Anatomy is for physiology what geography is for history: it describes the scene of action.
2.1 Developmental Aspects
The understanding of some evolutionary and embryological key features of central nervous system development can be very helpful, particularly for the comprehension of the final placements, shapes, and relationships of the cerebral hemispheric structures.
To some extent, the embryological development of the human central nervous system (CNS) mirrors the main changes that occurred during phylogeny (Sarnat and Netsky, 1981; Gould, 1977), and with regard to the morphological aspects of the CNS, bending and folding mechanisms predominate throughout both developments to yield an overall CNS enlargement without a proportional increase in its volume. The bending process of each developing cerebral hemisphere takes place around its center which corresponds to each thalamus, and this is responsible for the final C-shaped profile assumed by each hemisphere and by many of its inner structures. A similar folding process acting on its surfaces gives rise to the sulci, which delimit the cerebral convolutions, or gyri, significantly enlarging its cortical area.
Another important issue for the understanding of the complex anatomy of the human brain is to bear in mind that our whole body is composed of parts with roles that have changed since they first evolved, and that our nervous system in particular harbors many vestigial structures that were important for our ancestors but are currently less or even not functional for humans, with the olfactory structures probably representing the best example of this (Held, 2009; Gould, 1977; Sarnat and Netsky, 1981). The anterior commissure, which was the main commissure in ancient reptiles, now carries about 5 percent of all commissural fibers in the monkey (mainly connecting corresponding parts of the temporal lobes), and in humans only carries 1 to 2 percent (Brodal, 2010) with a uncertain functional importance.
2.1.1 Evolutionary Considerations
The primitive fishes left the oceans about 350 million years ago becoming amphibians and then reptiles (Gould, 2001; MacLean, 1973). According to evolutionary theory, among all of the tremendous body transformations they were submitted to, their primitive CNS, which was then characterized by a spinal cord segment with incipient brainstem, hypothalamus, and striatum on top, gained the olfactory lobes to perceive their new world and the hippocampus and amygdala, respectively, to identify their new perceptions and to direct their behavior in conjunction with the hypothalamus (MacLean, 1973; Sarnat and Netsky, 1981; Squire et al., 2003; Oró, 2004; Park et al., 2007). These new structures were arranged around the top of the ancient CNS and were called limbic structures, from limbus the Latin word for “ring.” In order to perform different input and output combinations, their cells were arranged in a laminar pattern characterizing then the most primitive cortices, called the archicortex (amygdala and hippocampus) and paleocortex (olfactory piriform area) (Sarnat and Netsky, 1981) (Figure 2.1).
Figure 2.1 Evolutionary development of the neural structures.
Regarding the cerebral hemispheres, and particularly their cortical surfaces, their ultimate development which began with these primitive structures came with the development of the neocortex. This started with the advent of the mammals about 230 million years ago, and proceeded particularly throughout the evolution of the primates, culminating with the emergence of modern man about 50 000 years ago (Gould, 2001, 2002).
According to Sarnat and Netsky (1981), cytoarchitectonic evidence suggests that the generalized six-layered neocortex of primitive mammals evolved simultaneously from both the hippocampal archicortex and from the olfactory piriform paleocortex. As the first stage, with the archicortex forming the medial side and the paleocortex forming the lateral side of the cerebral hemisphere, both probably gave rise to a cortex with an architecture intermediate in complexity between three and six layers that surrounded the original primitive cortex. A second zone of further differentiated neocortex then formed as an additional concentric ring becoming the parahippocampal and the cingulate gyri on the medial side, and the insular cortex laterally. A third ring of further differentiated neocortex then appeared characterizing the paralimbic and parainsular cortices which became sites of specialized sensory and motor functions, with part of the parainsular region also becoming an auditory center and with a cortical visual center being developed in the paralimbic zone (Table 2.1).
Table 2.1 Mammalian cortical development
Primitive Cortex Allocortex (3 layers) | Medial Cortical Ring Mesocortex (6 layers) | Lateral Cortical Ring Isocortex (6 organized layers) |
---|---|---|
Limbic structures | Paralimbic structures and insula | Parainsular structures and neocortical structures |
• Archicortex: | • Neocortex: | |
Amygdala | Parahippocampal gyrus | Motor cortex |
Hippocampus | Cingulate gyrus | Sensory cortex |
• Paleocortex: | Visual cortex | |
Olfactory or | Insula | Auditory cortex |
Piriform cortex | Language cortices (humans) | |
Rest of neocortex, associated areas |
The final shape and configuration of the human cerebral hemisphere itself is mostly given by the bending mechanism that takes place around its morphological center composed of its respective thalamus, throughout both evolution and human embryology (Figure 2.2).
Figure 2.2 (A) evolutionary and (B) human embryological and fetal developments of the central nervous system.
In lower terrestrial vertebrates, the hippocampus develops as a dorsal structure forming the medial part of the cerebral hemispheres, and in advanced mammals, it is rotated back and down into a ventral position by the great expansion of the neocortex (Romer, 1970 apud Sarnat and Netsky, 1981). Further development of the parahippocampal gyrus along the hippocampus and of its continuous cingulate gyrus comprising the cortical initial inner medial ring, and of the paralimbic, parainsular, and the rest of the neocortex then occurred progressively and along the circular route generated by bending of the whole cerebral hemisphere around the thalamus.
The tremendous development of the mammalian cortex occurred throughout an extensive infolding process that made possible a significant increase in its surface without a proportional enlargement of its outer extent and total volume, and resulted in the final cortical human pattern given by its fissures, sulci, and their delimitated gyral convolutions. Considering also the interhemispheric fissure, this invagination process led by the evolutionary forces finally left in man approximately two-thirds of the cortical surface buried in the depths of the sulci and fissures (Williams and Warwick, 1980).
The first hemispheric sulcus to appear phylogenetically is the sulcus that separates the archicortex from its surrounding structures (namely the hippocampal sulcus that separates the dentate gyri of the hippocampus from the parahippocampal subiculum, and that arises medially along the phylogenetic and embryological caudal migration of the previous supracallosal hippocampus) (Sarnat and Netsky, 1981). The second is the sulcus that demarcates the paleocortex from the neocortex (namely the rhinal sulcus, secondary to the ventral displacement of the piriform cortex caused by neocortical development (Sarnat and Netsky, 1981), and that in man separates the parahippocampal uncus from the rest of the neocortical temporal pole). Both sulci were already present in early mammals (Sarnat and Netsky, 1981).
On the other hand, the Sylvian fissure was a result of the overlapping growth of the infolding opercula of the surrounding lobes onto the insula (Ribas, 2010; Sarnat and Netsky, 1981), and which became narrow only in man with the particular development of the frontoparietal operculum that is underdeveloped even in the highest anthropoid apes (Sarnat and Netsky, 1981; Squire et al., 2003). The most notable development was the pars triangularis and the pars opercularis of the inferior frontal gyrus that in the dominant hemisphere of man correspond to the so-called Broca’s speech area (Broca, 1876b).
2.1.2 Embryological and Fetal Considerations
The embryological development of the nervous system begins around the third week of intrauterine life with thickening of the ectoderm which generates the neural plate under the inducing influence of the notochord. The development of neural folds with a neural groove between them starts the formation of the neural tube, with the so-called neurulation proceeding with the cranial to caudal approximation and fusion of the neural folds along the midline, and with the subsequent closures of the cranial and posterior neuropores around the end the fourth week.
While the neural tube itself sinks from the surface of the ectoderm to generate the CNS, the remaining pluripotent cells of the neural crest migrate to form sensory and autonomic ganglia, Schwann cells, adrenal medulla, the pia matter, and the arachnoid among other structures (Borden et al., 2016).
While the mantle layer of the neural tube forms the gray matter, its marginal layer gives rise to the white matter, and its lumen the ventricular cavities, the aqueduct, and the central canal of the spinal cord.
The spinal cord and caudal portion of the brainstem develop according to a straightforward organization, with the mantle layer of the spinal cord developing in each side into a basal plate (future anterior horn) and an alar plate (future posterior horn). These become separated by an evident groove known as the sulcus limitans, and with the motor and sensory cranial nerves of the medulla oblongata and of the pons having a medial to lateral arrangement; however, this organization is not observed from the midbrain up.
The more pronounced and complex development of the cranial portion of the neural tube has its final architecture determined by the development of the brain vesicles (three primary and five secondary vesicles) (Figure 2.3) and by the cranial folding of the neural tube (cervical, mesencephalic, and pontine) (Borden et al., 2016) (Figure 2.4).
Figure 2.3 Embryological development of the neural tube and brain vesicles.
III: third ventricle; IV: fourth ventricle; LV: lateral ventricle; Aq: aqueduct.
Figure 2.4 Embryological and fetal development of the ventricular system.
III: third ventricle; IV: fourth ventricle; Aq: aqueduct; Lv: lateral ventricle; Me: mesencephalon; Pr: proencephalon; Rh: rhombencephalon.
