Cervical Spine Injuries in Children
Stephen R. Thompson
Paul D. Sponseller
Cervical spine injuries in children represent a different spectrum of pathology compared to adults. Injury to the cervical spine typically involves the upper three segments, while in older children and adults, the subaxial spine is more commonly injured. Dens fractures, atlantooccipital instability, atlas fractures, atlantoaxial rotatory subluxation, os odontoideum, spondylolysis of the axis, and hangman’s fractures represent the bulk of cervical spine injuries in children. Children are also more likely to sustain an associated neurologic injury than adults, and spinal cord injury without radiographic abnormality (SCIWORA) remains almost exclusively a syndrome in the pediatric population. The fatality rate in children who sustain cervical spine injury is double that of their older counterparts.
Successful treatment of pediatric cervical spine fractures demands not only the recognition of patterns of injury but also an understanding of normal development and anatomy. Also needed is knowledge of the least invasive means of effective treatment for children. Recent developments in this field have included a more evidencebased approach to evaluation of a suspected cervical spine injury in a child, a better understanding of how to treat injuries in children, as well as a more complete elucidation of the epidemiology of these injuries.
CONCEPTS AND PRINCIPLES
EPIDEMIOLOGY
Fractures and dislocations of the cervical spine occur less commonly in children and represent only about 2% of all spine trauma. Even among pediatric trauma patients, cervical spine injuries are uncommon, with an incidence between 1% and 2% (1,2). The fatality rate in children with cervical spine trauma ranges from 7% to 26%, which represents a range double to triple that of adults (1,2). The majority of these deaths occur in children younger than 10 years of age and are typically due to a concomitant severe head injury (1).
The transition between pediatric and adult patterns of injury occurs between age 8 and 11 (Fig. 33.1). The reasons for the differences between younger and older groups appear to involve the relatively larger head size (3) in proportion to the rest of the body, as well as delay in muscular control of the head and protective reflexes, thus predisposing them to flexion and extension injuries. In addition, the fulcrum of cervical spine motion is between C2 to C3 in the young child compared to a fulcrum at C5 to C6 in the adolescent and adult. Ligamentous laxity is greater in children, and the cervical facets are more horizontal, allowing a greater range of physiologic flexion and extension (4).
The most common mechanism of injury across all ages is a motor vehicle accident, whether the injured is an occupant, pedestrian, or cyclist. The etiology of younger children’s injuries is more likely to include falls or being struck as a pedestrian by a motor vehicle (5,6). Other causes unique to this age group include birth trauma and nonaccidental injury (7,8). Breech presentation may predispose to a traction injury that affects the lower cervical or thoracic spine (8,9). Cephalic presentation more rarely accompanies spinal cord injury; when it does, it more often involves the upper cervical spine (9). Older children and adults, by contrast, are more likely to suffer cervical spine injury during sports, such as football, trampoline use, and diving (5,10,11). Gunshot wounds have shown a steady increase in frequency over the past decade. Although the thoracic spine is the most common region affected by gunshot injury, the cervical spine also may be injured; these injuries usually involve teenagers.
NORMAL DEVELOPMENT AND ANATOMY
It is important to have an understanding of the growth of the child’s cervical spine in order to interpret injury patterns and distinguish them from normal variants. The atlas develops from three ossification centers: a single anterior center for the body and paired posterior centers for the neurocentral arches (Figs. 33.2 and 33.3A). Ossification centers in the posterior arch are present after the seventh fetal week, but the anterior arch may not ossify until after birth. The posterior synchondrosis between the two halves of the neural arch may fail to fuse and may even be bifid. This common variation has obvious implications for surgical dissection. The canal of the atlas is exceptionally large to accommodate the extensive physiologic degree of
rotation that occurs at this joint as well as some forward translation (12). The vertebral arteries run horizontally in a groove on the rostral surface of the atlas, about 2 cm from the midline.
rotation that occurs at this joint as well as some forward translation (12). The vertebral arteries run horizontally in a groove on the rostral surface of the atlas, about 2 cm from the midline.
![]() Figure 33.3. Radiographic appearance of growth centers (arrows) of upper cervical vertebrae. A: Atlas. B: Axis. |
The axis normally develops from at least four ossification centers: one for the dens, one for the body, and two for the neural arches (Figs. 33.3B and 33.4). A small secondary ossification center also contributes to development of the cranial tip of the odontoid. The synchondrosis between the dens and body (dentocentral or basilar) is often referred to as a physis, but it closes early, between the ages of 3 and 6 years. It is recessed below the superior articular facets of the axis. Small, secondary centers of ossification also develop in the tips of the spinous processes.
