Caring for the Pediatric Neurosurgical Patient The care of pediatric neurosurgical patients is a broad and challenging topic. This chapter deals principally with general guidelines for the management of these children, from measures to reduce infection to pain control regimens to efforts to limit postoperative complications. Specific topics are addressed in considerably more detail in individual chapters. Patients at Children’s Hospital of Pittsburgh are requested to use a chlorhexidine gluconate shampoo and body wash (4% w/v chlorhexidine gluconate; Hibiclens) for five consecutive nights leading up to the surgical date for all elective procedures. Although the Cochrane Database guidelines do not recommend preoperative body washing to reduce surgical site infections (SSIs;commonly used abbreviations are listed in ▶ Table 4.1), those guidelines are based on data from 20 to 30 years ago.1 Multiple studies with sample sizes too small to achieve statistical significance have found lower numbers of colony-forming units (CFUs) on the incisions, on the intraoperative cultures, and on the skin of patients who showered preoperatively with chlorhexidine gluconate.2–5 Multiple days of showering have been recommended, with the most important day being the day before surgery.6,7 The preoperative skin preparation should be the same preparation used in the operating room (OR) immediately before surgery, as the effect of exposure to a skin preparatory product is cumulative.8 Hair clipping should be minimal and should be performed just before skin preparation in the OR. Clipping hair in the days before surgery may lead to an increased incidence of infection, although data have not been statistically significant.9,10 Shaving should not be performed, as infection rates are clearly higher.9,11,12 The avoidance of hair clipping is associated with a slightly lower rate of postoperative infection, and some have suggested that no hair removal be performed.13–15 Skin preparation before incision is not performed in a uniform fashion around the country. Chlorhexidine gluconate (2% chlorhexidine gluconate, 70% isopropyl alcohol) achieves greater reduction of skin microflora than does povidone–iodine and has greater residual activity after a single application.2,16,17 Ethanol produces faster bactericidal activity, and at high concentrations it is the most effective product against viruses. However, its flammability makes it a less desirable option in the OR.18 Preoperative skin antisepsis with chlorhexidine–alcohol was shown to be superior to povidone–iodine in reducing SSIs, both superficial and deep.19 Chlorhexidine acts by disrupting cell membranes and carries a risk for ototoxicity. Povidone–iodine acts by oxidizing amino acids and disrupting cell membranes. Because iodine can produce hypothyroidism in low-birth-weight infants, its use should be limited accordingly.20,21 Alcohol acts by denaturing proteins. Iodine has the lowest risk for complications, and alcohol has the shortest duration of action. Alcohol-based solutions should be avoided in neonates before of the possibility of burns.22 Chlorhexidine gluconate is recommended for use in children past the age of 2 months by the manufacturer for this reason. For elective neurosurgical patients, anticoagulation should nearly always be discontinued before surgery. Aspirin can be administered no more than 7 days before surgery without an adverse effect on coagulation, although aspirin may be intentionally continued through surgery in selected patients (e.g., those with moyamoya). Nonsteroidal anti-inflammatory drugs (ibuprofen, naproxen; medication dosages are listed in ▶ Table 4.2) should not be administered during the 24 hours before surgery. Patients on warfarin should be transitioned to heparin in the hospital with the consultation of the hematologist, or to low-molecular-weight heparin (enoxaparin [Lovenox] or dalteparin [Fragmin]) 3 days before the procedure. Low-molecular-weight heparin should be discontinued 24 hours before the procedure, and heparin 6 hours before the procedure. Clopidogrel (Plavix) must be stopped 5 to 7 days before surgery. The reason that the patient is taking the anticoagulant must be weighed against the risk for intraoperative and postoperative bleeding. Antibiotics are administered within 1 hour of surgery for every case, with the possible exception of cases in which identification of a microorganism is desired. The usual choice of antibiotic is cefazolin, although clindamycin and vancomycin are second-line substitutes for those with a cephalosporin allergy. Antibiotic prophylaxis has been shown to be generally effective in reducing the risk for wound infection in a wide variety of adult cases.23 Prophylactic antibiotic therapy has been shown to be effective for craniotomy and spinal surgery in adults, although no role for prophylaxis against gram-negative organisms or multiple doses of antibiotics could be proven effective.24,25 Prophylactic antibiotics reduce the risk for meningitis by approximately 50% in adult patients undergoing craniotomy.26 A single dose has been shown to be adequate for surgical wound prophylaxis in many cases, including gastrointestinal surgery cases.27 Repeat dosing while the patient remains in the OR is recommended to maintain adequate serum levels during surgery.28 Studies have demonstrated the efficacy of intraoperative dosing of antibiotics in reducing postoperative incisional infections, although not postoperative meningitis.29,30 Additional dosing beyond the termination of surgery is not generally recommended.28 However, the use of 24 hours of prophylactic antibiotics in patients undergoing implantation of shunts was found in a meta-analysis to reduce the rate of shunt infection.31 Dosing for more than 24 hours postoperatively in any case is not recommended.28 The use of local anesthetic has several advantages. Long-lasting anesthetics like bupivacaine allow improved postoperative pain control, with a reduction in the need for opioid administration. The addition of epinephrine to the local anesthetic prolongs the duration of the anesthetic effect; it also produces vasoconstriction, thereby reducing blood loss during skin opening. This is especially desirable in neonates, in whom little blood loss is tolerated. The use of local anesthetic must be meticulously avoided in patients undergoing extracranial–intracranial bypass, to avoid both vasoconstriction and inadvertent injury to the donor vessel. Hand washing by surgical personnel is a variable process among institutions and individual surgeons. Chlorhexidine gluconate is clearly superior to povidone–iodine in terms of reducing the number of CFUs that surgical personnel have on their hands after performance of routine preoperative hand antisepsis. No effect of the length of time a scrub is performed has been demonstrated, although 2 minutes appears to be the minimum necessary.32 Hand rubbing with an aqueous alcohol-based solution, preceded by a 1-minute nonantiseptic hand wash before the surgeon’s first case of the day or when hands are overtly soiled, is as effective as traditional hand scrubbing with antiseptic soap at preventing SSIs.33 The use of an alcohol-based chlorhexidine surgical rub (1% chlorhexidine gluconate and 61% ethyl alcohol w/w [Avagard; 3M, St. Paul, MN]) instead of a traditional aqueous chlorhexidine scrub may be