Very early during embryogenesis, the prosencephalic vesicle (forebrain), the most superior of the three primary brain vesicles originating from the neural tube, is divided cranial-caudally by the development of the transverse fissure of the brain forming the endbrain (telencephalic) and the interbrain (diencephalic) vesicles. A superior midline depression of the endbrain starts forming the interhemispheric fissure giving rise to both cerebral hemispheres. While the cerebral structures are developed from the walls of the endbrain vesicle, its residual central lumen becomes the lateral ventricles.
The superior midline depression of the endbrain, which originally corresponds to the top of the neural tube, as well as forming the interhemispheric fissure, is also displaced anteriorly and inferiorly ending up as the anterior wall of the midline interbrain vesicle that corresponds to the inferior part of the original prosencephalic vesicle. The diencephalic structures (thalamus, hypothalamus, subthalamus, and epithalamus) originate from its walls on each side, and its residual lumen becomes the third ventricle in between both thalami and with its anterior wall then having a telencephalic origin and being very appropriately called the lamina terminalis due to its original position.
Subsequently, the circular bending of the upper part of the neural tube around the thalami leads the developing telencephalic structures and the lateral ventricular cavities to assume C-shaped profiles (Figure 2.4).
Each thalamus ends up intimately connected and continuous with the midbrain, morphologically like a head placed on top of each upper half of the brainstem, at the center of the brain (Figure 2.5).
Figure 2.5 The ventricular system and the thalamus.
Th: thalamus; LV: lateral ventricle; III: third ventricle.
Each hippocampus, which initially occupies a superior and medial position, slides posteriorly and inferiorly around the thalamus ending within the inferior (temporal) horn of the lateral ventricle, leaving a tail of fibers along its course which constitute the fornix. The virtual space between each fornix and each thalamus becomes the choroidal fissure. The two small longitudinal striae of gray matter that end over the corpus callosum, which are known as the indusium griseum (supracallosal gyri), are believed to be remnants of both ancient hippocampi (Sarnat and Netsky, 1981) (Figure 2.6).
Figure 2.6 Migration of ancient hippocampus and human hippocampal formation structures.
Hipp: hippocampus; Fo: fornix.
Concomitantly, the callosal fibers develop over the top of the lateral ventricles from anterior to posterior, and the projection fibers, which connect the cortex with subcortical structures, develop splitting the previously formed corpus striatum on both sides.
Since the cranial-caudal projection fibers in each hemisphere assume a final fan-like shape, opening toward the cortical surface and converging toward the thalamus and brainstem, the dorsal aspect of each corpus striatum is divided generating the caudate nucleus medially and the putamen laterally. The ventral aspect of the striatum remains undivided still connecting the anterior and basal aspects of the caudate and putamen with the ventral striatum harboring the nucleus accumbens (Figure 2.7). Usually small residual nests of striatal cells can be found within the ascending and descending projection fibers, particularly within the most anterior groups of fibers (anterior limb of the internal capsule) where division of the corpus striatum can frequently be incomplete.
Figure 2.7 Embryological and fetal development of the caudate and putamen.
Lv: lateral ventricle; Str: striatum; CaN: caudate nucleus; Put: putamen; PrFi: projection fibers.
The globus pallidus has a different embryological origin but lies attached to the inferior and medial aspect of the putamen with both the globus pallidus and putamen constituting the lentiform nucleus. The projection fibers within its superior and inferior levels are referred to as the internal capsule of the lentiform nucleus.
The same evolutionary and embryological bending mechanisms also affect other deep structures such as the stria terminalis (which is a dorsal extension of the amygdala) ending up with these structures also encircling each thalamus in a C-shaped configuration, just like the lateral ventricle and the outer aspects of the cerebral hemisphere itself. The deeper structures in part become the walls of the lateral ventricles as discussed later, with each fornix wrapping around each thalamus medially and the caudate nucleus wrapping around each thalamus laterally.
Both the transverse fissure of the brain, which separates the endbrain (telencephalic) from the interbrain (diencephalic) vesicles, and the interhemispheric fissure, which starts as a superior midline depression dividing the endbrain vesicle, arise around the tenth week of gestation (Chi et al., 1977).
Regarding the cerebral surface, a similar folding process gives rise to the fissures and sulci that delimit the cerebral convolutions or gyri, and this process significantly enlarges its cortical area without a proportional increase in brain volume.
Embryologically, the sulci are formed according to a sequence which reflects their phylogeny and a true hierarchy exists among them. Their formation begins with the appearance of the fissures, followed by the sulci related to eloquent areas of the brain, and finally by sulci of the secondary and tertiary cortical areas (Broca, 1876a apud Stone, 1991; Broca, 1877 apud Finger, 1994; Chi et al., 1977; Nishikuni and Ribas, 2013) (Table 2.2) (Figure 2.8).
Table 2.2 Prenatal cerebral sulci development in weeks of gestation
Chi et al., 1977 | Nishikuni, 2006 | |
---|---|---|
No. of fetuses | 207 | 107 |
Gestational age range, weeks | 10–44 | 12–40 |
Longitudinal cerebral fissure | 10 | 12 |
Superolateral surface | ||
Lateral sulcus | 14 | 17 |
Circular insular sulcus | 18 | 17 |
Central insular sulcus | 29 | |
Central sulcus | 20 | 21 |
Precentral sulcus | 24 | 26 |
Superior frontal sulcus | 25 | 25 |
Inferior frontal sulcus | 28 | 30 |
Postcentral sulcus | 25 | 29 |
Intraparietal sulcus | 26 | 29 |
Transverse occipital sulcus | 30 | |
Lunate sulcus | 24 | |
Superior temporal sulcus | 23 | 26 |
Inferior temporal sulcus | 30 | 31 |
Transverse temporal sulcus | 31 | 33 |
Inferior surface | ||
Olfactory sulcus | 16 | 17 |
Orbital sulcus | 22 | |
Hippocampal sulcus | 10 | 12 |
Rhinal sulcus | 25 | |
Collateral sulcus | 23 | 29 |
Occipitotemporal sulcus | 30 | 33 |
Medial surface | ||
Callosal sulcus | 14 | 12 |
Cingulate sulcus | 18 | 19 |
Marginal ramus | 33 | |
Paracentral sulcus | 30 | |
Paraolfactory sulcus | 29 | |
Subparietal sulcus | 30 | |
Calcarine sulcus | 16 | 17 |
Parieto-occipital sulcus | 16 | 19 |
Secondary sulcus | 40 | 38 |
Figure 2.8 Development of sulci in the superolateral cerebral surface of the fetus at (A) 17, (B) 24, and (C) 36 weeks, and (D) at 1 postnatal week.
CS: central sulcus; IFS: inferior frontal sulcus; IHF: interhemispheric fissure (longitudinal cerebral fissure); IPS: intraparietal sulcus; ITS: inferior temporal sulcus; OrbS: orbital sulcus; OTS: occipitotemporal sulcus; PostCS: postcentral sulcus; PreCS: precentral sulcus; SFS: superior frontal sulcus; STS: superior temporal sulcus; SyF: lateral sulcus (Sylvian fissure); TrOS: transverse occipital sulcus.
Between the eighth and tenth weeks, transitory furrows, which are not precursors of the definite sulci, appear in the cerebral hemispheric surfaces and last until the fifth month when the brain surfaces become smooth with only the insular area present as an evident depression (Ono et al., 1990).
During the fourth to fifth months of fetal life, the first definite sulci (olfactory, calcarine, parieto-occipital, cingulate, and central) begin to appear initially as points or grooves, followed by further secondary and tertiary furrows, with some of these being developed only after birth (Chi et al., 1977; Ono et al., 1990; Nishikuni and Ribas, 2013).
Control of this process and of its relatively variable final result are to a large part genetically determined (Squire et al., 2003); however in practical terms, it is interesting to point out that, given the mechanism of infolding in which the sulci are developed and given the concomitant circular curvature that the whole developing brain is submitted to such as wrapping the thalami at its morphological center, the sulci, particularly of the superolateral and inferior surfaces of the cerebral hemisphere, end up pointing toward the nearest part of the lateral ventricular cavity. The development of the sulcal pattern in the medial surfaces seems to be particularly influenced by the development of the corpus callosum since its congenital absence is linked to the absence of an arched cingulate gyrus and to the radial pattern of the sulci in the medial surfaces (Ono et al., 1990).
Regarding the extensive variability of the basic arrangement of the cortical sulci and gyri, Régis et al. (2005) recently proposed that the occurrence of such variations might depend on the variable development of connecting gyri buried at depth in the sulci (“plis de passage”). Taking into account these buried gyri, and using a database of MR images from 20 healthy patients, these authors proposed an interesting generic model of folding patterns based on a constant number of indivisible units they termed “sulcal roots,” and proposed a common constant protomap. According to this hypothesis, the burying process is believed to result from a trade-off between the various folding pressures that occur during brain growth, with the superficial variability resulting from the chaotic behavior given by the greater or lesser development of the buried gyral connections, with a major development of a given gyral connection causing an interruption to the sulcus located above this connection. As an example based on this hypothesis, the occasional interruption of the central sulcus is then due to a more significant development of the middle frontoparietal connection (of Broca) that lies between the precentral and postcentral gyri at the so-called omega region (Boling and Olivier, 2004; Yousry et al., 1997).