The interval between the dens and the posterior cortex of the arch of the atlas is termed the atlantodens interval. It may be normally up to 4.5 mm in children, compared with 3 mm in adults (Fig. 33.5). This difference appears to result from unossified cartilage in these structures, as well as greater physiologic ligamentous laxity.
The bodies of the vertebrae below C2 are normally wedge shaped until about age 8 to 10 years. Apophyseal rings (secondary ossification centers) develop on the superior and inferior surfaces at age 10 to 12 years and fuse by maturity. Displacement of these ring ossification centers beyond the confines of the vertebral body may be a sign of physeal injury (13).
PHYSICAL EXAMINATION ANDACUTE CARE
Neck injuries in children are usually the result of major trauma. Head or facial trauma may be a clue to the presence of cervical spine fractures. The unconscious patient should be treated presumptively for a cervical spine fracture. Tenderness is a highly suggestive but not completely sensitive marker of cervical spine injury. The whole neck should be examined, as a patient may have more than one level of neck injury (5). A pediatric victim of major trauma should be protected from extreme flexion until tenderness of the cervical spine can be ruled out. This is important because some injuries in children are not readily detected by plain radiographs (14, 15 and 16) such as SCIWORA and transverse ligament injury of the axis. The screening neurologic examination should include, at a minimum, testing of active flexion-extension at all major joints, as well as sensation in all extremities.
RADIOGRAPHIC EXAMINATION
Computed tomography (CT) has largely replaced conventional radiography as the dominant screening radiographic examination in pediatric patients with suspected cervical spine injury (17). The reason for this evolution is most likely a result of the adult literature, where several prospective and retrospective studies have demonstrated a significant decrease in the rate of missed cervical spine injuries when CT of the cervical spine is performed. More recently, a retrospective study in children has demonstrated a significantly lower sensitivity of plain radiographs compared to CT scan (61% vs. 100%) (17).
Although CT scanning has the advantage of having a very high sensitivity of detecting cervical spine trauma, it is also associated with significant radiation exposure and increased cost compared to plain radiographs. Controversy continues to exist on how best to balance this risk-benefit scenario. Clinical guidelines were initially developed in adults and subsequently applied to the pediatric population. However, the relative infrequency of pediatric cervical spine trauma has hampered abilities to validate a pediatric-specific cervical spine clinical decision-making guideline (18).
At present, there is no clear evidence on which to base a recommendation for the optimal imaging strategy to detect cervical spine injury in children. Further clouding the situation is the high error rate of emergency room radiologist interpretation of plain radiographs and CT scans in children with cervical spine injury. Avellino et al. (19) found that 24% of children aged 8 and younger with suspected injury had an initial diagnostic error. The most common cited factors for misdiagnosis were unfamiliarity with pediatric cervical spine anatomy, failure to recognize normal variants seen during growth and development, and suboptimal conventional film techniques.
This finding underscores the importance of the clinician’s understanding of patterns of injury and developmental anatomy.
This finding underscores the importance of the clinician’s understanding of patterns of injury and developmental anatomy.
NORMAL VALUES
The occipitoatlantal interval is the most difficult to assess because of the lack of discrete, reproducible landmarks on the lateral plain films. The occipital condyles should rest in the depressions of the facets of the atlas. The interval between the condyles and facets should be less than 5 mm (20). The basion is the anterior cortical margin of the foramen magnum. The interval between it and the tip of the dens should be less than 10 mm (21,22). Powers’ ratio (Fig. 33.5) assesses the relative position of the skull base and the atlas: the distance BC (from the Basion to the anterior cortex of the C1 lamina) divided by the distance OA (from the Opisthion to the posterior cortex of the C1 Arch) should be less than 1. A greater value suggests an anterior dislocation of this joint, the usual type. Figure 33.5 shows the normal values for this and other levels of the pediatric cervical spine. Further helpful imaging of the atlanto-occipital interval may include magnetic resonance imaging (MRI), which can illustrate injury here by showing edema in the occipitocervical facet capsules and basocervical ligaments as well as an acute cervicomedullary angulation. A CT image can be used to show whether the occipital condyles are located in the C1 facets by superimposing them or obtaining a sagittal reconstruction. The anterior cortex of the cervical laminae should form a smooth line or curve (23), whether or not the cervical spine is lordotic. A substantial number of normal children do not have lordosis in the cervical spine (12).
