Neurologic Disorders Caused by Lesions in Specific Parts of the Cerebrum: Introduction
The long-standing controversy about cerebral functions, whether they are diffusely represented in the cerebrum with all parts roughly equivalent, or localized to certain lobes or regions, has been resolved to the satisfaction of most neurologists. Clinicians have demonstrated beyond doubt that particular functions are assignable to certain cortical regions. For example, the pre- and postrolandic zones control motor and sensory activities, respectively, the striate occipital zones control visual perception, the superior temporal gyri are auditory, and so on. Beyond these broad correlations, however, there is a notable lack of precision in the cortical localization of most of the behavioral and mental operations described in Chaps. 20 and 21. In particular, of the higher order functions, such as attention, vigilance, apperception, and analytic and synthetic thinking, none has a precise and predictable anatomy; or, more accurately, the neural systems on which they depend are widely distributed among several regions.
One may inquire into what precisely is meant by cerebral localization. Does it refer to the physiologic function of a circumscribed group of neurons in the cerebral cortex, indicated clinically by a loss of that function when the neurons in question are destroyed? This is the way in which neurologists have assigned functions to particular areas of the cerebral cortex. However, from what we know of the rich connectivity of all parts of the specialized cortical centers, one must assume that this is only partly the case. Most who ponder this subject believe that the organization of cerebral function is based on discrete networks of closely interconnected afferent and efferent neurons in several regions of the brain. These ensembles must be linked by both regional and more widespread systems of fibers. This is especially apparent in the discussion of the anatomy of complex cognitive properties such as intelligence, as described in Chap. 21. Thus, many basic functions are anchored in one cortical region and a lesion there causes loss of a particular ability. But it is apparent from physiologic studies such as functional imaging and electromagnetic stimulation that widely distributed networks are engaged, which nonetheless encompasses the region that can be ablated and eliminate the function in question.
These aspects of cerebral localization—brought out so clearly in the writings of Wernicke, Déjerine, and Liepmann, have been elaborated by Luria (1966 and 1969) and the Russian school of physiologists and psychologists and extended by Geschwind (1965). In keeping with the model of interconnected networks, they viewed function not as the direct property of a particular, highly specialized region of the cerebrum but as the product of complex, diffusely distributed activity by which sensory stimuli are analyzed and integrated at various levels of the nervous system and then united, through a system of temporarily acquired connections, into a working mosaic adapted to accomplish a particular task. To some extent, this model has been corroborated by functional imaging studies, which show increased metabolic activity in several cortical regions during almost every form of human behavior, including willed motor acts, language tasks, and those coinciding with perceptive and apperceptive sensory experiences. Within such a functional system, the initial and final points (the task and the effect) remain unchanged, but the intermediate links (the means of performance of a given task) may be modified within wide limits and will never be exactly the same on two consecutive occasions. Thus, when a certain act is called for by a spoken command, the dominant temporal lobe must receive the message and transmit it to the premotor areas. Or it may be initiated by the intention of the individual, in which case the first measurable cerebral activity (a “readiness potential”) occurs anterior to the premotor cortex. The motor cortex is also always under the dynamic control of the proprioceptive, visual, and vestibular systems. Thus, a lesion that affects any one of several elements in the act may cause loss of a skilled ability, either the motor centers themselves or their connections with the other elements.
Another theoretical scheme of cerebral function identifies cortices of similar overall structure and divides the cerebral mantle into three longitudinally oriented zones, the triune brain articulated by Paul Maclean. A central vegetative neuronal system (allocortex and hypothalamus) provides the mechanisms for all internal functions, the milieu intérieur of Bernard and Cannon. An outer zone, comprising the sensorimotor and association cortices and their projections, provides the mechanisms for perceiving the external world and interacting with it, and a region between them (limbic–paralimbic cortices) that provides the bridges that permit the adaptation of the organism’s needs to the external environment. This ostensibly metaphoric concept of central nervous system function, first proposed by Broca, was elaborated by Yakovlev and has been adopted more recently by Benson and by Mesulam (1998). Such a model retains to a large degree the cytoarchitectural similarities among areas that serve similar functions (i.e., the scheme of Brodmann discussed further on) and also respects the sequence of brain maturation (myelination) of connecting pathways proposed by Flechsig (see Fig. 28-3). In this way, localization may be viewed as the product of genetic patterns of structure, which mature during development, and their synaptic formations, which permit the development of complex circuits during lifelong learning and experience.
It is worthwhile to point out that these broadened concepts of cerebral function, which apply to all mental activities, contradicts both the historical notion that there is a functional equivalence of all cerebral regions and also the more recently developed one that assumes strict localization of any given activity.