The telencephalic insula, basal ganglia (lentiform and caudate nuclei) and its surrounding fibers (internal and external capsules of the lentiform nucleus), together with the diencephalic thalamus itself, morphologically comprise a rather well-defined anatomical block within each hemisphere, delimited medially by the lateral and third ventricular cavities. As discussed in more detail later, this block is referred to as the central core of the brain.
2.2 The Cerebral Hemispheres
The two cerebral hemispheres together constitute the most developed part of the human nervous system (Ribas, 2015), and each corresponds to a large mass of neuronal tissue with a C-shaped format which medially wraps the ipsilateral thalamus with the lateral ventricle in between (Figure 2.9).
Figure 2.9 (A and B): The margins of the cerebral hemispheres.
a: Superomedial; b: inferolateral – b1: superciliary, b2.1: inferolateral-sphenoidal part, b2.2: inferolateral – basal temporal part; c: medial orbital; d1: medial perimesencephalic, d2: medial occipital; Th: thalamus.
Each cerebral hemisphere has the cerebral cortical mantle as its surface, anteriorly it is characterized by the frontal and temporal poles, and posteriorly by the occipital poles. It harbors the basal ganglia (caudate nucleus, putamen, nucleus accumbens, globus pallidus amygdala, and claustrum) (Lockard, 1977), and the association, commissural, and projection fibers, with both hemispheres being connected mainly by the corpus callosum along the midline.
Each hemisphere has a superolateral surface (dorsal, cerebral convexity), a medial surface, and an inferior or basal surface, respectively separated by the superomedial, inferolateral (with its anterior part also known as superciliary), medial orbital, and medial occipital margins (Figure 2.9 A and B).
The superolateral or dorsal surface is concave and lies underneath the bones of the cranial vault, with the frontal, parietal, temporal, and occipital lobes approximately corresponding in surface extent to the overlying cranial bones from which they take their names. The frontal and parietal lobes are separated from the temporal lobe by the very evident lateral (Sylvian) sulcus.
The inferior or basal surface is divided by the anterior part of the lateral sulcus into a small anterior and a larger posterior part. The anterior part constitutes the orbital surface of the frontal lobe, and rests on the cribriform plate of the ethmoid, on the orbital plate of the frontal bone, and on the lesser wing of the sphenoid bone, which altogether constitute the floor of the anterior cranial fossa. The posterior part of the inferior surface is larger, composed of the basal aspects of the temporal and occipital lobes, and rests on the floor of the middle cranial fossa and on the posteriorly continuous upper face of the tentorium cerebri, which is a dural fold that covers the cerebellum.
The medial cerebral surface is flat and lies within the great longitudinal fissure, limited inferiorly by the commissural fibers of the corpus callosum which lie in the depths of the fissure. The surface is separated from the medial surface of the opposite hemisphere by a crescent-shaped fold of the dura mater, the falx cerebri.
The cerebral surface is excavated by the sulci which roughly separate the gyri, with the more pronounced and well-defined sulci generically referred to as fissures (Gusmão et al., 2000; Gratiolet, 1854 apud Pearce, 2006).
2.2.1 The Meninges, the Subarachnoid Space, and the Main Cerebral Fissures
The CNS is surrounded externally by the three meninges: the pia mater, the arachnoid, and the dura mater.
The pia mater is firmly attached to the surface of the CNS along its whole extent, depressions, and recesses, and is responsible for the consistency and relative endurance of the CNS surface. The arachnoid completely covers the CNS as a tight envelope, harboring the subarachnoid space and with the cerebrospinal fluid (CSF) running underneath the arachnoid. Delicate trabeculae of the arachnoid stand out and bind to the pia mater, thus justifying its name.
The dura mater is the most superficial of the meninges, being the thickest and the only one that contains blood vessels and nerves. While the dura that covers the brain is formed by two leaflets, the spinal cord dura is composed of only one leaflet. The falx and the tentorium are thick dural folds. The falx separates the two cerebral hemispheres and the tentorium supports both temporo-occipital surfaces. The subdural space between the dura and the arachnoid is a virtual space throughout its whole extent.
On the superolateral surface of the brain, the subarachnoid space is very shallow over the crest of the gyri, and extends to the intrasulcal spaces. Along the lateral (Sylvian) fissure, it gradually expands toward the cerebral base giving rise to the subarachnoid cisterns (Key and Reteius, 1875 apud Yasargil, 1984a; Matsuno et al., 1988; Yasargil et al., 1976). The basal subarachnoid cisterns (Table 2.3) are CSF compartments that are anatomically relatively distinct, are separated by porous trabeculated walls, and harbor the basal vessels and cranial nerves (Yasargil, 1984a; Yasargil et al., 1976). While the arteries lie loosely within the cisterns, attached only to the arachnoid trabeculae, the veins are always firmly adhering to the pial surface of the brain.
Table 2.3 The supratentorial subarachnoid cisterns
Anterior (parasellar) | |
1) Carotid cistern | |
2) Chiasmatic cistern | |
3) Lamina terminalis cistern | |
4) Olfactory cistern | |
5) Sylvian cistern | |
Lateral (parapeduncular) | |
1) Crural cistern | |
2) Ambient cistern | |
Posterior (tentorial notch) | |
1) Quadrigeminal cistern | |
2) Velum interpositum cistern | |
Superior (callosal) | |
1) Corpus callosum cistern – anterior portion | |
2) Corpus callosum cistern – posterior portion | |
3) Interhemispheric cistern |
Given the anatomy of the arachnoid and of the pia, the brain sulci correspond to extensions of the superolateral subarachnoid space, and the brain fissures harbor the basal subarachnoid cisterns.
There are three major cerebral fissures which are particularly characteristic of each hemispheric brain surface, and which define much of the brain architecture: the lateral or Sylvian fissure along the superolateral surface, the interhemispheric or longitudinal fissure along the medial surface, and the transverse fissure of Bichat (Bouchet et al., 1966; Testut and Jacob, 1932a; Yasargil, 1987) within the cerebral basal surface (Figure 2.10). These three fissures constitute well-defined and anatomically constant natural spaces, and they harbor the main supratentorial subarachnoid cisterns (Ono et al., 1990; Yasargil, 1984a; Yasargil et al., 1976; Yasargil et al., 2002a) (Figure 2.10).
Figure 2.10 The concept of three main cerebral fissures and the cisterns which they harbor. Th: thalamus.
The lateral (or Sylvian) fissure is classically divided into an anterior part (also known as the sphenoidal part and as the stem of the Sylvian fissure) and into a lateral or posterior part (Ono et al., 1990; Yasargil, 1984a; Yasargil et al., 1976; Yasargil et al., 2002a). The division occurs at the anterior Sylvian point, which corresponds to a usually evident enlargement of the fissure at the bottom of the triangular part of the inferior frontal gyrus (Ribas et al., 2005a).
The anterior part of the Sylvian fissure lies anteriorly to the lateral aspect of the anterior perforated substance, ends at the level of the limen insulae, and harbors the most basal part of the Sylvian cistern with the initial segment of the middle cerebral artery (M1 segment) and with its temporal and perforating branches (Gibo et al., 1981a; Gibo et al., 1981b; Yasargil, 1984a).
The lateral or posterior part runs obliquely along the superolateral brain surface separating the surfaces of the frontal and temporal lobes, usually underneath the superficial Sylvian vein. It is composed of a thin superficial part located between the frontoparietal and the temporal opercular surfaces, and by a deep part that corresponds to a real fossa over the insular surface.
Sequentially along the superior aspect of the extent of the Sylvian point, first the horizontal and anterior ascending branches that delimit the triangular part of the inferior frontal gyrus, and then the anterior and posterior subcentral branches that delimit the subcentral gyrus originate from the anterior Sylvian point. The posterior ascending branch which ends inside the supramarginal gyrus, and, occasionally, a distal descending branch that penetrates inside the superior temporal gyrus originate from the posterior Sylvian point (Ono et al., 1990; Ribas et al., 2005a; Yasargil, 1984a; Yasargil, 1984b; Yasargil and Abdulrauf, 2003)(Figure 2.13 and Figure 3.9). Eventually, the most inferior segments of the precentral, central, and postcentral sulci can reach the lateral (Sylvian) fissure, but they cannot be considered Sylvian branches since these sulci always end inside U-shaped convolutions which may eventually lie inside the lateral (Sylvian) fissure (Ribas et al., 2005a).
The lateral or posterior part of the Sylvian fissure harbors the largest part of the Sylvian cistern, with the superior and inferior branches of the middle cerebral artery at its base (M2 segments), and with its frontoparietal and temporal branches (M3 segments) looping around the respective opercula toward the brain surface. The most distal branches of the middle cerebral artery displayed on the frontoparietal and temporal superolateral surfaces (M4 segments) are already external to the lateral (Sylvian) fissure (Gibo et al., 1981a; Yasargil, 1984a; Yasargil et al., 2002a).
The interhemispheric or longitudinal fissure separates the medial surfaces of both cerebral hemispheres around and superiorly to the corpus callosum, and is divided longitudinally by the falx cerebri. Since the falx does not reach the superior surface of the corpus callosum, both cingulate gyri are usually attached along the midline. The interhemispheric or longitudinal fissure contains the callosal and the interhemispheric cisterns, which harbor the pericallosal and the callosomarginal arteries, and the distal branches of both anterior cerebral arteries (A2, A3, A4, and A5 segments of the anterior cerebral arteries) (Perlmutter and Rhoton, 1978; Rhoton, 2003).
