Abstract
Autophagy is a term derived from the Ancient Greek word autóphagos, meaning “self-eating” or “self-devouring.” The term is used to describe a major cellular degradation process that ensures cellular quality control. By targeting dysfunctional cellular components and delivering them to the endosome–lysosome system for degradation, autophagy clears cells of cellular debris and recycles basic building blocks for de novo synthesis of cellular components.
Introduction
Autophagy is a term derived from the Ancient Greek word autóphagos, meaning “self-eating” or “self-devouring.” The term is used to describe a major cellular degradation process that ensures cellular quality control. By targeting dysfunctional cellular components and delivering them to the endosome–lysosome system for degradation, autophagy clears cells of cellular debris and recycles basic building blocks for de novo synthesis of cellular components. The term autophagy was coined in the 1960s by the Belgian biochemist Christian de Duve who observed that certain intracellular vesicles contained the properties to encircle and digest cytoplasmic material. He named these vesicles “lysosomes” and the process of cellular self-devouring “autophagy.” In 1974 he was awarded the Nobel Prize in Physiology or Medicine for his “discoveries concerning the structural and functional organization of the cell.” Around 20 years later, the Japanese cell biologist Yoshinori Ohsumi identified and isolated 15 autophagy genes in yeast, which he named Atg1-15. For his “discoveries of mechanisms for autophagy” he was awarded the Nobel Prize in Physiology or Medicine in 2016. In recent years, the broad availability of next-generation sequencing technologies allowed the identification of a number of pathogenic variants in core autophagy genes that give rise to a novel class of inborn disorders of neurometabolism [1–3]. These “congenital disorders of autophagy” share striking phenotypic similarities and often affect the cerebellum, the long white matter tracts, and the basal ganglia leading to complex movement disorders. Here, we provide an overview of the molecular mechanisms of autophagy and discuss congenital disorders of autophagy with prominent movement disorders by the examples of eight recently identified single gene disorders.
The Autophagy Pathway: Molecular Mechanisms
Autophagy has been classically divided into three subtypes: microautophagy, chaperone-mediated autophagy, and macroautophagy. Macroautophagy, hereafter referred to as “autophagy,” is the most important and extensively studied subtype occurring in all eukaryotic cells (Figure 20.1). Autophagy is an evolutionary highly conserved degradation pathway characterized by de novo synthesis of double-membrane vesicles, termed autophagosomes, that selectively target cellular molecules and organelles and deliver them to the lysosomal degradation machinery [4, 5]. This precise stepwise degradation process of cellular components is tightly controlled by a core set of autophagy-related genes. To ensure cellular homeostasis, autophagy dynamically integrates external and internal environmental stimuli to meet the metabolic needs of the cell. Whenever the cell’s energy stores are filled, anabolic pathways block autophagic degradation of cellular components and fuel protein and organelle biosynthesis. Conversely, in situations of cellular energy depletion, catabolic pathways activate autophagy to ensure the breakdown of macromolecules to maintain cellular metabolism. To efficiently regulate these complex cellular processes, key regulatory pathways of cellular metabolism, namely the mTORC1 and AMPK pathways, converge on autophagy initiation and tightly regulate this early step (Figure 20.1).
Figure 20.1 Overview of macroautophagy. Macroautophagy is a stepwise process resulting in the formation of double-membrane-bound autophagic vesicles that engulf their cargo before fusing with lysosomes. The principle stages of macroautophagy include: Initiation with nucleation of an isolation membrane (also called phagophore); elongation of evolving autophagic vesicles; engulfment of cargo and closure of the autophagosomal membrane; autophagosome maturation; fusion with late endosomes or lysosomes; and finally degradation of cargo through lysosomal hydrolases. The last step yields basic metabolites that are then recycled.
Once autophagy is initiated, an isolation membrane (the phagophore) forms and subsequently undergoes elongation and maturation (Figure 20.1). This is regulated by Atg family members involving two ubiquitination-like conjugation systems. During the process of elongation and maturation, specific autophagy receptors and anchoring molecules such as SQSTM1/p62 recruit cargo labelled for autophagic degradation to the growing phagophore. Eventually, the phagophore closes and forms a mature autophagosome, a double-membrane vesicle coated with the surface protein LC3, a widely used laboratory marker to monitor autophagy [6]. In the next step, the mature autophagosome fuses with late endosomes or lysosomes, forming what is called an autolysosome (Figure 20.1). Here, hydrolytic lysosomal enzymes are introduced, enabling degradation of the sequestered cargo under an acidic pH. Finally, the basic molecular building blocks are recycled to the cytosol for de novo synthesis of cellular components. Similarly, the lysosomal membranes are retrieved and re-join the perinuclear lysosomal pool, readily available for a new cycle of autophagy (Figure 20.1).