From these remarks, it follows that subdivision of the cerebrum into frontal, temporal, parietal, and occipital lobes is somewhat of an abstraction in terms of landmarks and cerebral function. Some of these delineations were made long before our first glimmer of knowledge about the function of the cerebrum. Even when neurohistologists began parceling the neocortex, they found that their areas did not fall neatly within zones bounded by sulci and fissures. Therefore, when the terms frontal, parietal, temporal, and occipital are used, it is largely to provide the clinician with familiar and manageable anatomic landmarks for localization (Fig. 22-1).
The current method of study of cortical activity is by functional imaging techniques (positron emission tomography [PET] and functional magnetic resonance imaging [fMRI]). Invariably, an ensemble of areas, a “network” of the variety described earlier, is activated to perform even seemingly simple tasks such as recalling a name, visualizing or identifying an object, or carrying out a commanded task. The fact that multiple areas of the cortex are entrained may seem at odds with the classic view of lesional neurology, but as already stated, the discrepancy is one of epistemology in that normal function does not equate with abnormal function as exposed by a focal lesion. A lesion in the cerebrum merely exposes the site at which damage results in the greatest loss of that particular function but does not reveal the much wider area that is essential for the full normal operation of that function. Imaging studies similarly demonstrate that certain regions of the cortex are necessary to fully conduct particular behaviors, but they are not sufficient for their enactment.
General Anatomic and Physiologic Considerations of Cortical Function
Pertinent to this subject are a number of morphologic and physiologic observations. Along strictly histologic lines, Brodmann distinguished 47 different areas of cerebral cortex (Fig. 22-2), and von Economo identified more than twice that number. Although this parceling was severely criticized by Bailey and von Bonin (and the data upon which Brodmann based his system were never published), it is still used by physiologists and clinicians, who find that the Brodmann areas do indeed approximate certain functional zones of the cerebral cortex (Fig. 22-3). Also, the cortex has been shown to differ in its various parts by virtue of connections with other areas of the cortex and with the thalamic nuclei and other lower centers. Hence, one must regard the cortex as a heterogeneous array of many anatomic systems, each with highly organized intercortical and diencephalic connections.
Figure 22-3.
A and B. Approximate distribution of functional zones on lateral (A) and medial (B) aspects of the cerebral cortex. Abbreviations: A1, primary auditory cortex; AA, auditory association cortex; AG, angular gyrus; CG, cingulate cortex; IPL, inferior parietal lobule; IT, inferior temporal gyrus; M1, primary motor area; MA, motor association cortex; MPO, medial parietooccipital area; MT, middle temporal gyrus; OF, orbitofrontal region; PC, prefrontal cortex; PH, parahippocampal region; PO, parolfactory area; PS, peristriate cortex; RS, retrosplenial area; S1, primary somatosensory area; SA, somatosensory association cortex; SG, supramarginal gyrus; SPL, superior parietal lobule; ST, superior temporal gyrus; TP, temporopolar cortex; V1, primary visual cortex; VA, visual association cortex. (Redrawn by permission from M-M Mesulam.)
The sheer size of the cortex is remarkable. Unfolded, it has a surface extent of about 4,000 cm2, about the size of a full sheet of newsprint (right and left pages). Contained in the cortex are many billions of neurons (estimated at 10 to 30 billion) and five times this number of supporting glial cells. The intercellular synaptic connections number in the trillions. Because nerve cells look alike and presumably function alike, the remarkable diversity in human intelligence, store of knowledge, and behavior must depend on the potential for almost infinite variations in neuronal interconnectivity.
Most of the human cerebral cortex is phylogenetically recent, hence the term neocortex. It was also referred to as isocortex by Vogt because of its uniform embryogenesis and morphology. These latter features distinguish the neocortex from the older and less uniform allocortex (“other cortex”), which comprises mainly the hippocampus and olfactory cortex. Concerning the detailed histology of the neocortex, six layers (laminae) can be distinguished—from the pial surface to the underlying white matter they are as follows: the molecular (or plexiform), external granular, external pyramidal, internal granular, ganglionic (or internal pyramidal), and multiform (or fusiform) layers (illustrated in Fig. 22-4). Two cell types—relatively large pyramidal cells and smaller, more numerous rounded (granular) cells—predominate in the neocortex, and variations in its lamination are largely determined by variations in the size and density of these neuronal types.
Figure 22-4.
The basic cytoarchitecture of the cerebral cortex, adapted from Brodmann. The six basic cell layers are indicated on the left, and the fiber layers on the right (see text).
Many variations in lamination have been described by cortical mapmakers, but two main types of neocortex are recognized: (1) the homotypical cortex, in which the six-layered arrangement is readily discerned, and (2) the heterotypical cortex, in which the layers are less distinct. The association cortex—the large areas (75 percent of the surface) that are not obviously committed to primary motor or sensory functions—is generally of this latter type. Homotypical areas are characterized by either granular or agranular nerve cells. The precentral cortex (Brodmann areas 4 and 6, mainly motor region) is dominated by pyramidal rather than granular cells, especially in layer V (hence the term agranular). Agranular cortex is distinguished by a high density of large pyramidal neurons. In contrast, the primary sensory cortices, postcentral gyrus (areas 3, 1, 2), banks of the calcarine sulcus (area 17), and the transverse gyri of Heschl (areas 41 and 42), where layers II and IV are strongly developed for the receipt of afferent impulses, has been termed granular cortex because of the marked predominance of granular cells a preponderance of which are small neurons (Fig. 22-5).