The transverse fissure of Bichat (Bouchet et al., 1966; Testut and Jacob, 1932a; Yasargil, 1987) is located around the inner basal aspects of both cerebral hemispheres, resembling two horseshoes with anterior concavities and with a common median part. While its lateral limbs lie along each side of the tentorial incisural region (Testut and Jacob, 1932a; Yasargil, 1987), its superior, median limb lies superiorly within the roof of the third ventricle (Testut and Jacob, 1932a; Yasargil, 1987; Williams and Warwick, 1980).
Each lateral limb of the transverse fissure is delimited medially by the cerebral peduncle, superiorly and anteriorly by the optic tract, superiorly and more posteriorly by the pulvinar of each thalamus (lateral and medial geniculate bodies), and inferiorly by the superior surface of each parahippocampal gyrus (the subiculum). Its most anterior aspect corresponds to the anterior perforated substance space from which it is continuous with the lateral (Sylvian) fissure, and its most posterior aspect corresponds to the subsplenial space that overlies the quadrigeminal plate, from where it is anteriorly continuous with its median limb along the roof of the third ventricle (Testut and Jacob, 1932a; Yamamoto et al., 1981; Yasargil, 1987; Yasargil, 1994).
Each lateral limb of the transverse fissure harbors anteriorly and superiorly the crural cistern between the cerebral peduncle and the uncus, and this cistern contains the anterior choroidal artery (Fujii et al., 1980; Rhoton et al., 1979; Yasargil, 1987; Yasargil et al., 1976). More inferiorly and posteriorly, between the cerebral peduncle and the subiculum, each lateral limb of the transverse fissure also harbors the ambient cistern, which contains the dentate gyri, the fimbria, the posterior cerebral artery, and the basal vein of Rosenthal (Ono et al., 1984; Párraga et al., 2011; Testut and Jacob, 1932a; Yasargil, 1984a; Yasargil, 1987). Posteriorly, both ambient cisterns are continuous with the quadrigeminal or pineal cistern.
The median limb of the transverse fissure lies below the splenium and above the superomedial surfaces of both thalami, and between the superior and inferior layers of the tela choroidea that run within the roof of the third ventricle. Whereas the superior layer of the tela choroidea is attached along and underneath both fornices and the hippocampal commissure, the inferior layer is attached along both thalamic striae and the superior surface of the pineal body (Testut and Jacob, 1932a; Williams and Warwick, 1980; Yamamoto et al., 1981; Yasargil, 1987). The median limb of the transverse fissure harbors the cistern of the velum interpositum which extends from the quadrigeminal cistern as far as the posterior borders of both interventricular foramens (of Monro), and which contains both internal veins and the branches of both posteromedial choroidal arteries (Fujii et al., 1980; Yamamoto et al., 1981; Yasargil, 1987).
Since both the transverse fissure and the choroidal fissure encircle each thalamus, they are parallel and intimately related, being continuous throughout their whole extent. Nevertheless, whereas each transverse fissure is a broader space than the choroidal fissure delimited by part of the surface of each thalamus and by the surfaces of the neural structures that encircle the thalamus, each choroidal fissure is a narrow cleft between each thalamus and each fornix (Nagata et al., 1988). The transverse fissure is continuous with the lateral ventricle along the choroidal fissure, which implies that any opening of the choroidal fissure from the lateral ventricle leads to the transverse fissure and to its subarachnoid cisterns. The opening of the choroidal fissure from the temporal horn will lead to the ambient cistern, from the atrium will lead to the quadrigeminal cistern, and from the body of the lateral ventricle will lead to the velum interpositum cistern within the roof of the third ventricle.
With regard to the other basal cisterns that are not contained within the three major brain fissures, anteriorly and inferiorly to the most anterior and basal aspect of the callosal cistern, there is the lamina terminalis cistern, which is anteriorly contiguous with the chiasmatic cistern. Inferiorly, the chiasmatic cistern is continuous with the interpeduncular cistern which has the Liliequist membrane as its anterior wall. More anteriorly, there are both olfactory cisterns. Lateral to these, there are on each side the parasellar carotid cistern which extends posteriorly to the parapeduncular crural and ambient cisterns. Laterally, these basal cisterns are contiguous with the most medial and inferior aspect of the Sylvian cistern (Yasargil, 1984a) (Figure 2.11).
Figure 2.11 The basal cisterns (Arabic numbers), the anterior and posterior arterial systems (in red), and the cranial nerves (Roman numerals). (Adapted from Yasargil (1984a).)
1: Olfactory cistern; 2a: Callosal cistern; 2b: Lamina terminalis cistern; 3: Chiasmatic cistern; 4: Carotid cistern; 5: Sylvian cistern; 6: Crural cistern; 7: Interpeduncular cistern; 8: Ambient cistern; 9: Prepontine cistern; 10: Superior cerebellar-pontine cistern; 11: Inferior cerebellar-pontine cistern (lateral cerebello-medullary); 12: Anterior spinal cistern; 13: Posterior spinal cistern.
2.2.2 The Cerebral Surface, Its Sulci and Gyri
The evolutionary invagination process that produced the convoluted form of the cerebral surface increased its extent threefold having generated a cortical area of approximately 2200 cm2 (von Economo and Koskinas, 1925 apud Williams and Warwick, 1980). Only one-third is exposed on its surface with two-thirds buried inside the intrasulcal spaces, and with a thickness that varies between 3 and 5 mm (Brodal, 2010; Standring, 2008).
Although still a subject of debate (Azevedo et al., 2009), it is believed that the human cerebral cortex harbors about 20 billion of the almost 100 billion neurons in the whole human brain, being also supported by up to 10 times as many neuroglial cells, and with each neuron being able to perform from some hundreds up to thousands of synapses. Within 1 mm2, the cerebral cortex harbors about 100 000 neurons (Brodal, 2010; Henneberg, 1910 apud Catani and Schotten, 2012).
According to its structural differences, the cerebral surface or pallium (cerebral cortex and its underlying white matter) (Lockard, 1977) can be divided into the archipallium which still has white matter as its outer surface (hippocampal formation) and neopallium which has gray matter as its outer surface (Meynert, 1885 apud Catani and Schotten, 2012). The term paleopallium is related only to the intermediary cortex of the pyriform area (uncus and adjacent part of the parahippocampal gyrus) (Lockard, 1977).
The cerebral surface is comprised of a small proportion of phylogenetically old allocortex (unlaminated or partly laminated cortex) of the archipallium and paleopallium, and a huge proportion of newer isocortex (well-defined six-layered cortex of the neopallium) (Lockard, 1977). The isocortex has different organizational arrangements of its layers throughout its whole extent, which are related to different functional roles (Ribas, 2015).
The cerebral cortex is activated through projections arising in the reticular formation of the brainstem and within the thalami, and is directly or indirectly connected to all the subcortical structures. The complex neural physiology provided by the different arrangement of cortical layers throughout the brain surface, and by the extensive network of white matter fibers (with each neuron being able to make thousands of synaptic contacts), influences the autonomic functions and generates the basic (sensory and motor) and the higher cortical functions (cognition, emotion and behavior). The interactions of all these functions give rise to the experience of consciousness, to the notion of one’s self, and to the tailoring of our personality.
According to Mesulam, anatomically there are five well-defined large-scale networks that are most relevant to clinical practice: a left-dominant perisylvian network for language; a right-dominant parietofrontal network for spatial cognition; an occipitotemporal network for face and object recognition; a limbic network for retentive memory; and a prefrontal network for attention and behavior (Catani et al., 2005; Mesulam, 1987; Mesulam, 1990; Mesulam, 2011; Papez, 1937).
Anatomically, given their phylogenetic (Butler and Hodos, 2005; Park et al., 2007; Sarnat and Netsky, 1981) and embryological (Chi et al., 1977; Nishikuni and Ribas, 2013; Ono et al., 1990; Williams and Warwick, 1980) development of folding, the cerebral sulci delineate the brain gyri and correspond to natural extensions of the subarachnoid space. When they are deep and anatomically more constant, they are also referred to generically as fissures (Broca, 1876b; Broca, 1861 apud Gusmão et al., 2000; Gusmão et al., 2000; Gratiolet, 1854 apud Pearce, 2006).
The main sulci have approximate depths ranging from 1 to 3 cm, and their walls harbor small gyri that face, adapt to, and connect with each other; those gyri are generically designated as transverse gyri. The sulci that separate the transverse intrasulcal gyri vary in length and depth, and, at the surface of the brain, they become visible as incisures. The indentations caused by cortical arteries can have an appearance similar to that of the incisures.
It is noteworthy that the timing of the embryological development of the sulci and their degree of variability (Chi et al., 1977; Nishikuni and Ribas, 2013) define a true morphological hierarchy, at the top of which are the fissures and main sulci (Table 2.2). It is equally notable that this structural hierarchy is directly correlated with the functional importance of the areas to which the sulci are related, with the more anatomically constant sulci being those that are topographically related to areas that are more specialized (Penfield and Baldwin, 1952 apud Hansebout, 1977; Uematsu et al., 1992).