more effective in reducing the bacterial flora on the hands in both an immediate and a delayed fashion.34 Multiple studies demonstrating a reduced number of CFUs on the hands with the use of chlorhexidine have been performed, but none has linked the use of any preoperative hand scrub or surgical site preparatory solution to a decreased incidence of SSIs. Double gloving has been resisted by many surgeons because of reduced tactile sensation and increased cost. Nonetheless, bacterial migration through punctures in surgical gloves has been shown to occur.35,36 Double gloving has been shown to reduce the likelihood of blood-borne pathogen transmission, and some studies have suggested that it reduces the risk for SSIs.37 This is a simple measure to reduce the incidence of infection for the patient and the surgical team and should be carried out routinely. It has been suggested that the outer gloves be changed before shunt components are handled, and several studies have documented a decrease in the incidence of shunt infection when this is done.38,39 Bacteria are present on the outer gloves within 15 minutes of the initiation of surgery38; changing the outer gloves after a period of time, or before handling implants, is reasonable. The use of a no-touch technique has been advocated by many pediatric neurosurgeons, but a recent study from the Hydrocephalus Clinical Research Network did not demonstrate a statistically significant decrease in infection when a no-touch technique was used.40 Antibiotic-impregnated shunt catheters are available for use as external ventricular drains (EVDs) or ventriculoperitoneal shunts. The catheters are impregnated with rifampin and clindamycin (Bactiseal; DePuy, Warsaw, IN; Ares; Medtronic Neurosurgical, Goleta, CA) or with minocycline and rifampin (Ventriclear; Medtronic); no difference in infection rates has been identified between the two catheter types for external ventricular drains.41 One study of adult neurosurgical patients found that the use of antibiotic-impregnated EVDs was as effective as the use of systemic antibiotics in preventing cerebrospinal fluid (CSF) infection.42 Other studies have found a clear reduction in EVD infections with the use of antibiotic-impregnated catheters and have recommended their routine use.43,44 The use of prophylactic systemic antibiotics while an EVD is in place has been found to reduce the incidence of infection in multiple studies.44 The use of antibiotic-impregnated shunt catheters may provide a reduction in shunt infection.31 A multicenter research initiative under way through the Hydrocephalus Clinical Research Network aims to identify factors that reliably decrease the incidence of shunt infections. The use of antibiotic-impregnated shunt catheters is one of the factors being investigated at present. Skin closure is largely a matter of surgeon preference. Staples, nylon sutures, and absorbable sutures are options. There is a significant advantage to not having to remove sutures or staples in combative or fearful children. The use of staples may be associated with a lower risk for infection than the use of suture, but staples are also associated with increased pain.45 Some surgeons apply an antibiotic ointment, such as bacitracin (X-Gen Pharmaceuticals, Big Flats, NY) or Neosporin (neomycin sulfate, polymyxin B, and bacitracin zinc; Johnson & Johnson, New Brunswick, NJ), over the suture line before applying a dressing. Others use petrolatum gauze (Xeroform; Covidien, Mansfield, MA). Dressings range from 2-octyl cyanoacrylate (Dermabond; Ethicon, Somerville, NJ), to Telfa (Kendall [Covidien]) secured with paper tape or a clear occlusive dressing (Tegaderm; 3M), to full head wraps with bandage roll, such as Kerlix (Covidien) and Kling (Johnson & Johnson). Dressings are typically left in place for 24 to 48 hours. There is no advantage to leaving dressings in place longer, or in replacing them, over conventionally closed incisions. Some argue that dressings are unnecessary.46,47 The need to keep a child’s hands away from the incision frequently mandates a dressing, however. The application of tissue adhesives, such as Dermabond, in addition to sutures may provide a lower risk for infection than suture or staples alone, although tissue adhesives should not be used independently for primary wound closure, as the risk of dehiscence is unacceptably high.48,49 One small study demonstrated a lower rate of shunt infection with the use of subcuticular sutures and Dermbond than with conventional nonabsorbable skin sutures.50 The use of antibiotic-coated suture has become popular over the last ten years. Triclosan-coated polyglactin 910 (Vicryl Plus, Ethicon) and poliglecaprone 25 with triclosan (Monocryl Plus, Ethicon) are the most popular choices. There are approximately as many studies concluding that the use of antibiotic-coated suture reduces the incidence of SSIs as there are that the use of antibiotic-coated suture has no impact on the incidence of SSIs.51–54 The cost of antibiotic-coated suture is approximately 50% higher than the cost of standard suture,55 so consideration of its use should not be taken lightly. The use of antimicrobial suture for wound closure resulted in lower infection rates in 61 patients undergoing 84 ventriculoperitoneal shunt surgeries in a prospective, randomized, double-blinded controlled trial.56 Healthy children readily tolerate the absence of oral intake overnight. Standard preoperative instructions include nothing by mouth for 8 hours before surgery, with the following exceptions. Breast milk is allowed until 4 hours before surgery, and formula until 6 hours before surgery. Clear liquids, such as water, apple juice, Pedialyte (Abbott Nutrition; Columbus, OH), and Gatorade (Pepsico, Purchase, NY), are allowed until 2 hours before surgery. Medications normally taken in the morning are to be taken more than 2 hours before surgery with a sip of water. Certain medical conditions, such as moyamoya syndrome and diabetes insipidus, mandate that a patient be admitted to the hospital preoperatively for intravenous (IV) hydration overnight. These recommendations are based on the desire to limit both surgical and anesthetic complications associated with the procedure. In general, hospitalized children beyond the neonatal period do best with 0.45% normal saline (½ NS) or more concentrated solutions, although there are reports of hyponatremia even with ½ NS.57–59 Perioperative patients are more likely to develop hyponatremia than nonsurgical patients.60 Neonates have higher water needs per kilogram and usually require D10 ¼ NS. Infants become dehydrated faster than older patients because of their higher body surface area. The use of isotonic fluids like NS or lactated Ringer solution (LR) is increasingly prevalent, as it avoids hyponatremia and prevents the hemolysis that results from hypotonic solutions. ¼ NS (osmolality 77) should not be administered peripherally, but D5 ¼ NS (osmolality 355) can be. Moderately hypertonic solutions are not problematic. Daily maintenance IV fluid requirements are calculated as follows: 100 mL/kg for the first 10 kg, 50 mL/kg for the second 10 kg, and 20 mL/kg thereafter, with an upper limit of 2,400 mL/d. Sensible fluid losses, those that can be measured, including those from urine, stool, and nasogastric tube output, as well as surgical wounds, should