The Role of Autophagy in Health and Disease
Autophagy acts as a survival mechanism under conditions of stress and low energy levels by selectively clearing cells of macromolecules and dysfunctional organelles and regenerating metabolic precursors. Thus, autophagy can be regarded as a protective mechanism preventing cell death. Autophagic pathways are involved in multiple cellular processes, and important roles in adaptive immune response, pulmonary disease, vascular disease, metabolic disease, cancer, and aging have been reported [5]. Efficient autophagic flux is particularly important for post-mitotic cells, such as neurons, that lack the ability to dispose of dysfunctional organelles through cell division. In neurodevelopment, autophagy is a critical pathway that holds a key role in axon outgrowth, synaptic function, and neurotransmission. Not surprisingly, primary disorders of autophagy present with profound neurological manifestations. Interestingly, the cerebellum, the long white matter tracts, and the basal ganglia seem to be particularly vulnerable to disruptions in autophagy and lysosomal degradation pathways, clinically resulting in complex movement disorders such as ataxia, spasticity, dystonia, and parkinsonism. To illustrate the impact of perturbed autophagy on neurometabolism and the development of movement disorders, we summarize eight congenital disorders of autophagy.
Congenital Disorders of Autophagy
In recent years, a number of distinct childhood-onset neurological diseases caused by mutations in core autophagy genes have been identified. This group is rapidly expanding. The so-far identified genetic variants are mostly inherited in an autosomal-recessive manner (except X-linked BPAN, see below). All known variants map to genes in core autophagy genes and are believed to be loss-of-function mutations, perturbing autophagic flux.
Unifying Features
Congenital disorders of autophagy are single-gene disorders of the autophagy pathway. All congenital disorders of autophagy have an onset in early childhood, often as early as the neonatal period. Since autophagy is a ubiquitously expressed pathway, perturbations in the pathway often lead to multisystem disease. Due to the dependence of post-mitotic cells on efficient autophagic flux, neurons are severely affected, leading to neuronal dysfunction and neurodegeneration. Common brain imaging findings include cerebellar hypoplasia or atrophy, thinning of the corpus callosum, and storage disease phenotypes in some. Most disorders show a neurodevelopmental and neurodegenerative phenotype with developmental delay, intellectual disability, seizures, and movement disorders such as ataxia, gait disorders, spasticity, dystonia, and parkinsonism.
WDR45-Associated BPAN
Beta-propeller protein-associated neurodegeneration (BPAN) is a rare subtype of neurodegeneration with brain iron accumulation (NBIA). The NBIA spectrum encompasses a heterogeneous group of diseases sharing the key unifying features of an abnormal iron deposition in the basal ganglia, mainly the globus pallidus and substantia nigra. However, the cortex and cerebellum can also be affected. The clinical picture of NBIA spectrum diseases can range from severe early-onset neurodegeneration with rapid neurodevelopmental regression to a mild parkinsonian syndrome with minimal cognitive impairment in adulthood [7]. BPAN is the only X-linked subtype of the NBIA spectrum and affects mostly females, although some male patients have been reported. The underlying genetic causes are variants in WDR45, a gene that plays an important role in autophagosome formation and elongation [8]. Most mutations are sporadic and no mutational hotspots or genotype–phenotype correlations are known. Clinically, BPAN is characterized by a remarkable biphasic disease course with global developmental delay in childhood, followed by gradual neurodegeneration in adulthood, presenting with progressive dystonia, parkinsonism, and dementia [9]. Due to its distinct biphasic disease course BPAN was previously described as “static encephalopathy of childhood with neurodegeneration in adulthood” (SENDA) [8]. Affected children present with developmental delay, intellectual disability, and motor symptoms, such as spasticity or a broad-based, ataxic gait. Seizures are common and reported seizure types include focal seizures with impaired awareness, absence seizures, atonic seizures with head nodding, epileptic spasms, generalized tonic–clonic seizures, and myoclonic seizures. Individuals may present with multiple seizure types that may be refractory to medical therapy. Some patients show sleep disorders, ocular defects, and Rett-like hand stereotypies.
After a static disease phase, the second phase begins in adolescence or early adulthood when patients develop parkinsonism, dystonia, and progressive cognitive deficits. The parkinsonian features include bradykinesia, rigidity, freezing, and postural instability. Tremor is less common.