On the brain surface, the sulci can be long or short as well as continuous (Sylvian fissure, callosal, calcarine, parieto-occipital, collateral, and generally the central sulcus)or interrupted. Ono et al. (1990) have described four main types of sulci: large primary sulci (e.g., central, precentral, postcentral, and continuous sulci); short primary sulci (e.g., rhinal, olfactory, lateral, and occipital sulci); short sulci composed of several branches (e.g., orbital and subparietal sulci); and short, free supplementary sulci (e.g., medial frontal and lunate sulci). Frequently, the sulci are composed of side branches that can be unconnected or connected (with end-to-side, end-to-end, or side-to-side connections that can also join two neighboring parallel sulci).
Due to their frequent variations and connections, the nomenclature of sulci varies among different authors according to their interpretations and designations (Duvernoy, 1991; Ono et al., 1990; Ribas, 2010; Testut and Jacob, 1932a). For a better understanding, it is important to emphasize that the sulci can vary in size and shape from person to person. The brain gyri constitute a real continuum that present as a serpentine configuration on the surface of the brain due to their connections along the sulcal extremities and interruptions, and have their continuity mainly along the sulci depths and intrasulcal multiple connecting extension arms (Yasargil, 1994). The gyral separation is then only superficial and each gyrus should be understood in reality as a region and not as a well-defined structure (Ribas, 2010).
For practical surgical purposes, it is also interesting to note that, due to their origin by a process of infolding, the sulci of the superolateral and the inferior surfaces of the brain are usually oriented toward the nearest ventricular cavity, which does not apply to the medial cerebral face where the sulci are predominantly secondary to the development of the corpus callosum (Ono et al., 1990).
Although appearing as having a labyrinthine disposition, the main cerebral sulci and gyri are arranged through a predominantly basic configuration, and their main points display fairly constant relationships with the cranial vault and with the deep neural structures (Ribas et al., 2006). According to their anatomical uniformity, and to their related parallel cortical functional importance, both the gyri and sulci can be categorized as primary, secondary, or tertiary. The gyri that are more rounded or quadrangular are usually referred to as lobules.
On the superolateral surface of the brain, the frontal and temporal regions of each hemisphere are each composed of three horizontal gyri (superior, middle and inferior frontal, and temporal gyri); the central area is composed of two slightly oblique gyri (pre- and postcentral gyri); the parietal region is composed of two semicircular lobules (superior and inferior parietal lobules, with the inferior lobule composed of the supramarginal and angular gyri); the occipital region is composed of two or three less well-defined gyri (superior, middle, and inferior occipital gyri); and the insula, which lies deep in the floor of the very evident lateral (Sylvian) fissure, is composed of four to five diagonal gyri (short and long insular gyri) (Figure 2.12.A).
Figure 2.12 Basic organization of the brain gyri. (A) Superolateral surface and (B) medial and basal surfaces. Red lines indicate the constant arrangement of the main brain gyri. (Adapted from Ribas (2010).)
Figure 2.13 The main brain sulci (A) and gyri (B) of the superolateral surface of the brain.
AG: angular gyrus; ASCR: anterior subcentral ramus of Sylvian fissure; CS: central sulcus; IFG: inferior frontal gyrus; IFS: inferior frontal sulcus; IOS: inferior occipital sulcus; IPS: intraparietal sulcus; ISJ: intermediary sulcus of Jensen; ITG: inferior temporal gyrus; ITS: inferior temporal sulcus; MFG: middle frontal gyrus; MFS: middle frontal sulcus; MOG: middle occipital gyrus; MTG: middle temporal gyrus; OP: opercular part of inferior frontal gyrus; Orb: orbital part of inferior frontal gyrus; PostCG: postcentral gyrus; PostCS: postcentral sulcus; PreCG: precentral gyrus; PreCS: precentral sulcus; PSCR: posterior subcentral ramus of Sylvian fissure; SFG: superior frontal gyrus; SFS: superior frontal sulcus; SMG: supramarginal gyrus; SOG: superior occipital gyrus; SOS: superior occipital sulcus; SPaLob: superior parietal lobule; STG: superior temporal gyrus; STS: superior temporal sulcus; SyF: lateral or Sylvian fissure; Tr: triangular part of inferior frontal gyrus. (Adapted from Ribas (2010).)
Figure 2.14 (A) The hand motor activation site corresponds to a knob-like cortical area of the contralateral precentral gyrus, which in MRI axial planes usually resembles an inverted omega with the posterior end of the superior frontal sulcus pointing towards it. Functional MRI of the right (B) and left hand motor (C) activity of a patient that harbors a cavernoma within the most posterior aspect of the right middle frontal gyrus, disclosing cortical activities of the omega region of each contralateral precentral gyrus and supplementary motor area.
Omega: within the red circle; PreCG: precentral gyrus; PreCS: precentral sulcus; SFS: superior frontal sulcus (non-continuous, interrupted) CS: central sulcus; PostCS: postcentral sulcus; PreCS: precentral sulcus, SFS: superior frontal sulcus; SMA: supplementary motor area. (Courtesy of E. Amaro, Department of Radiology, University of São. Paulo Medical School.)
The superolateral gyri extend along its inferolateral border constituting the cerebral inferior surface with its orbital part (orbital gyri and basal aspect of rectus gyri) and its tentorial part (basal aspects of the inferior temporal, inferior occipital and lingual gyri, and fusiform gyrus). The gyri of the superolateral and inferior surfaces extend along their medial margins constituting the cerebral medial surface. This is characterized by a very well-defined C-shaped inner ring, primarily composed of two continuous gyri (cingulate and parahippocampal gyri), which is surrounded by a much less well-defined outer ring of gyri (medial aspects of rectus and superior frontal gyri, paracentral lobule, precuneus, cuneus, and medial aspect of lingual gyrus). Unlike the other gyri (neocortical), each parahippocampal gyrus (paleocortical) is harbored inside the more ancient hippocampus (archicortical) along the inferior horn of the lateral ventricle, with the amygdala anteriorly. Due to their disposition around each thalamus and hypothalamus, the structures of this inner ring are referred to as limbic structures (Figure 2.12.B).
Among all these features, the evident lateral (Sylvian) fissure and the uniquely oblique pre- and postcentral gyri with their related sulci predominantly show up as distinctive structures on the superolateral aspect of the brain. The macroscopic study of the sulci and gyri of each cerebral hemisphere should therefore begin with the identification of the lateral (Sylvian) fissure, which clearly separates the superolateral surfaces of the frontal and parietal lobes from the temporal lobe. This should be followed by identification of the precentral and postcentral gyri, which divide the portion of this surface that is superior and posterior to the Sylvian fissure into an anterior and a posterior half. On the cerebral medial surface, one should initially identify the cingulated and the parahippocampal gyri that constitute the very well-defined C-shaped inner ring, and then identify their surrounding gyri.
For practical purposes, Ecker (1869) identified the gyri through numbers. The superior, middle, and inferior frontal gyri were referred to as F1, F2, and F3; the superior, middle, and inferior temporal gyri as T1, T2, and T3, with T4 and T5 corresponding, respectively, to the fusiform (currently divided into T4 and O4 (Duvernoy, 1991)) and to the lingual gyri (currently O5 (Duvernoy, 1991)); the superior parietal lobule and the precuneus to P1, the supramarginal gyrus to P2, and the angular gyrus to P’2 (currently frequently referred to as P3); and the occipital superior, middle, and inferior gyri, respectively, to O1, O2, and O3.
The divisions of the cerebral hemispheres into lobes are described in detail in their specific sections.
2.2.3 The Cerebral Lobes and Related Regions
The arbitrary division of the cerebral hemispheres into lobes has been done through progressive editions of the Nomina Anatomica, which recently changed its denomination to Terminologia Anatomica – International Anatomical Terminology (Federative Committee on Anatomical Terminology, 1998), based on neural, morphological, and functional aspects and having as its main objective the establishment of a categorization that could help the medical practice in the fields of neurology, neurosurgery, and neuroradialogy.
The first version, known as the Basle Nomina Anatomica (BNA) was published in 1895 (His, 1895), dividing each cerebral hemisphere into frontal, parietal, occipital, and temporal lobes as already proposed by Gratiolet (1854 apud Pearce, 2006), and considering the insula as a separate addendum but not a lobe.
The following version did not change this division until the publication of the fourth edition of the Paris Nomina Anatomica (PNA) in 1975 (Excerpta Medica Foundation, 1975), which then considered the insula as another brain lobe, a notion that was also kept in the fifth edition published in 1980 (Excerpta Medica Foundation, 1980).
Finally, the Terminologia Anatomica – International Anatomical Terminology published in 1998 (Federative Committee on Anatomical Terminology, 1998) substituted the previous Nomina Anatomica adding a list of English terms in common usage to the revised Latin terminology, and included the limbic lobe as another subdivision of each cerebral hemisphere.
According to the official anatomical nomenclature, each cerebral hemisphere is then currently divided into six lobes: frontal, parietal, occipital, temporal, insular, and limbic lobes, which are described in the following sections.