The atlantodens interval is greater in children than adults. Up to 4.5 mm is considered normal (12,14,24, 25 and 26). This interval should be measured from the posteroinferior cortex of the body of the atlas along a line perpendicular to its posterior surface (21). In extension, the anterior arch of the atlas may slide up on the dens. The absence of soft tissue swelling does not rule out atlantoaxial instability.
The normal retropharyngeal soft tissue space in children is 6 mm or less at C3, and the retrotracheal space is less than 14 mm at C6 (12,20). The C2-C3 vertebrae may translate up to 3 mm in flexion (12). This phenomenon is termed pseudosubluxation. It can be confirmed as a normal variant by examining Swischuk’s line, which is drawn between the C1 and C3 laminae, and noting that the lamina of the atlas does not fall more than 1 mm forward of this line.
Children may have normal spine radiographs and CT scans but also have an acute spinal cord injury resulting in some degree of motor, sensory, or combined deficit (27). This is the phenomenon of SCIWORA, which was brought to light by Pang and Wilberger (28). The incidence of SCIWORA is estimated to be between 19% and 34% of all spinal cord injuries and is more common in children under age 10 (29). The site of injury is typically in the cervical cord and felt to be secondary to forced excessive spinal movement. The use of MRI has considerably improved the ability to detect abnormalities in patients with SCIWORA. These findings can be divided into extraneural, involving the ring of supportive soft tissues around the cord, and neural, involving the cord. It is possible for there to be an obviously injured cord without injury to the surrounding bony and ligamentous elements. The pediatric spine is particularly prone to distraction and the cord could potentially have been injured by a degree of distraction that is tolerated by the bony and ligamentous elements. It also may represent an ischemic injury in some cases. Consequently, MRI has not been useful in
determining stability of the spine in these cases. The ideal algorithm for evaluating these patients is still evolving (30,31). They should be protected with a cervical collar as well as by avoidance of extreme flexion, extension, or rotation, until cleared. SCIWORA is discussed further in Chapter 34.
determining stability of the spine in these cases. The ideal algorithm for evaluating these patients is still evolving (30,31). They should be protected with a cervical collar as well as by avoidance of extreme flexion, extension, or rotation, until cleared. SCIWORA is discussed further in Chapter 34.
TREATMENT OF SPECIFIC INJURIES
ATLANTO-OCCIPITAL INSTABILITY
Traumatic atlanto-occipital dislocation is one of the most frequently fatal injuries of the cervical spine. It occurs twice as frequently in children as in adults, (31) presumably because of the relatively large size of the child’s cranium. The mechanism of injury is believed to be one of hyperextension in most cases, although lateral rotation or even forward flexion also may be involved.
Children with traumatic atlanto-occipital instability and dislocation have a wide spectrum of symptomatology. Twenty percent of patients with atlanto-occipital instability are free of major neurologic injury, although some may experience cranial nerve injury, vomiting, headache, torticollis, or minor weakness (21,32, 33, 34, 35 and 36). Some symptoms of brainstem injury may result from vertebrobasilar vascular insufficiency. Dislocation at this level can present with full cardiorespiratory arrest secondary to injury of the respiratory centers of the brainstem, C3-C5. Other patients may have an asymmetric partial quadriparesis or a high cervical Brown-Sequard syndrome. However, the diagnosis may be very subtle, and there are case reports of patients whose diagnoses were not made for weeks after injury (37). The diagnosis of atlanto-occipital instability may be masked or overlooked in patients with concomitant brain injury. The clinician should be alert for clues to cervical cord injury in the presence of brain injury. These include spinal shock and urinary retention in cases of complete cervical lesions, as well as neck pain, diffuse tingling, or spasticity out of proportion to cognitive impairment in partial lesions (22).
Radiographic features hinge on demonstrating displacement (usually anterior) of the base of the skull on the atlas, which may include displacement of the condyles on the facets of the atlas, a Power ratio greater than 1, and an increased dens-basion interval greater than 1 cm (21,38) (Fig. 33.6). Recently, Pang et al. (39,40) have described the use of the condyle-C1 interval (CCI) to aid in the diagnosis of atlanto-occipital dislocation. The CCI is obtained from reformatted CT scans in the coronal or sagittal planes and represents the distance between the articulating surfaces of the occiput-C1 joint. Using a 4 mm cutoff, the CCI criterion was found to be 100% sensitive.