be replaced. Insensible losses of water include water lost in perspiration and respiration, and these must be estimated. Exhaled air contains nearly 100% humidity. Maintenance fluids need to be adjusted for circumstances in which insensible losses (normally one-third of water loss) are increased, such as fever, the use of radiant warmers for premature infants (who may require 120 to 180 mL/kg/d), emesis or nasogastric suction, or the use of nonhumidified mechanical ventilation. In general, the larger the incision, the larger the amount of intraoperative sensible fluid loss. And the smaller the patient, the larger the insensible losses because of the relatively large surface area of the skin. The addition of dextrose to IV fluids is often avoided unless a patient’s status is nothing by mouth (NPO) for a prolonged period of time or hypoglycemia (glucose < 70 mg/dL) is identified on blood testing. There is strong evidence that hyperglycemia increases the extent of infarction after stroke, so hyperglycemia is avoided to protect the vulnerable margins of a cortical resection. Hyperglycemia is also avoided for 24 to 48 hours following severe closed head injury, as several studies have demonstrated poorer outcomes in patients who were hyperglycemic on admission or during their hospitalization.61,62 Even a single dose of dexamethasone during the course of a craniotomy significantly elevates the serum glucose level and should be avoided in patients for whom hyperglycemia could be detrimental.63 Hypoglycemia, however, particularly in neonates, entails its own set of problems and must be avoided as well. Whereas adults are able to maintain near-normal blood glucose levels for days, healthy neonates and young children become hypoglycemic after 24 to 48 hours. The glycogen stores of young children are adequate to maintain the serum glucose for no more than 5 to 10 hours. Because of the small muscle mass of children, oxidative metabolism of fatty acids occurs early, along with ketone body production. The relatively increased glucose requirements in children frequently result in hypoglycemia. The glucose in maintenance IV fluid is sufficient to provide approximately 20% of a patient’s caloric needs, preventing ketoacidosis, but allows the loss of 0.5 to 1% of their weight each day. Neonatal hypoglycemia may be manifested by cyanosis, apnea, hypothermia, hypotonia, lethargy, or seizures. Consideration should be given to adding dextrose to IV fluid in neonates if enteral feeds are not expected to commence within 2 to 3 hours of surgery. Neonates require a glucose infusion rate of at least 6 to 8 mg/kg/min, which will usually be adequately supplied with a solution of D10 ¼ NS for term babies, with a goal serum glucose level above 60 mg/dL. Serum glucose levels should be closely monitored in patients who are NPO and not receiving dextrose in their IV fluids. Intraoperatively, IV fluid management depends upon the goals of the surgery and estimated losses. Hyponatremia is meticulously avoided in all cases. Patients with brain tumors are maintained slightly hypovolemic, often with the assistance of mannitol, 3% NS, or furosemide (Lasix; Sanofi, Bridgewater, NJ), to minimize vasogenic edema of the tissue surrounding the tumor and to minimize the degree of retraction necessary to visualize and resect the tumor. In contrast, patients with moyamoya are aggressively hydrated, as their impaired cerebrovascular autoregulation limits their ability to adapt to hypovolemia without the risk for cerebral infarction. LR is routinely used as replacement for CSF in patients undergoing intraventricular endoscopy; this limits the incidence of mutism, which can develop postoperatively in these patients for reasons that are not understood.64,65 Patients enter the OR with a fluid deficit from being NPO for a number of hours before surgery. This is calculated as the maintenance IV fluid requirement multiplied by the number of hours the patient has been NPO, and the deficit is generally replaced in the first 2 to 3 hours of surgery. If the surgery lasts less than 2 hours, fluids can be replaced more rapidly and often are at the start of surgery because of relative hypotension in the dehydrated patient, although rapid replacement of fluid can be very undesirable in patients undergoing craniotomy and must be discussed with the anesthesiologist before the start of the case. Fluid replacement in the OR includes crystalloid, colloid, and blood products. The SAFE Study indicated that colloid replacement in patients with a disrupted blood–brain barrier (secondary to traumatic brain injury) was associated with higher mortality, so colloid (e.g., albumin) is almost never used in neurosurgical patients.66 A neurosurgical patient’s hemoglobin level is generally maintained at about 9 to 10 g/dL intraoperatively. Transfusion above a hemoglobin level of 8 g/dL has not been shown to lead to better outcomes, with the exception of patients with acute myocardial ischemia.67 In selected patients with vascular or cardiac issues, transfusion at a higher hemoglobin level may be warranted.68 The decision regarding intraoperative and postoperative transfusion should be based upon hemodynamic parameters (hypotension, tachycardia), and usually occurs at a hemoglobin concentration of 7 to 8 g/dL in patients without cardiac or central nervous system pathology.68 A preoperative and intraoperative platelet count of 100,000/µL is necessary for hemostasis in neurosurgery, although much lower platelet counts are tolerated in general surgical patients (50,000/µL) and stable nonsurgical patients (10,000/µL).69 Similarly, a prothrombin time (PT) of less than 16 seconds, an international normalized ration (INR) of less than 1.2, and a partial thromboplastin time (PTT) of less than 39 seconds (depending on the norms at an individual institution) are necessary for intraoperative hemostasis, although values moderately outside of these limits do not produce bleeding difficulties in nonsurgical patients. The administration of fresh frozen plasma (FFP) has few guidelines but is generally recommended for patients with warfarin-related intracranial hemorrhage and for those undergoing massive transfusion.70 Recent articles indicate that an INR of 1.5 (or possibly higher) does not produce increased bleeding in neurosurgical procedures, and that the administration of FFP produces fluid overload and an unnecessary delay in surgical intervention.71 A massive transfusion protocol has been established at the University of Pittsburgh for patients with major blood loss resulting from trauma or surgery (in whom the use of 10 units of packed red blood cells [PRBCs] is expected) in response to literature suggesting improved survival in patients transfused with high FFP- and platelet-to-red blood cell ratios.72–74 A pediatric massive transfusion protocol has been proposed, based on the same principles; triggers of persistent hemodynamic instability or ongoing bleeding after the infusion of 40 mL/kg of crystalloid are used to initiate the transfusion of PRBCs (30 mL/kg). The addition of FFP (20 mL/kg) is made after the loss of one blood volume, and the addition of platelets (20 mL/kg) and cryoprecipitate (4 mL/kg) after the loss of two blood volumes.75 The off-label use of recombinant factor VII (Novoseven; Novo Nordisk, Plainsboro, NJ) can be considered in cases of