction, before and during surgery.
However, despite these advantages, some concerns have also been expressed in the literature regarding this joint distraction technique. First, there is a debate regarding the C2 neuropathic pain after C2 root resection. Although C2 root resection is not mandatory for C1–C2 facet opening, it is useful to make a wide exposure for the C1–C2 facet surface, especially when C1–C2 interlaminar space is narrow and venous plexus is prominent. 58,59 Thus, C2 root resection is one of the key parts of the C1–C2 joint distraction procedure. In the literature, most authors have reported that the incidence of C2 neuropathic pain is quite low after C2 root resection. 58,59 However, C2 neuropathic pain could be troublesome for some patients. Therefore, surgeons should be aware of both the benefits and risks associated with C2 root resection. Second, we do not fully know the adequate amount of distraction required for the best neurological recovery and prevention of neurological complications related to the overstretching of the spinal cord and VA. Moreover, the long-term fate of adjacent C0–C1 segment has rarely been reported in patients after receiving C1–C2 vertical distraction procedure with respect to the segmental motion and adjacent segmental degeneration. Yoshida et al reported that C1–C2 rigid fixation can provide prophylactic benefits against vertical subluxation and subaxial subluxation in RA patients. 60 Werle et al reported that C0–C1 degeneration is very low after C1–C2 fusion in RA patients. 61 Therefore, C0–C1 segment sparing monosegmental C1–C2 fusion should be the preferred treatment of choice, especially for RA patients.
21.5.1 Preoperative Planning
▶ Fig. 21.4 provides decision-making pathways to treat CVJ deformity.
Fig. 21.4 Algorithm shows decision-making pathways for the treatment of the CVJ deformity.
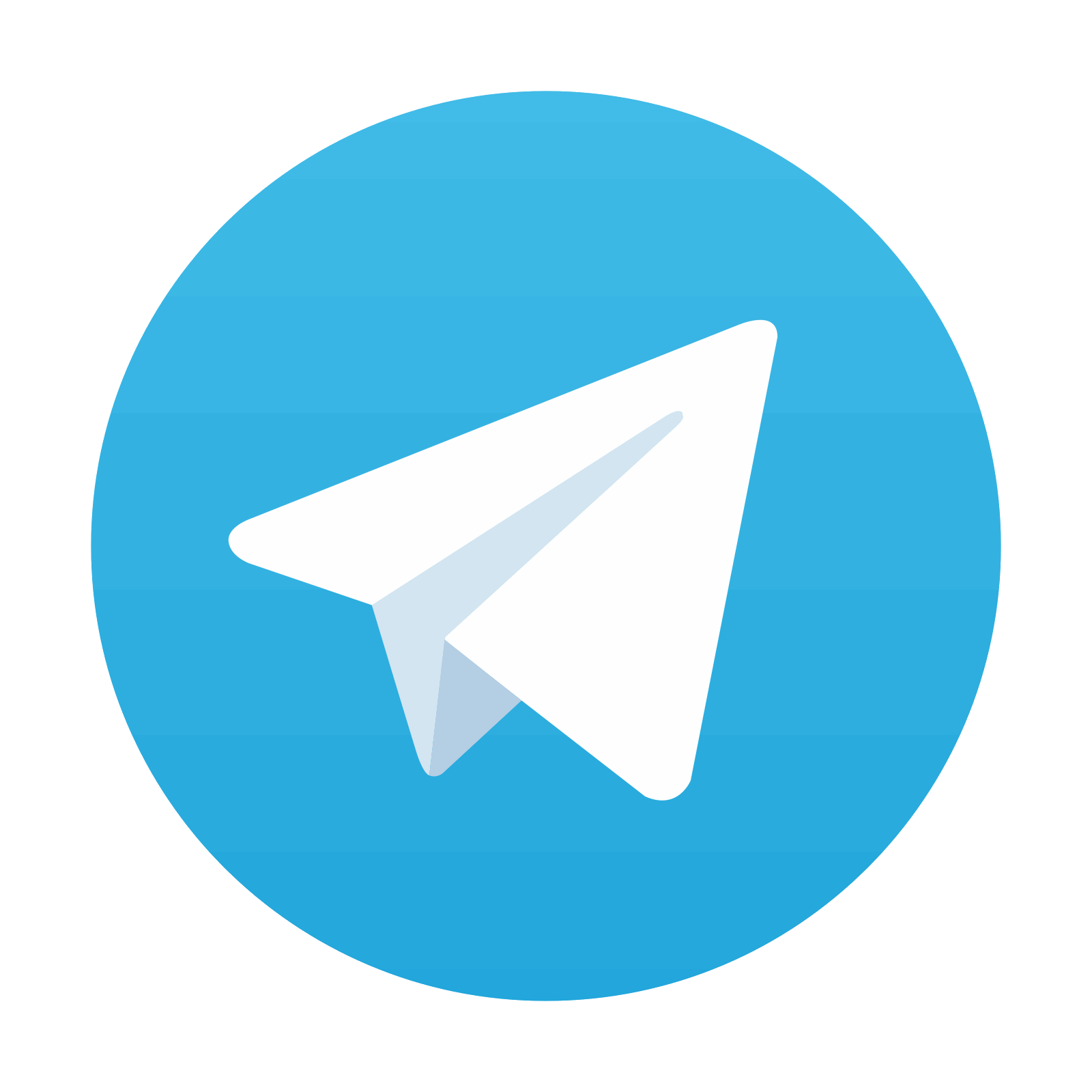
Stay updated, free articles. Join our Telegram channel

Full access? Get Clinical Tree
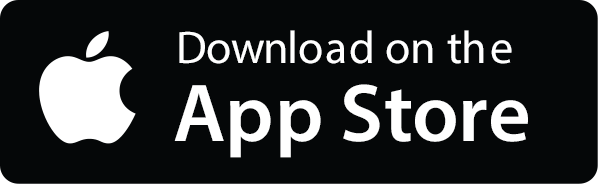
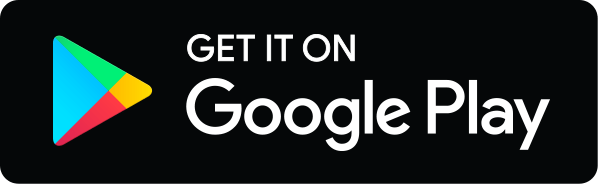