Therapeutic management can be challenging. For parkinsonism and dystonia, some short-lived response to levodopa has been observed; however, nearly all patients experienced early motor fluctuations, rapidly progressing to disabling dyskinesia [9, 10], requiring discontinuation of treatment. Cognitive decline may be insidious at first with the gradual loss of expressive language; however, eventually all patients advance to profound dementia.
Neuroimaging can establish the diagnosis. On T2-weighted sequences, iron accumulation can be detected as hypointensities in the globus pallidus, substantia nigra, and cerebral peduncles. On T1 sequences, the pathogenic changes in the substantia nigra produce a pathognomonic hyperintense “halo” sign, extending to the cerebral peduncles. These imaging findings are thought to represent iron-neuromelanin complexes, which are formed following neuromelanin release from degenerating neurons of the substantia nigra pars compacta. Further, non-specific findings on MRI include hypomyelination, thinning of the corpus callosum, cerebellar atrophy, and, as the diseases progresses, global cerebral atrophy.
Using exome sequencing, mutations in the X-chromosomal gene WDR45, the human ortholog of yeast Atg18, have been discovered as the genetic cause of BPAN [8, 11]. WDR45 is a member of the WD40 repeat family of proteins. WD40 repeat proteins assume a highly symmetrical, seven-bladed, beta-propeller scaffold that serves as a platform for specific protein interactions. WDR45 enables interactions with phospholipids, and importantly acts as a platform for protein interaction of the known autophagy proteins Atg2 and Atg9, which are involved in autophagosome formation and elongation [12].
SNX14-Associated Autosomal-Recessive Spinocerebellar Ataxia 20
Autosomal-recessive spinocerebellar ataxia 20 (SCAR20) has been described as a distinct recognizable ataxia syndrome, characterized by early-onset cerebellar atrophy and a storage-phenotype due to mutations in the sorting nexin 14 (SNX14) gene. Most patients with SCAR20 come to clinical attention in the first year of life due to global developmental delay and hypotonia. On examination, most patients show ataxia, nystagmus, impaired ambulation, and reduced deep tendon reflexes. Progressive coarsening of facial features with a prominent forehead, epicanthal folds, upturned nares, long philtrum, full lips, hypertrichosis, kyphoscoliosis, and hepatosplenomegaly have been reported and confirm an overlap with lysosomal storage disorders. Additional features include sensorineural hearing loss, camptodactyly, relative macrocephaly, and seizures [13].
On neuroimaging, cerebellar atrophy involving both cerebellar hemispheres and the vermis is evident. Separation of the folia, indicating atrophy rather than hypoplasia, with relative sparing of the pons represents distinctive features from the group of pontocerebellar hypoplasias [14]. The underlying molecular genetic defects of SCAR20 were recently identified as truncating bi-allelic mutations in the SNX14 gene on chromosome 6q14.3, encoding a ubiquitously expressed modular PX-domain-containing sorting factor [13]. SNX14 is expressed in all brain regions with the highest expression levels in the cerebellum. SNX14 localizes to lysosomes and associates with phosphatidyl-inositol residues, a key component in late endosome/lysosome pathways. Induced-pluripotent-stem-cell-derived neuronal precursor cells from SNX14-patients show enlarged lysosomes and slower autophagy flux with the accumulation of autophagy markers such as LC3-II and p62, confirming disruption in the autophagy pathway [13, 14].
ATG5-Associated Autosomal-Recessive Ataxia Syndrome
In 2016, a homozygous missense variant in the ATG5 gene was identified in siblings with cerebellar hypoplasia and early-onset non-progressive ataxia [15]. ATG5 is one of the core autophagy proteins involved in the elongation of the phagophore. As part of the Atg5–Atg12–Atg16L1 conjugation system, ATG5 is involved in the lipidation of LC3-I to LC3-II, therefore supporting elongation and closure of the autophagosome. Interestingly, the ataxia in these siblings was non-progressive and no signs of cerebellar degeneration on neuroimaging could be detected on close follow-up over two decades. In yeast, the homologous Atg5 mutation led to a 30–50% reduction of induced autophagy and a Drosophila melanogaster model expressing pathogenic E122D human ATG5 confirmed an ataxic phenotype. These findings illustrate that even slight perturbations in the autophagy pathway, although not leading to prominent cerebellar neurodegeneration, may still cause clinically significant disturbances in cerebellar pathways.