continued blood loss.75 Nearly all pediatric surgical patients (not only neurosurgical) are now placed on isotonic fluids (either NS or LR) in the postoperative period.76 It is clear that hypotonic maintenance IV fluids cause hyponatremia in children, with the potential for the development of hyponatremic encephalopathy, cerebral edema, and death in children who have severe infections or are in the postoperative state.77 A trial in 258 postoperative patients revealed that hypotonic IV fluids (0.45% NS in this study) significantly increased the risk for hyponatremia compared with isotonic IV fluids (0.9% NS).78 The development of hyponatremia is felt to be a function of the tonicity of the maintenance fluids, rather than of the volume of fluid administered.79 Antidiuretic hormone (ADH) levels are normally elevated in the 24 hours following surgery, and fluid restriction delays the normalization of ADH levels.80 Sodium losses from an EVD can warrant replacement if hyponatremia develops. Postoperatively, it is common for neurosurgical patients to be placed on NS. Sodium levels are strictly maintained at the upper limit of normal or higher (140 to 150 mEq/L is a fairly standard range) in the majority of pediatric neurosurgical patients. The blood–brain barrier is often disrupted at least locally in these patients, allowing the passive diffusion of water into the brain and a corresponding increase in cerebral edema and intracranial pressure. The blood–brain barrier comprises tight junctions between the endothelial cells lining the capillaries of the brain; the astrocytic foot processes surrounding the capillaries may play a minor role as well. The blood–brain barrier is disrupted by prematurity, infection, ischemia and stroke, neoplasia, hypertension exceeding the limits of autoregulation, and trauma (including surgery).81 It can be intentionally disrupted with the use of hyperosmolar solutions, such as mannitol, to improve the delivery of chemotherapeutics. Increasing the sodium level in the bloodstream decreases the amount of free water in the brain, both intracellularly and extracellularly. The IV use of hypertonic saline or mannitol exerts an osmotic pressure gradient across the blood–brain barrier, allowing water to diffuse down the osmotic pressure gradient into the bloodstream. When the blood–brain barrier is disrupted, these molecules freely enter the brain through the widened tight junctions, leading to edema, excitotoxicity secondary to the accumulation of excitatory amino acids, and the loss of cerebral autoregulation. Steroids and hypothermia are thought to reduce the permeability of the blood–brain barrier. Hypertonic saline at various concentrations, with 3% NS the most common (a bolus dose of 3 to 10 mL/kg or a continuous infusion beginning at 1 mL/kg/h and increased to effect), is used as a treatment for intracranial pressure (ICP) above 20 mm Hg in pediatric traumatic brain injury, titrated to an ICP below 20 mm Hg and a serum osmolality below 360 mOsm/L.82 Mannitol is also frequently used, although clinical studies demonstrating its efficacy are lacking.82 Doses of 1 g/kg reduce ICP through a reduction in blood viscosity in the short term (less than 75 minutes) and through an osmotic effect lasting up to 6 hours. This effect requires an intact blood–brain barrier; mannitol may diffuse into injured areas of the brain, pulling free water into the parenchyma.83,84 3% NS functions via similar mechanisms, although much higher serum osmolarity is tolerated by patients given 3% NS than by those given mannitol.85 3% NS is also useful in treating cerebral salt wasting. Antiepileptic drugs (AEDs) are often administered prophylactically in the perioperative period. The risk for postoperative epilepsy ranges from 12 to 17%.86,87 The administration of phenytoin in the perioperative period was shown to reduce the chances of postoperative epilepsy from 17 to 8% in a double-blinded trial of 203 patients undergoing craniotomy.88 The chances of perioperative seizure can be increased (sevoflurane, enflurane) or decreased (thiopental, halothane) by the use of certain anesthetics.89 A seizure can be induced in a patient with epilepsy by using anesthetics that are not proconvulsant in the normal brain (e.g., ketamine, isoflurane).89 The same anesthetic drug can be proconvulsant or anticonvulsant, depending on the clinical setting and dose.90 Risk factors for perioperative seizures include a supratentorial tumor, age younger than 2 years, and hyponatremia due to inappropriate secretion of antidiuretic hormone (SIADH) or cerebral salt wasting.91 Nonaccidental trauma, prehospital hypoxia, a Glasgow Coma Scale score of less than 8, and subdural hematoma have also been identified as risk factors for seizure in trauma patients.92,93 AEDs decrease the early (< 7 days) but not the late (> 7 days) risk for seizure after head injury in adults.82,94,95 Studies have principally been performed with phenytoin, although carbamazepine and phenobarbital have also been shown to provide a reduction in early posttraumatic seizures.95 The combination of phenytoin and phenobarbital appears to be the most effective in reducing provoked seizures; the majority of drugs developed after 1980 have not been tested.96 Levetiracetam and phenytoin are both associated with a low risk for early postoperative seizures, and levetiracetam is associated with fewer early adverse reactions.97 Some small studies of levetiracetam suggest equivalent seizure control and improved neurologic outcomes, but the numbers do not reach statistical significance, and this fact combined with the 20-fold higher cost of levetiracetam suggest that these data be interpreted with caution.98–100 Because of the higher rate of side effects with phenytoin, a common practice is to begin a patient on phenytoin perioperatively and switch to levetiracetam if any side effects occur. This transition has been shown to be safe in a small pilot study.101 Several small studies not reaching statistical significance failed to show a reduction in early posttraumatic seizures for phenytoin in children.102 No drug has been shown to reduce the incidence of late seizures following trauma or craniotomy. The effect on children is extrapolated to be the same as for adults, but confirming data do not exist. The use of AEDs in children following head trauma and craniotomy for other reasons is controversial, and largely a matter of surgeon preference. A common management choice is to load a patient who has a tumor or vascular malformation with an AED at the start of surgery and continue the AED for some period of time thereafter. Although the literature does not support a role for AEDs in preventing late-onset epilepsy, it is certainly not ideal for the recovering brain, unfused craniotomy bone, and healing skin to be subjected to the trauma and hypertension associated with a generalized tonic–clonic seizure. Many pediatric neurosurgeons advocate 6 to 12 weeks of AED therapy after a craniotomy for this reason and taper the drug after that time point, with or without an electroencephalogram (EEG) before or after completion of the taper. The routine use of perioperative dexamethasone to limit peritumoral cerebral edema was initiated in the 1960s.103 The use of dexamethasone has expanded over the years, and it is now used by some surgeons for patients undergoing surgery