In relation particularly to the pre- and postcentral gyri, some of the anatomists of the nineteenth century, such as Bischoff (Broca, 1876a apud Stone, 1991) and Taylor (Taylor and Haughton, 1900 apud Uematsu et al., 1992), had already started proposing to group these two together given their morphologically unique character and juxtaposition. Although, respectively more related to the anterior frontal and posterior parietal cortical areas, the pre- and postcentral gyri do constitute a morphological and functional unit given their anatomical continuity, their own reciprocal connection, and the observation that both generate a rather similar amount of corticospinal fibers to constitute the pyramidal tract (Brodal, 1981; Jane et al., 1967; Liu and Chambers, 1964 apud Brodal, 1981; Nyberg-Hansen and Brodal, 1963 apud Brodal, 1981; Standring, 2008). On clinical grounds, the vast dimensions and heterogeneity of the conventionally named frontal lobe justify its subdivision, and the pre- and postcentral gyri grouping was made more recently initially by Penfield (Penfield and Baldwin, 1952 apud Hansebout, 1977) who named these two gyri the Rolandic or sensorimotor cortex. Later, Rasmussen (1979, 1991a, 1991b) referred to them as the central region, and more recently, Yasargil (1994) designated these two gyri and their related sulci as the central lobe. Considering the criteria adopted by the previous Nomina Anatomica editions that progressively incorporated further divisions of the brain hemispheres into cerebral lobes, the pre- and postcentral gyri, and their related sulci could very well be grouped together as another cerebral lobe.
Parallel to the concept of cerebral lobes and to the knowledge of their underlying white matter fibers, the medical practice requires an understanding of the anatomy of the intracranial neural structures mainly regarding the three-dimensionality of these structures, the topographical relationships among them, their vascularization, and their relationships with the natural spaces containing the cerebrospinal fluid (CSF) and with the skull vault and base.
The identification of the encephalic structures, and of their occasional lesions, in all neuroimaging studies, always requires an initial recognition of the natural CSF spaces (subarachnoid space with sulci and fissures that constitute its outer limits, and inner ventricular cavities that delimit its deeper contours), and of the shape of its main and well-defined structures. Therefore, the anatomical characterization of well-defined regions is useful because it leads to a more specific understanding of their structures with their respective continuity, vascularization, and functions, and of their occasional harboring lesions.
For this reason, the main, well-defined cerebral topographic regions are described here together with their respective related cerebral lobes.
2.2.3.1 The Frontal Lobe
The frontal lobe corresponds to the rostral region of each cerebral hemisphere, and is the largest part of each hemisphere. It is delimited posteriorly by the central sulcus, anteriorly by the cerebral supracilliary margin, medially by the interhemispheric fissure, and laterally and inferiorly by the lateral (Sylvian) fissure (Figures 2.13 and 2.15).
Figure 2.15 The main sulci (A) and gyri (B) of the medial and basal temporo-occipital surfaces.
AntComm: anterior commissure; Ant and PostOlfS: anterior and posterior paraolfactory sulcus; CaF: calcarine fissure; CaN: caudate nucleus; CaS: callosal sulcus; CC: corpus callosum; CiG: cingulate gyrus; CiPo: cingulate pole; CiS: cingulate sulcus; ColS: collateral sulcus; CS: central sulcus; Cu: cuneus; Fo: fornix; FuG: fusiform gyrus; GRe: gyrus rectus; IIIv: third ventricle; InfRosS: inferior rostral sulcus; Ist: isthmus of cingulate gyrus; ITG: inferior temporal gyrus; IVeFo: interventricular foramen of Monro; LatV: lateral ventricle; LiG: lingual gyrus; MaCiS: marginal ramus of the cingulate sulcus; MedFG: medial frontal gyrus; OTS: occipitotemporal sulcus; PaCLob: paracentral lobule; PaCS: paracentral sulcus; PaOlfG: paraolfactory gyri; PaTeG: paraterminal gyrus; PHG: parahippocampal gyrus; POS: parieto-occipital sulcus; PreCS: precentral sulcus; PreCu: precuneus; RhiS: rhinal sulcus; RoCC: rostrum of the corpus callosum; SFG: superior frontal gyrus; Spl: splenium of corpus callosum; SubPaS: subparietal sulcus; SupRosS: superior rostral sulcus; TePo: temporal pole; Th: thalamus; Un: uncus. (Adapted from Ribas (2010).)
Its superolateral (dorsal) surface underlies the frontal bone and is constituted posteriorly by the slightly oblique precentral gyrus that extends to the medial surface, and the area of the frontal lobe lying anteriorly to it is divided into the longitudinal superior, middle, and inferior frontal gyri, with the frontal pole lying in front of these gyri.
The frontal basal (ventral) surface lies over the orbital part of the frontal bone and over the cribriform plate of the ethmoid bone, and is composed of the orbital and rectus gyri.
The medial frontal surface faces the falx cerebri, extends from the frontal to the central sulcus within the paracentral lobule, and consists of the medial aspect of the superior frontal gyrus.
The frontal lobe harbors the primary motor area (MI) within the precentral gyrus, the supplementary motor area (SMA) anteriorly and medially, and the ventral and dorsal premotor areas anteriorly and laterally with the frontal eye field cortical area in between, and hence is functionally predominantly related to motor functions. While the movement itself is generated within MI, the supplementary motor and the lateral premotor areas are believed to instruct the MI area (Brodal, 2010).
The most anterior and basal aspects of the frontal lobes are bilaterally related to judgment and complex volitional aspects of behavior.
2.2.3.1.1 Frontal Lobe Sulci and Gyri
The precentral gyrus is disposed obliquely along the superolateral surface of the cerebral hemisphere, with its upper aspect extending along its medial surface, and is delineated posteriorly by the central sulcus.
The central sulcus separates the frontal and parietal lobes and demarcates the primary motor and somatosensory areas of the cortex, located in the precentral and postcentral gyri, respectively. It starts in or near the superomedial border of the hemisphere, a little behind the midpoint between the frontal and occipital poles (Williams and Warwick, 1980), and runs, resembling a lengthened italic S (Talairach and Tornoux, 1993 apud Yousry et al., 1997), downward and forwards, to end usually a little above the posterior ramus of the lateral sulcus. The central sulcus is usually a continuous sulcus (92 percent in both hemispheres (Ono et al., 1990)).
Broca (Broca, 1888 apud Boling and Oliver, 2004; Testut and Jacob, 1932a) classically described the central sulcus as having two (superior and inferior) anteriorly convex genua, and one posteriorly convex middle genu. Cunningham (Cunningham, 1892 apud Yousry et al., 1997), and more recently Ono (1990), considered the central sulcus as having only two genua, but described the superior one as being posteriorly convex probably then consisting of both the superior and middle genua classically previously described (Broca, 1888 apud Boling and Oliver, 2004; Déjérine, 1895; Testut and Jacob, 1932a), and disregarded the most superior one because of its small size (Yousry et al., 1997).
Posteriorly, the precentral gyrus is superiorly and inferiorly connected and continuous with the postcentral gyrus along connections that encircle both extremities of the central sulcus, comprising altogether a lengthened and oblique ellipse excavated by the central sulcus.
The inferior connection corresponds to the subcentral gyrus which is delineated anteriorly and posteriorly by the anterior and posterior subcentral rami of the Sylvian fissure, respectively. It can be situated either completely over the Sylvian fissure or can be in part internal to the fissure, then giving the false impression that the central sulcus is a branch of the Sylvian fissure (Ribas et al., 2005a).
The superior connection corresponds to the paracentral lobule of Ecker (Déjérine, 1895) already disposed along the medial surface of the cerebral hemisphere inside the interhemispheric fissure, delineated anteriorly by the paracentral sulcus and posteriorly by the ascending and distal part of the cingulated sulcus, that is, the marginal ramus of the cingulated sulcus.
Broca also described a middle connection between these two gyri (“plis de passage” moyen of Broca) characterized by a gyral bridge usually hidden within the central sulcus, and which in the cortical surface corresponds to the classic middle genu of the central sulcus that is posteriorly convex (Broca, 1888 apud Boling and Oliver, 2004). For Régis, this middle connection corresponds to a sulcal root, and when it is developed enough to reach the brain surface, it interrupts the central sulcus (Régis et al., 2005).
Using functional resonance imaging, Yousry et al. (1997) described that the anatomical cortical location of the motor hand area is anatomically related to a knob-like structure of the precentral gyrus that, on the cortical surface, corresponds precisely to the classic middle knee of the central sulcus, which is topographically located at the level of the distal end of the superior frontal sulcus (Yousry et al., 1997; Ribas et al., 2006; Ribas, 2010). Having also studied cadaveric specimens, Yousry et al. observed that this posteriorly oriented protrusion of the precentral gyrus is delimited by two anteriorly directed fissures that deepen toward the base of the knob resulting in a characteristic inverted omega shape in the axial planes of the MRIs in 90 percent of the hemispheres. The occasional occurrence of a third fissure with a horizontal course between the two fissures is responsible for a horizontal epsilon shape in the other 10 percent of brain hemispheres (Yousry et al., 1997) (Figure 2.14). In the sagittal plane, the appearance of the knob assumes the shape of a posteriorly directed hook (Yousry et al., 1997).