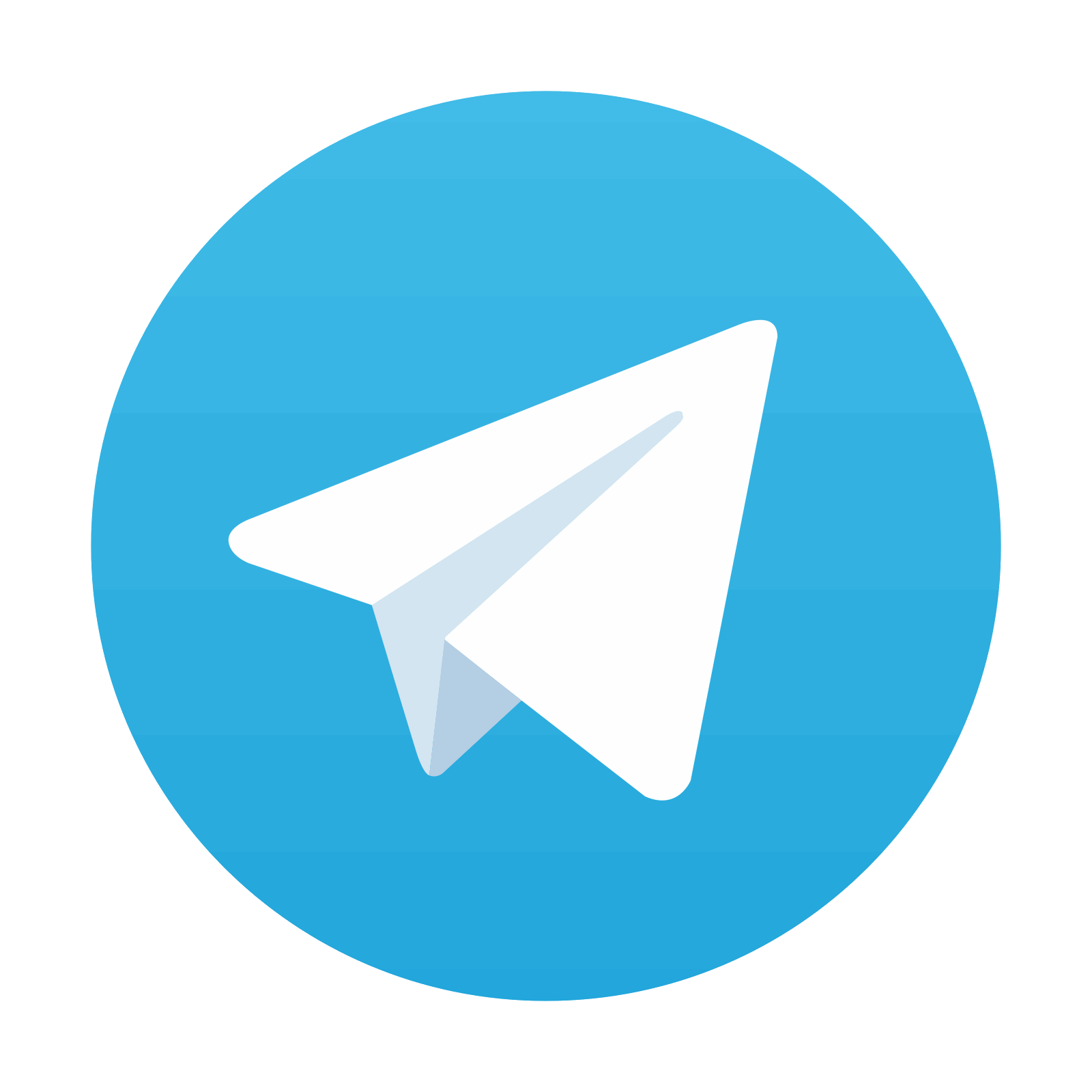
Stay updated, free articles. Join our Telegram channel

Full access? Get Clinical Tree
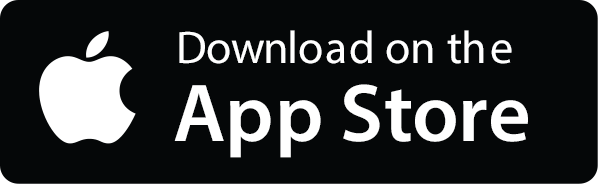
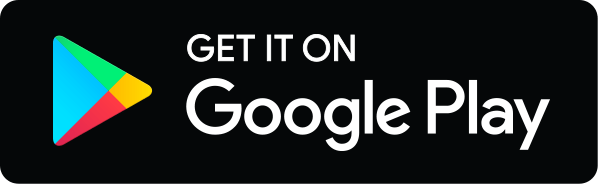