for aneurysms or arteriovenous malformations, surgery for Chiari malformations, craniofacial surgery, or resection of epileptic foci, in addition to those with brain tumors. Dexamethasone, although accompanied by a host of side effects, including mood changes, increased oral intake, and acne, in addition to the classic cushingoid appearance (buffalo hump, moon facies, abdominal striae), produces an improvement in the neurologic examination findings in some patients, a reduction in nausea and vomiting, improved pain control, and a shortened hospital stay.104–106 Dexamethasone dosing if continued for 5 days must not be abruptly discontinued, to prevent acute adrenal insufficiency; rather, it must be tapered off over a minimum of 5 days, with the rate of recovery dependent upon the length of steroid administration.107 Dexamethasone is a strong glucocorticoid agonist with little to no mineralocorticoid effect, whereas hydrocortisone is a stronger mineralocorticoid agonist than glucocorticoid agonist. Hydrocortisone is thus administered preferentially to patients who have primary or secondary adrenal insufficiency; a classic example is the patient with a craniopharyngioma. Stress dosing should be undertaken for surgery, illness, and fever in patients with panhypopituitarism or adrenal insufficiency.108 Stress dosing is roughly three times the maintenance dose. The necessity of stress dosing has been questioned in many patients on long-term glucocorticoids, but not for neurosurgical patients; a patient receiving high-dose dexamethasone for cerebral edema does not require the additional administration of hydrocortisone.108 A course of dexamethasone is highly effective in treating chemical, or aseptic, meningitis following a craniotomy. The secondary hydrocephalus often resolves after the first few days of a several-week tapering course of dexamethasone. These patients typically present with a fever and meningismus but are alert and interactive, whereas patients with bacterial meningitis are usually somnolent. Once infection has been ruled out with a lumbar puncture demonstrating the absence of bacteria and a lymphocytic predominance, a weight-dependent bolus of dexamethasone followed by regular dosing of a slowly tapering course over several weeks produces resolution of symptoms. Blood pressure management is important, and often critical, in postoperative neurosurgical patients. Patients who have undergone craniotomies for tumor or epilepsy focus resection are predisposed to hemorrhage if allowed to become hypertensive. An upper limit is usually set for the systolic blood pressure (SBP), as intermittent spikes in blood pressure can be reasoned to override tenuous clot formation in, or coagulation of, a blood vessel, eliciting a significant intracranial hemorrhage; upper and lower limits for mean arterial pressures (MAPs) are also typically specified to ensure adequate perfusion of the brain while limiting venous ooze into the resection cavity. Blood pressure and heart rate norms vary with patient age and size, and intra- and postoperative targets should be adjusted accordingly. A general rule for adult patients is to keep the SBP under 140 to 160 mm Hg; in children, the baseline preoperative blood pressure is a good approximation of the goal postoperative blood pressure. β-Blockers (propranolol, metoprolol) reduce cardiac output by reducing contractility and the heart rate. α-Blockers (prazosin, doxazosin) reduce afterload. Mixed α-/β-antagonists (labetalol, carvedilol) may also be used. Calcium channel blockers (nicardipine, nifedipine, verapamil, diltiazem) reduce systemic vascular resistance with minimal effect on contractility. Nimodipine, a calcium channel blocker commonly used for adult patients with aneurysms to reduce the complications of vasospasm, may be used in children to treat or prevent vasospasm. However, the dose and efficacy of this strategy in children have not been established, and children seem to have many fewer complications of vasospasm (behavioral change, stroke) than do adults following aneurysmal subarachnoid hemorrhage.109,110 Angiotensin-converting enzyme (ACE) inhibitors (captopril, lisinopril) produce vasodilation by reducing the body’s production of angiotensin, but they have prolonged durations of action and effects on renal function that limit their usefulness. Vasodilators (hydralazine, minoxidil) are also used for hypertensive control. Nitroprusside causes vasodilation as a nitric oxide donor to the vasculature, reducing afterload, without an effect on contractility. Although its short half-life allows rapid dose titration, the production of cyanide during its metabolism yields significant toxic potential. Nitroprusside may increase ICP by increasing cerebral venous blood volume and should be administered only as a drug of last resort, together with thiosulfate. β-Blockers and calcium channel blockers, particularly labetalol and nicardipine, are the antihypertensives most commonly used in the PICU for neurosurgical patients. Hypotension, relative or absolute, may be equally harmful. Patients who have undergone AVM resection may develop normal perfusion pressure breakthrough (cerebral edema or hemorrhage, thought to be from altered cerebral autoregulation secondary to the restoration of normal blood flow to the chronically ischemic tissue surrounding the AVM) if allowed to achieve normotension.111 Such patients should routinely be maintained at least at the lower limit of cerebral perfusion to reduce the chances of this dangerous syndrome developing. Patients with moyamoya syndrome and resultant altered cerebral autoregulation may have strokes at higher than anticipated cerebral perfusion pressure.112 Patients in spinal shock may require augmentation of their blood pressure, as volume resuscitation is contraindicated in these patients. Selected patients may require the use of pressors to increase the MAP. Epinephrine and dopamine are β-receptor agonists at low doses and α-receptor agonists at high doses. Epinephrine has a narrow therapeutic range and carries a significant risk for adverse reactions; it is used principally for cardiac arrest and anaphylaxis. Low-dose dopamine (0.5 to 5 µg/kg/min) increases renal and cerebral blood flow. Intermediate-dose dopamine (5 to 15 µg/kg/min) increases cardiac output. High-dose dopamine (> 15 µg/kg/min) produces progressive systemic and pulmonary vasoconstriction, increasing afterload and limiting cardiac output. Dobutamine is principally a β1-agonist (increased cardiac output), although it has mild β2 effects (vasodilation). Norepinephrine is an α-receptor agonist that increases systemic vascular resistance through diffuse vasoconstriction, an effect that overshadows its minor effect on cardiac output. This is a second-line drug used principally in septic shock. Dopamine and dobutamine are the drugs of choice for increasing MAPs in the PICU. Nausea and vomiting are common side effects of general anesthesia, and their incidence is increased in children.113 Nausea is partially controlled by the frequent use of intra- and postoperative dexamethasone and histamine2-blockers (such as famotidine and ranitidine) in neurosurgical patients. Anesthesiologists routinely administer at least one antiemetic drug during surgery. The drug of choice for prophylaxis and treatment is a serotonin (5-HT3) antagonist, such