Boling and Olivier (2004) studied the middle connection of the pre- and postcentral gyri (the “plis de passage” moyen of Broca), with positron emission tomography and MRI. Through fixed cadaveric brain dissections removing the precentral component of the “plis de passage” moyen (that corresponds to the cortical substratum of hand motor function), they demonstrated that hand sensory function is highly correlated with the postcentral component of this cortical fold. Utilizing cortical stimulation in a posterior study, Boling et al. (2008) identified a strong relationship among the “plis de passage” moyen, whole-hand sensory and motor stimulation responses, and functional magnetic resonance imaging (fMRI) hand activation.
The precentral gyrus is anteriorly delimited by the precentral sulcus which is systematically divided into a superior and an inferior precentral sulcus by an evident connection of the middle frontal gyrus with the precentral gyrus (Duvernoy, 1991; Ono et al., 1990; Petrides, 2012). Further connections of the superior, middle, and inferior frontal gyri can divide the superior and inferior precentral sulcus into additional segments (Ono et al., 1990).
The superior part of the precentral sulcus is very often interrupted superiorly due to a connection between the superior frontal and the precentral gyri. When interrupted, this gives rise to a more medial segment called the medial precentral sulcus, and this corresponds to the sulcus precentralis medialis of Eberstaller (Petrides, 2012). More dorsally and within the precentral region, there is frequently another short gyrus called the marginal precentral sulcus, which corresponds to the sulcus precentralis marginalis of Cunningham, and which may merge with the superior precentral sulcus or with the central sulcus (Petrides, 2012).
Inferiorly, the inferior segment of the precentral sulcus always ends inside the opercular part of the inferior frontal gyrus, giving rise to its U-shape (Ribas et al., 2005a; Ribas, 2010; Ribas et al., 2006).
In relation to the skull surface, the pre- and postcentral gyri are roughly parallel to the coronal suture, with the precentral sulcus placed slightly posterior to this suture (Ebeling et al., 1987; Ebeling et al., 1989; Ebeling and Steinmetz, 1995b; Gusmão et al., 2001; Pernkoff, 1980; Ribas et al., 2006).
The superior, middle, and inferior frontal gyri are then disposed longitudinally anteriorly to the precentral gyrus, and are, respectively, separated by the superior and inferior frontal sulci. These frontal gyri are frequently referred to as F1, F2, and F3 (Ecker, 1869; Duvernoy, 1991) (Figure 2.17).
Figure 2.16 Anterior view of the cerebral hemispheres (A) and a view of the basal frontotemporal surface (B).
AntOrbG: anterior orbital gyrus; AntPerfSubst: anterior perforated substance; ARSyF: anterior ramus or stem of lateral or Sylvian fissure; BrSt: brainstem (pons); ColS: collateral sulcus; FMaS: frontomarginal sulcus; FuG: fusiform gyrus; GRe: gyrus rectus; HySta: hypophyseal stalk; IFG: inferior frontal gyrus; IFS: inferior frontal sulcus; IHF: interhemispheric fissure; Ist: isthmus of cingulate gyrus; ITG: inferior temporal gyrus; ITS: inferior temporal sulcus; LatOlfStr: lateral olfactory striae; LatOrbG: lateral orbital gyrus; MaBo: mammillary body; MedOlfStr: medial olfactory striae; MedOrbG: medial orbital gyrus; MeFS: medial frontal sulcus; MFG: middle frontal gyrus; MFS: middle frontal sulcus; MTG: middle temporal gyrus; OlfBu: olfactory bulb; OlfS: olfactory sulcus; OlfTr: olfactory tract; OptTr: optic tract; Orb: orbital part of inferior frontal gyrus; OrbGi: orbital gyri; OrbS: orbital sulcus; OTS: occipitotemporal sulcus; PHG: parahippocampal gyrus; PostMedOrbLob: posteromedial orbital lobule; PostOrbG: posterior orbital gyrus; PostPerfSubst: posterior perforated substance; RhiS: rhinal sulcus; SFG: superior frontal gyrus; SFS: superior frontal sulcus; Spl: splenium of corpus callosum; STG: superior temporal gyrus; STS: superior temporal sulcus; TePo: temporal pole; Un: uncus; IIn: optical nerve; IIIn: oculomotor nerve.
Figure 2.17 Superior view of the cerebral hemispheres.
AG: angular gyrus; CaF: calcarine fissure; CS: central sulcus; IOG: inferior occipital gyrus; IPaLob: inferior parietal lobule; IPS: intraparietal sulcus; MOG: middle occipital gyrus; PaCLob: paracentral lobule; POArc: parieto-occipital arch; POS: parieto-occipital sulcus seen on the superolateral surface, which corresponds to the parieto-occipital incisure within the parieto-occipital arch; PosCS: postcentral sulcus; PostCG: postcentral gyrus; PreCG: precentral gyrus; PreCu: precuneus; PreOccNo: pre-occipital notch; SFG: superior frontal gyrus; SFS: superior frontal sulcus; SMG: supramarginal gyrus; SOG: superior occipital gyrus; SPaLob: superior parietal lobule. (Adapted from Ribas (2010).)
The superior frontal gyrus is continuous with the rectus gyrus anteriorly and inferiorly, and can also be connected to the orbital gyri and to the middle frontal gyrus. Posteriorly, the superior frontal gyrus is connected to the precentral gyrus by at least one fold, which more commonly lie medially along the interhemispheric fissure. Usually, the superior longitudinal gyrus is subdivided into two longitudinal portions by the so-called medial frontal sulcus, and its medial portion is also called the medial frontal gyrus by some authors (Ono et al., 1990).
Along the most medial portion of the superior frontal gyrus and immediately facing the precentral gyrus is the important region known as the supplementary motor area (SMA). This corresponds to the medial premotor cortex, related to performing learned sequences of movements and which has poorly defined borders (Brodal, 1981; Williams and Warwick, 1980; Standring, 2008). Anteriorly to the SMA there is the Pre-SMA area believed to be involved in learning sequential movements (Figure 2.14B).
The superior frontal sulcus that separates the superior and middle frontal gyri is a very deep and frequently continuous sulcus (40 percent in the right side, 32 percent in the left side (Ono et al., 1990)), and ends posteriorly encroaching the precentral gyrus at the level of its already mentioned omega region (Yousry et al., 1997). This corresponds to the portion of the gyrus that functionally harbors the motor representation of the contralateral hand. Therefore, the superior frontal sulcus tends to point toward the middle frontoparietal “plis de passage,” as well as to the middle knee of the precentral gyrus, with its respective motor representation of the hand (Boling et al., 1999).
The most caudal aspect of the superior frontal gyrus anterior to the precentral gyrus, corresponds to the dorsal premotor (dPM) cortical area which is involved with planning movements.
The middle frontal gyrus is typically the largest of the frontal gyri and harbors a complex of multiple shallow sulcal segments known altogether as the middle (Duvernoy, 1991; Petrides, 2012) or intermediate frontal sulcus (Ono et al., 1990). Usually the middle frontal gyrus is superficially connected to the precentral gyrus by a prominent root that lies between the extremities of a marked interruption in the precentral sulcus. Within its caudal portion are the frontal eye fields (FEF), the cortical area responsible for saccadic eye and voluntary gaze movements.
The inferior frontal sulcus is always interrupted, due to the multiple connections between the middle frontal gyrus and the inferior frontal gyrus.
The constant emergence of the horizontal and anterior ascending rami of the lateral (Sylvian) fissure from the anterior Sylvian point (Ribas et al., 2005a), which divides this fissure into anterior and posterior branches (Yasargil et al., 2002a; Yasargil, 1984a; Yasargil et al., 1976; Yasargil and Abdulrauf, 2003; Ono et al., 1990), characterizes the triangular part of the inferior frontal gyrus in between its orbital and opercular parts (Figure 2.13).
The orbital part is the most prominent of the three parts that constitute the inferior frontal gyrus. The triangular part is usually more retracted causing the small widening of the lateral (Sylvian) fissure that corresponds to the anterior Sylvian point at its base. The opercular part is always U-shaped harboring the inferior aspect of the precentral sulcus and is posteriorly continuous with the basal aspect of the precentral gyrus over the anterior subcentral ramus of the lateral (Sylvian) fissure. In some cases, the anterior basal portion of the opercular part is more developed and is divided by another branch of the lateral fissure that runs from front to back and is called the diagonal sulcus of Eberstaller. When the diagonal sulcus of Eberstaller is present, it divides the anterior portion of the opercular part into two triangular portions that are positioned inversely to each other.
In the dominant hemisphere, the opercular and triangular parts of the inferior gyrus correspond to the Broca area, which is responsible for the production of spoken language (Broca, 1861 apud Finger, 1994; Brodal, 1981; Heimer, 1995; Quiñones-Hinojosa et al., 2003; Williams and Warwick, 1980).
The most posterior aspect of the inferior frontal gyrus, given by the connection of its opercular part with the precentral gyrus, corresponds to the ventral premotor cortical area (vPM), and its stimulation causes speech arrest bilaterally (Duffau, 2011b).