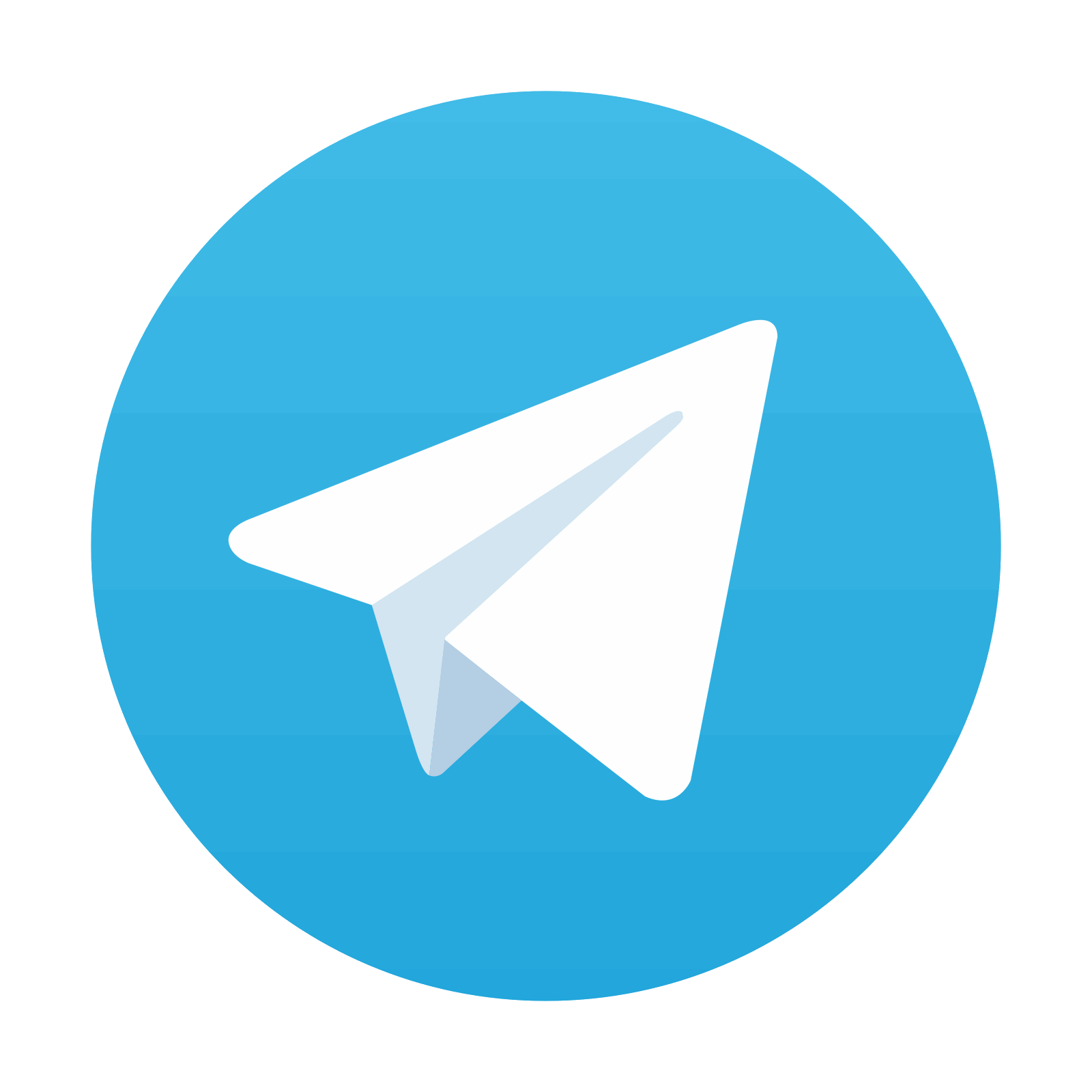
Stay updated, free articles. Join our Telegram channel

Full access? Get Clinical Tree
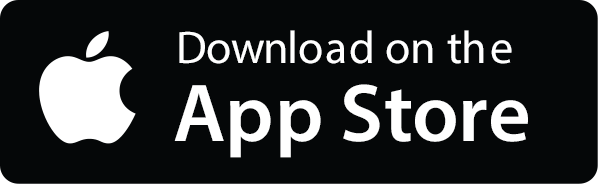
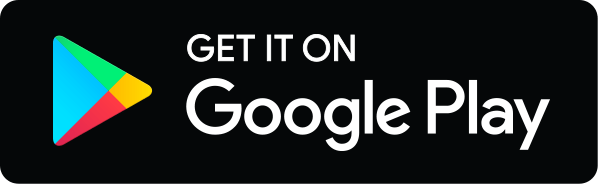