as ondansetron (Zofran; GlaxoSmithKline, Philadelphia, PA). Antiemetics like metoclopramide (Reglan), prochlorperazine (Compazine; GlaxoSmithKline), and promethazine (Phenergan; Baxter, Deerfield, IL) have potential neurologic and respiratory side effects and should be used as second-line agents and with caution. Constipation is exceedingly common postoperatively in pediatric patients. This is problematic, as Valsalva maneuvers increase the risk for pseudomeningocele formation and CSF leak. Constipation causes unnecessary pain for the postoperative patient and can needlessly prolong a hospital stay. Patients should be routinely started on an osmotic laxative, such as polyethylene glycol (MiraLax; MSD Consumer Care, Summit, NJ), which is extremely well tolerated by patients, as soon as they begin an oral diet. An emollient stool softener, such as docusate (Colace; Purdue, Stamford, CT), or stimulants, such as senna (Senekot; Purdue) and bisacodyl (Dulcolax; Boehringer Ingelheim, Ridgefield, CT), are more objectionable to pediatric patients because of their taste but are adequate alternatives. Enemas and suppositories are less often necessary when these medications are begun early in a patient’s hospital course. Pain control in surgical patients is a critically important issue. The accurate assessment of pediatric pain is an essential part of postoperative management, as it allows the prevention of both over- and underadministration of pain medication. Many institutions have developed a pediatric pain management service to assist practitioners with this management. The most commonly used scales are CRIES for neonates, the Wong-Baker FACES scale for toddlers, FLACC (face, legs, activity, cry, consolability) for slightly older children, and VAS (visual analog scale) in children older than 7 years.114–119 The administration of local anesthetic, either a long-acting medication (such as bupivacaine) at the start of surgery or a shorter-acting medication (such as lidocaine) at the end of surgery, is effective in providing several hours of postoperative craniotomy pain control.120 Oral sucrose may be helpful in minimizing distress for neonates past 34 weeks of gestation. The usual dose is 0.5 to 1.5 mL of 24% sucrose, given by syringe 2 minutes before the procedure. Breast milk and glucose are reported to be equally effective. Swaddling and nonnutritive sucking are first-line pain-relieving measures in neonates and infants. Acetaminophen and ibuprofen are typically administered in preference to oral opioids. Many patients require only acetaminophen or ibuprofen after a proximal ventriculoperitoneal shunt revision. Several studies have suggested that ibuprofen provides better pain relief than codeine or acetaminophen with codeine in children with long bone fractures.121,122 Topical skin anesthetics, such as EMLA and amethocaine gel, should be considered before painful procedures like IV placement, lumbar puncture, or suturing in infants and children when possible, to minimize the pain and distress associated with the procedure.123 Opioid administration is common, although the topic is controversial. Many neurosurgeons limit opioid administration in their postoperative patients because of a concern that somnolence or vomiting from postoperative cerebral edema or hemorrhage could be dismissed as a medication side effect. Opioids are to be administered in a response-dependent manner. Side effects like respiratory depression and somnolence occur after pain control has been achieved, so opioids should not be withheld for pain control because of a fear of these side effects. The most commonly used IV medication is morphine; hydromorphone (Dilaudid; Purdue) is occasionally used as a second-line drug in patients whose pain is refractory. The use of IV opioids should always be considered in neonates, in whom oral opiates are not recommended, although acetaminophen is highly effective in these patients. A morphine dose of 0.02 to 0.05 mg/kg IV every 4 to 8 hours may be used with caution in neonates, with close monitoring for respiratory depression, as pharmacokinetics in this age group are variable.124 Morphine metabolism is decreased in infants younger than 6 months of age, and infants correspondingly require lower doses of morphine for pain control.125 When older patients begin tolerating an oral diet, the IV opioid is typically transitioned to an oral opioid like oxycodone or hydrocodone. These drugs are favored over codeine because they provide more consistent analgesia. Oral morphine is three times less potent than IV morphine, and dosing must be adjusted accordingly.126 Pediatric patients are likely to develop symptoms of opioid withdrawal after as little as 5 days of fentanyl infusion and may require transition to a tapering dose of methadone.127 Patient-controlled analgesia (PCA), although infrequently used in young children, provides superior pain control in children older than 5 years of age.128 PCA by proxy (parental administration of opioid) has been shown to have an increased incidence of respiratory depression secondary to opioid overdose, and it must be used with caution and extensive education of the proxy user.129 Medications like low-dose ketamine (a dissociative anesthetic) can be added to the PCA for improved pain control and decreased opioid administration. Epidural PCA is used in patients undergoing spinal surgery, although rarely in patients undergoing craniotomy. A continuous epidural morphine infusion, with or without bupivacaine, has been demonstrated to be highly effective in patients undergoing lumbar dorsal rhizotomy.130 Meta-analyses have demonstrated that continuous epidural analgesia is more effective in controlling postoperative pain than IV opioids (via either PCA or intermittent administration).131,132 Patients having spinal surgery, to include posterior fossa surgery, frequently experience spasm in the paraspinous musculature. This responds well to the administration of diazepam (Valium) or cyclobenzaprine (Flexeril). These drugs have a sedating effect and must be administered with caution. The use of IV ketorolac (Toradol; Roche, Nutley, NJ) has been controversial in neurosurgery. Many neurosurgeons are reluctant to use it because of a concern about postoperative hemorrhage. Reports exist in the literature describing the development of spinal epidural hematomas in patients on ketorolac. One such case report describes an elderly patient who developed a spinal epidural hematoma after a lumbar puncture while on enoxoparin, aspirin, and ketorolac.133 No reports of intracranial hemorrhage in patients receiving ketorolac exist in the literature to date. A study of 89 adult patients undergoing lumbar microdiskectomy demonstrated improved pain control and no increased bleeding (from surgical wound or by coagulation parameters) with a single dose of ketorolac administered at the termination of surgery.134 Multiple studies have confirmed the absence of an increased risk for postsurgical bleeding with the use of ketorolac in children, with the possible exception of those undergoing tonsillectomy.135 Ketorolac is not recommended for use in children younger than 1 year of age, although it is routinely used at Children’s Hospital of Pittsburgh in infants who have subgaleal drains in place. An increased risk for bleeding has been demonstrated in infants younger than 