Superiorly, the inferior frontal gyrus is crisscrossed by various small branches of the interrupted inferior frontal sulcus, with one of them typically piercing the superior aspect of the triangular part, usually as a descending branch from anterior to posterior (Ribas et al., 2005a) and called the triangular sulcus (Duvernoy, 1991; Petrides, 2012).
Inferiorly, the orbital part continues with the lateral orbital gyrus, at times passing under a shallow sulcus known as the fronto-orbital sulcus. The basal apex of the triangular part is always above the lateral (Sylvian) fissure, and the base of the opercular part can be located either superiorly or within the fissure (Ono et al., 1990; Ribas, 2005b).
Anteriorly, the inferior frontal gyrus terminates merging with the anterior portion of the middle frontal gyrus and all the frontal gyri together are anteriorly delineated by the appropriately named frontomarginal sulcus, which lies superior and parallel to the supraciliary margin, separating the superolateral and orbital frontal surfaces (Ono et al., 1990; Yasargil, 1994). Very frequently, this so-called frontomarginal sulcus of Wernicke (Duvernoy, 1991) is interrupted and composed of three segments (Petrides, 2012).
Posteriorly, the inferior frontal gyrus is connected to the precentral gyrus along the posterior aspect of its opercular part as already mentioned.
On the frontobasal or orbital surface of each frontal lobe, the deep olfactory sulcus lies longitudinally in a paramedian position harboring the olfactory tract and bulb. Posteriorly, the olfactory tract is divided into its medial and lateral striae, which delineate the anterior-most aspect of the anterior perforated substance (Figure 2.16).
Medial to the olfactory sulcus is the long and narrow gyrus rectus, considered the most anatomically constant of the cerebral gyri, which is continuous with the superior frontal gyrus.
Lateral to the olfactory sulcus are the orbital gyri, which account for the greatest proportion of the frontobasal surface. The anterior, posterior, medial, and lateral orbital gyri are delineated by the H-shaped orbital sulci, which are composed of the lateral and medial orbital sulci united by the transverse orbital sulcus, with all the segments corresponding together to the cruciform sulcus of Rolando. The posterior orbital gyrus is situated anterior to the anterior perforated substance and typically presents a configuration similar to a tricorner or “Napoleon” hat. This can facilitate its identification in anatomical specimens in which the H-shaped orbital sulcus presents variations.
While the anterior orbital gyrus is traversed by small intermediate orbital sulci, the posterior orbital gyrus is traversed by small posterior orbital sulci.
The posterior orbital gyrus is connected medially to the medial orbital gyrus, characterizing the posteromedial orbital lobule (Yasargil, 1994) situated posterior and along the olfactory tract and the lateral olfactory striae, which is in turn connected to the anterior portion of the insula via the transverse insular gyrus. The remaining orbital gyri are connected to the superior, middle, and inferior frontal gyri, along the frontal pole.
On its medial surface, the frontal lobe is inferiorly delimited by the cingulated sulcus, which starts within the subcallosal region and extends over the cingulated gyrus ending along an upward and curved segment known as the marginal ramus of the cingulated sulcus. Frequently, the cingulated sulcus ends anteriorly between the anterior aspect of the cingulate gyrus and a connection of this gyrus with the medial and anterior aspect of the superior frontal gyrus, and frequently shows inferiorly directed side branches (Ono et al., 1990) (Figure 2.15).
The paracentral lobule is bounded posteriorly by the marginal ramus, and anteriorly by the paracentral sulcus, a branch of the cingulate sulcus. The paracentral lobule harbors the distal part of the central sulcus, and inferiorly to it, the so-called paracentral fossa (Petrides, 2012).
Anteriorly to the paracentral lobule, the medial aspect of the superior frontal gyrus lies over the cingulated sulcus and the cingulated gyrus, merging inferiorly with the rectus gyrus (Duvernoy, 1991).
The rectus gyrus is bounded superiorly by the superior rostral sulcus, and harbors along its surface the shallower inferior rostral sulcus.
Around the posterior end of the superior rostral sulcus the cingulated gyrus systematically connects with the rectus gyrus through a very evident U-shaped cortical fold known as the cingulate pole (Yasargil, 1994), located immediately anteriorly to the subcallosal gyri (paraolfactory gyri, paraterminal gyrus) (Figure 2.15B).
Superiorly to the superior rostral sulcus, small supra-orbital sulci (Petrides, 2012) can be observed within the medial surface of the frontal pole, at the level of the knee of the corpus callosum.
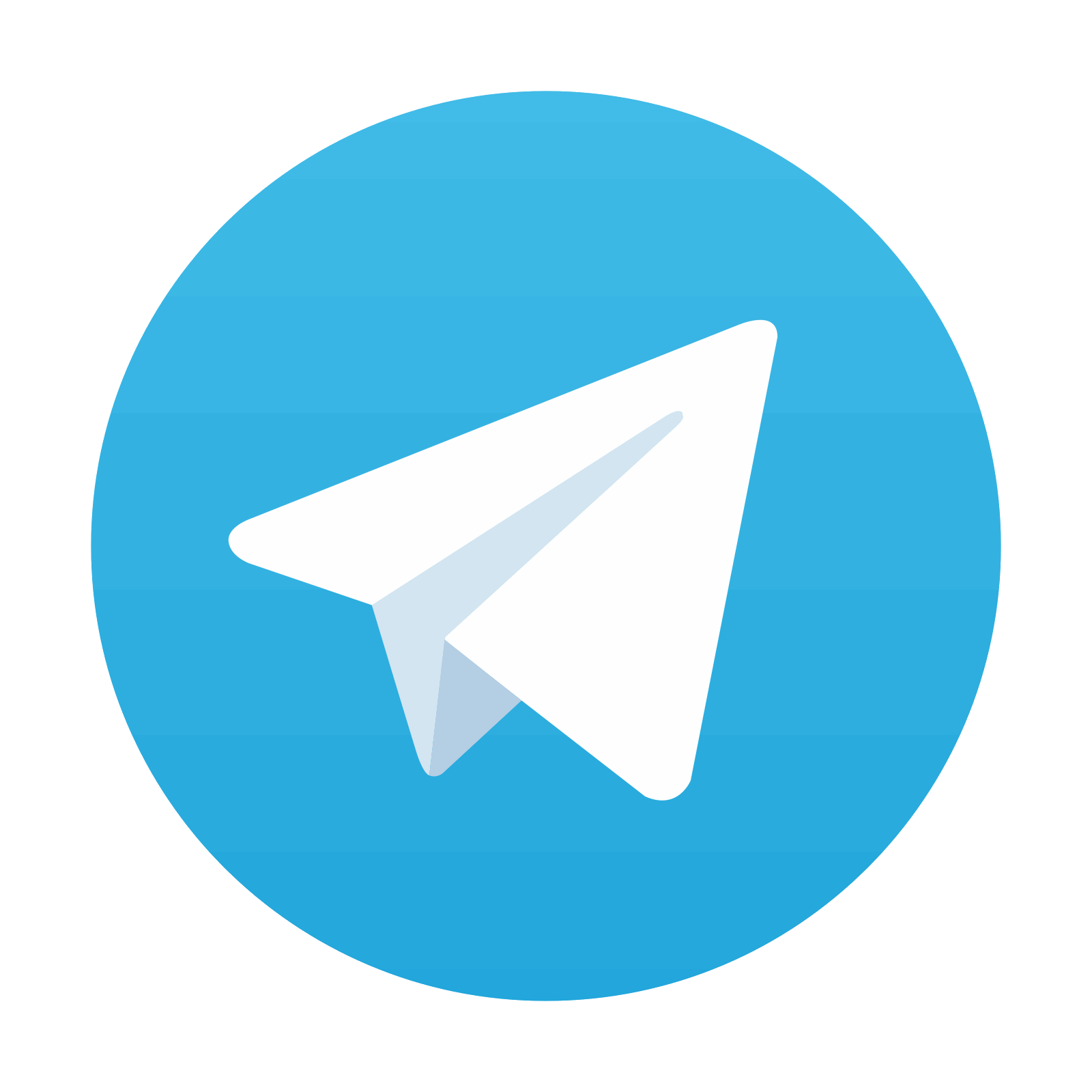
Stay updated, free articles. Join our Telegram channel

Full access? Get Clinical Tree
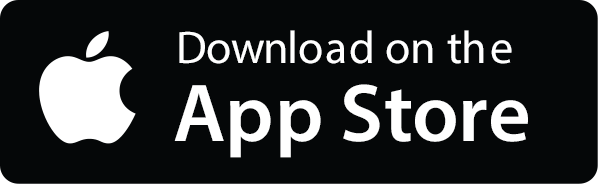
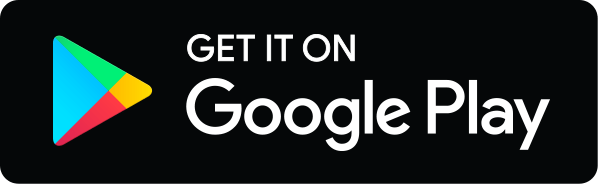