21 days of age.136 Ketorolac is routinely used at Children’s Hospital of Pittsburgh in the postoperative setting, particularly for pain control in patients undergoing Chiari decompressions or craniosynostosis repair, starting as soon as immediately postoperatively. Some surgeons opt to begin therapy with ketorolac a day or two following surgery, if clinically warranted. An additional concern centers on conflicting reports in the literature suggesting that the use of nonsteroidal anti-inflammatory medications may inhibit spinal fusion. An increased rate of pseudarthrosis is well reported in adults.137–139 More recent literature has indicated that the short-term use of ketorolac (48 hours postoperatively) has no effect on spinal fusion.140 A predisposition to pseudarthrosis or nonunion has not been demonstrated in children following instrumentation for scoliosis.141 Ketorolac produces superior pain control with fewer side effects than opioids, and although the risks must be weighed, its use is highly effective in many patients. Activity restrictions are imposed regularly for pediatric neurosurgical patients. Little evidence exists, however, to guide these decisions. Only the results of practice surveys completed by practicing pediatric neurosurgeons exist for most clinical questions. Return to school or day care is an important consideration. Children are kept out of crowded situations like school or day care because of concerns regarding possible injury to the incision, pain production, bleeding into a resection cavity, infection, and possibly the management of new neurologic deficits. Most children are able to return to school or day care within 2 weeks of a craniotomy. Pain and limited mobility may prevent patients undergoing spinal surgery from returning to school for 4 to 6 weeks. Older children may need to remain out of school for a longer period of time and undergo home tutoring so that they will be at the same level academically as their peers when they return to the classroom. Most school districts are mandated to provide a home tutor for any patient deemed by a physician to be unable to return to school for 4 weeks. It is logical that Valsalva maneuvers should be limited following intradural spinal or posterior fossa surgery for a period of time following surgery, to minimize pseudomeningocele formation or CSF leak. This author has not observed pseudomeningocele formation more than 6 weeks postoperatively and uses this time period to instruct families to limit Valsalva maneuvers. Patients are restricted from lifting more than 5 pounds, straining, and physical activity beyond walking during this time. Patients are placed on stool softeners to limit constipation. Following the 6 weeks, these restrictions are lifted completely for the majority of patients. Patients who have undergone Chiari decompression undergo a slower mobilization regimen, with running and swimming permitted after 6 weeks and noncontact competitive sports permitted after 3 to 6 months, depending upon the patient and the sport. Return to gym class depends upon the patient’s neurologic deficits and typically occurs at least 4 to 6 weeks following return to school. Return to sports is a particularly urgent issue for many families. Cranial concerns include the strength of the fusion between the craniotomy bone and the skull, the risk for hemorrhage associated with neovascularization and scar tissue, and risks for CSF dynamic alteration.142 While return to sports following concussion has been widely discussed and multiple sets of guidelines exist, return to sports following craniotomy has not been studied in a systematic way. Current guidelines for sports-related concussion advise against return to play in patients with neurologic symptoms, with or without provocative testing.143 This guideline should certainly be observed in patients recovering from a craniotomy. Any patient with permanent central neurologic deficits should be prohibited from a return to contact sports.142 Most neurosurgeons insist that computed tomography of the head demonstrate fusion of the craniotomy bone before an athlete is permitted to return to play.144 Patients undergoing posterior fossa surgery should be counseled about the potential decrease in ligamentous stability at the craniocervical junction as a result of the surgical approach. Return to sports following spinal injury or surgery is problematic, as well. Several issues make the pediatric spine more vulnerable to injury than the adult spine. The pediatric head is larger relative to the body than is the adult head, allowing increased momentum with rapid movement. The pediatric paraspinous muscle mass is relatively less well developed, smaller, and more flexible than that of the adult, rendering it less able to withstand significant forces. The ligaments of the pediatric spine are more flexible than those of the adult, allowing more movement within the vertebral column and resultant spinal cord injury, without an identifiable ligamentous injury. This mobility, combined with the frequent finding of cervical spinal stenosis, is thought to be responsible for the “stinger” phenomenon seen commonly in high school football players. A stable spine by established criteria (normal strength, full range of motion without pain, and the absence of compressive forces) is a baseline requirement for return to play. Patients who have undergone spinal fusion are prohibited from collision sports on a permanent basis by the majority of spinal surgeons, from contact sports by some, and from noncontact sports (golf, swimming) for a variable period of months following surgery.145 The decision regarding return to play must be made by the neurosurgeon and parents in conjunction, as only the family can determine their level of risk aversion. Restriction of unoperated patients from activity is controversial. Because of case reports in the literature regarding subdural hematoma formation following participation in sports, including basketball, soccer, and football, in patients with arachnoid cysts, counseling of the parents regarding the risks associated with contact sports is held.146–148 Both arachnoid cysts and asymptomatic Chiari malformations are viewed as relative contraindications to participation in contact sports.142 Many pediatric neurosurgeons restrict patients with ventriculoperitoneal shunts from participating in contact sports, although the established risk for complications (including shunt disconnection and subdural hematoma formation) is well under 1%.149 With little information in the literature to inform decision making, counseling of the family regarding the potentially serious consequences must be undertaken in these cases. A final topic, critical to the care of the pediatric neurosurgical patient, is the relationship between the neurosurgeon and the parents of the patient. The parents have been placed in the excruciating role of trusting a relative stranger to cut open their child’s body, fix a problem, and return the child to them in the same or better condition. They must acknowledge their inability to protect their child from the hardships of life, and even from the pain of surgery. They feel helpless and desperate. The stress for some families is intolerable. Extensive discussion of the risks and benefits of surgery, as well as the likely outcome, lessens the burden somewhat. We in pediatric neurosurgery frequently see families at the most stressful moments of their lives, and we must deal with them with the utmost sensitivity. Pearls
4.1 Preoperative and Perioperative Management
4.1.1 Patient Preparation for Surgery
Abbreviation
Definition
ACE
Angiotensin-converting enzyme
ADH
Antidiuretic hormone; vasopressin
AED
Antiepileptic drug
AVM
Arteriovenous malformation
CFU
Colony-forming unit
EEG
Electroencephalogram
EMLA
2.5% lidocaine, 2.5% prilocaine; dermal anesthetic
EVD
External ventricular drain
FFP
Fresh frozen plasma
ICP
Intracranial pressure
INR
International normalized ratio
IV
Intravenous
LR
Lactated Ringer solution
MAP
Mean arterial pressure
NPO
Nil per os (“nothing by mouth”)
NS
Normal saline solution (0.9% sodium chloride)
OR
Operating room
PICU
Pediatric intensive care unit
PO
Per os (“by mouth”)
PR
Per rectum
PRBCs
Packed red blood cells
PT
Prothrombin time
PTT
Partial thromboplastin time
SBP
Systolic blood pressure
SQ
Subcutaneous
SSI
Surgical site infection
4.1.2 Preoperative Medication Management
Drug
Dosing
Acetaminophen (Tylenol; McNeil, Fort Washington, PA)
PO/PR: Neonates: 10–15 mg/kg/dose every 6–8 hours Infants and children: 10–15 mg/kg/dose every 4–6 hours, maximum 3.25 g/day
Aspirin
PO: 3–10 mg/kg/day rounded to a convenient dose, maximum 325 mg/day
Bisacodyl (Dulcolax; Boehringer Ingelheim, Ridgefield, CT)
PO: Children 3–12 years: 5–10 mg/dose (0.3 mg/kg) Children ≥12 years: 10 mg/dose
Bupivacaine (Marcaine; Hospira, McPherson, KS)
SQ: 0.25% bupivacaine, 1:200,000 epinephrine; 1 mL/kg patient weight
Carbamazepine (Tegretol; Novartis, East Hanover, NJ)
PO: Initial: 10–20 mg/kg/day (up to 200 mg/day) divided into 2–3 times daily as tablets or 4 times daily as suspension; increase weekly by up to 100 mg/day until optimal response achieved; maximum 35 mg/kg/day or 1,000 mg/day Maintenance: divide into 3–4 doses
Cefazolin (Ancef; GlaxoSmithKline, Philadelphia, PA; Kefzol; Lilly, Indianapolis, IN)
IV: 15–25 mg/kg/dose; maximum 6 g/day
Clindamycin (Cleocin; Pfizer, New York, NY)
IV: 10 mg/kg preoperatively; maximum 600 mg/dose
Cyclobenzaprine (Flexeril; Janssen, Titusville, NJ)
PO: Children: dosage not established Adolescents: 5 mg 3 times daily, may increase to 7.5–10 mg/dose; use for more than 2–3 weeks not recommended
Dexamethasone (Decadron; Merck, Whitehouse Station, NJ)
IV/PO: Loading dose: 1–2 mg/kg/dose up to 10 mg/dose Maintenance: 1–1.5 mg/kg/day divided every 4–6 hours; maximum 16 mg/day; tapered off over 5 days
Diazepam (Valium; Genentech, South San Francisco, CA)
IV: 0.04–0.3 mg/kg/dose every 2–4 hours to a maximum of 0.6 mg/kg/8 h PO: 0.12–0.8 mg/kg/day divided every 6–8 hours, maximum 10 mg/dose
Dobutamine (Dobutrex; Lilly)
IV: 2–15 µg/kg/min continuous infusion, titrate to response; maximum 15 µg/kg/min
Docusate sodium (Colace; Purdue, Stamford, CT)
PO: 5 mg/kg/day divided into 1–4 doses, maximum 400 mg/day
Dopamine (Intropin; DuPont, Wilmington, DE)
IV: 1–20 μg/kg/min continuous infusion, maximum 50 µg/kg/min
Famotidine (Pepcid; Merck)
PO: Neonates and infants < 3 months: 0.5–1 mg/kg/dose every 24 hours IV: Neonates and infants < 3 months: 0.25–0.5 mg/kg/dose every 24 hours PO/IV: Children > 3 months: 0.5 mg/kg/dose twice daily, maximum 40 mg/day
Fentanyl
IV: Continuous analgesia: 1–2 µg/kg bolus, then 0.5–2 µg/kg/h, titrate to effect
Furosemide (Lasix; Sanofi, Bridgewater, NJ)
IV: Neonates: 1–2 mg/kg/dose every 12–24 hours Infants and children: 0.5–2 mg/kg/dose every 6–12 hours
Hydrocortisone (Cortef and Solu-Cortef; Pfizer)
IV stress dose for surgery: 50 mg/m2 bolus followed by 50 mg/m2/day as continuous infusion for 48–72 hours IV stress dose for neonates: 20–30 mg/m2/day divided into 2 or 3 doses IV stress dose in PICU: 2 mg/kg/dose x 1, then 2 mg/kg/day as a continuous infusion or divided every 6 hours PO physiologic replacement: Neonates: 7–9 mg/m2/day divided every 8–12 hours Children: 0.5–0.75 mg/kg/day divided every 8 hours
Hydromorphone (Dilaudid; Purdue)
IV: 0.008 mg/kg/dose every 3 hours as needed, up to 0.015 mg/kg/dose
Ibuprofen (Advil, Pfizer; Motrin, McNeil)
PO: 4–10 mg/kg/dose every 6–8 hours, maximum 40 mg/kg/day or 3.2 g/day
Ketorolac (Toradol; Roche, Nutley, NJ)
IV: 0.5 mg/kg/dose every 6 hours up to 30 mg/dose; do not exceed use beyond 5 days
Labetalol (Normodyne; Schering, Kenilworth, NJ)
IV: 0.2–0.5 mg/kg/dose as frequently as every 10 minutes; maximum 20 mg/dose
Levetiracetam (Keppra; UCB Pharma, Smyrna, GA)
IV/PO: 5–10 mg/kg/day divided into 2–3 doses, increased weekly to 60 mg/kg/day maximum; dose not established in children < 16 years although off-label use frequent
Mannitol (Osmitrol; Baxter, Deerfield, IL)
0.25–1 g/kg every 6 hours; maximum 50 g every 6 hours, maintaining serum osmolality at 310–320 mOsm/L
Metoclopramide (Reglan [injectable]; Baxter; Reglan [oral]; Alaven, Marietta, GA)
IV: 0.1–0.2 mg/kg/dose, repeated every 6–8 hours as needed; maximum 10 mg/dose
Morphine sulfate
IV: Neonates: 0.02–0.05 mg/kg/dose every 4–8 hours, maximum 0.2 mg/kg/dose Infants 0 to < 6 months: 0.02 mg/kg/dose every 4–6 hours, maximum 0.06 mg/kg/4 h Infants ≥ 6 months: 0.05 mg/kg/dose every 4–6 hours, maximum 0.12 mg/kg/4 h Children: 0.05 mg/kg/dose every 3 hours as needed, escalate to 0.15 mg/kg/dose as needed; maximum 15 mg/dose in children Adolescents (> 50 kg): 2.5–10 mg every 3 hours as needed PO: oral dose one-third as effective as IV dose
Naproxen (Aleve; Bayer, Pittsburgh, PA; Naprosyn; Roche)
PO: children > 2 years: 5–7 mg/kg/dose every 8–12 hours; maximum 1,000 mg/day
Nicardipine (Cardene; EKR Therapeutics, Bedminster, NJ)
IV: 0.5–1 μg/kg/min via continuous infusion, titrating to goal blood pressure with increases every 15–30 minutes; maximum dose 4–5 µg/kg/min
3% Normal saline
3–10 mL/kg as a bolus dose, or a continuous infusion beginning at 1 mL/kg/h and increased to effect, maintaining serum osmolarity < 360 mOsm/L
Oxycodone (OxyIR; Roxicodone; Xanodyne, Newport, KY)
PO: 0.05–0.1 mg/kg/dose every 3 hours as needed; escalate as needed to 0.15 mg/kg/dose; begin at 5–10 mg/dose in adolescents > 50 kg
Phenobarbital
IV loading dose: 10–20 mg/kg; maximum 30–40 mg/kg IV/PO maintenance dose: Neonates: 3–4 mg/kg/day in 1–2 doses, increase to 5 mg/kg/day if needed Infants: 5–6 mg/kg/day in 1–2 doses Children 1–5 years: 6–8 mg/kg/day in 1–2 doses Children 5–12 years: 4–6 mg/kg/day in 1–2 doses Children > 12 years: 1–3 mg/kg/day in 2 doses
Phenytoin
IV: Loading dose: 15–20 mg/kg Maintenance dose: begin at 5 mg/kg/day divided into 3 doses, may increase to 10 mg/kg/day
Polyethylene glycol (MiraLax; MSD Consumer Care, Summit, NJ; GlycoLax; Kremers Urban, Seymour, IN)
PO: 0.5–1.5 g/kg/day, not to exceed 34 g/day; titrate to goal of two soft stools per day
Sucrose
Vancomycin
IV: Children 1 month–12 years: 15 mg/kg/dose every 6 hours Children > 12 years: 15 mg/kg/dose every 8 hours; maximum 2,000 mg/dose
Note: All dosages should be confirmed with the hospital pharmacist before administration.
4.1.3 Infection Control Measures
4.1.4 Fluid and Electrolyte Management
4.2 Postoperative Management
4.2.1 Fluid and Electrolyte Management
4.2.2 Perioperative Medications
4.2.3 Pain Management
4.2.4 Restriction of Activity
4.3 Conclusion
Stay updated, free articles. Join our Telegram channel

Full access? Get Clinical Tree
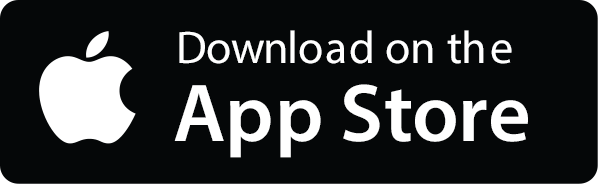
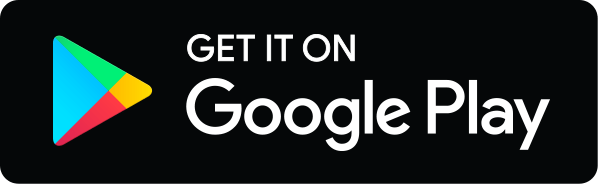