Figure 22-5.
Four fundamental types of cerebral cortex and their distribution in the cerebrum. The primary visual cortex has a preponderance of small neurons; hence, it was historically called “granular.” The primary motor cortex, by contrast, has relatively fewer small neurons and was described as “agranular.” (Reproduced with permission from Kandel ER, Schwartz JH, Jessel TM: Principles of Neural Science, 4th ed. New York, McGraw-Hill, 2000.)
Beyond these morphologic distinctions, the intrinsic organization of the neocortex follows a pattern elucidated by Lorente de Nó. He described vertical chains of neurons arranged in cylindrical modules or columns, each containing 100 to 300 neurons and heavily interconnected up and down between cortical layers and to a lesser extent, horizontally. Figures 22-4 and 22-5 illustrate the fundamental vertical (columnar) organization of these neuronal systems. Afferent fibers activated by various sensory stimuli terminate mainly in layers II and IV. Their impulses are then transmitted by internuncial neurons (interneurons) to adjacent superficial and deep layers and then to appropriate efferent neurons in layer V. Neurons of lamina III (association efferents) send axons to other parts of the association cortex in the same and opposite hemisphere. Neurons of layer V (projection efferents) send axons to subcortical structures and the spinal cord. Neurons of layer VI project mainly to the thalamus. In the macaque brain, each pyramidal neuron in layer V has about 60,000 synapses, and one afferent axon may synapse with dendrites of as many as 5,000 neurons; these figures convey some idea of the wealth and complexity of cortical connections. These columnar ensembles of neurons, on both the sensory and motor sides, function as the elementary working units of the cortex.
Whereas certain regions of the cerebrum are committed to special perceptual, motor, sensory, mnemonic, and linguistic activities, the underlying intricacy of the anatomy and psychophysical mechanisms in each region are just beginning to be envisioned. The lateral geniculate-occipital organization in relation to vision and recognition of form, stemming from the work of Hubel and Wiesel, may be taken as an example. In area 17, the polar region of the occipital lobe, there are discrete, highly specialized groups of neurons, each of which is activated in a small area of lamina 4 by spots of light or lines and transmitted via particular cells in the lateral geniculate bodies; other groups of adjacent cortical neurons are essential for the perception of color. Lying between the main unimodal receptive areas for vision, audition, and somesthetic perception are zones of integration called heteromodal cortices. Here neurons respond to more than one sensory modality or neurons responsive to one sense are interspersed with neurons responsive to another.
The integration of cortical with subcortical structures is reflected in volitional or commanded movements. A simple movement of the hand, for example, requires activation of the premotor cortex (also called accessory motor cortex), which projects to the striatum and cerebellum and back to the motor cortex via a complex thalamic circuitry before the direct and indirect corticospinal pathways can activate certain combinations of spinal motor neurons, as described in Chaps. 3 and 4.
Interregional connections of the cerebrum are required for all natural sensorimotor functions; moreover, as indicated above, their destruction disinhibits or “releases” other areas. Denny-Brown referred to the latter as cortical tropisms. Thus, destruction of the premotor areas, leaving the precentral and parietal lobes intact, results in release of sensorimotor automatisms such as groping, grasping, and sucking. Parietal lesions result in complex avoidance movements to contactual stimuli. Temporal lesions lead to a visually activated reaction to every observed object and its oral exploration, and limbic sexual mechanisms are rendered hyperactive.
Another group of disorders known as disconnection syndromes depend not merely on involvement of certain cortical regions but more specifically on the interruption of inter- and intrahemispheric fiber tracts. Extensive white matter lesions may virtually isolate certain cortical zones and result in a functional state that is the equivalent of destruction of the overlying cortical region. Some of these disconnections are indicated schematically in Fig. 22-6; the usually involved fiber systems include the corpus callosum, anterior commissure, uncinate temporofrontal fasciculus, occipito- and temporoparietal tracts. An example is the isolation of the perisylvian language areas from the rest of the cortex, as occurs with anoxic–ischemic infarction of border zones between major cerebral arteries (see “Disconnection Syndromes” further on).
Figure 22-6.
Connections involved in naming a seen object and in reading. The visual pattern is transferred from the visual cortex and association areas to the angular gyrus, which arouses the auditory pattern in the Wernicke area. The auditory pattern is transmitted to the Broca area through the arcuate fasciculus, where the articulatory form is aroused and transferred to the contiguous face area of the motor cortex. With destruction of the left visual cortex and splenium (or intervening white matter), the words perceived in the right visual cortex cannot cross over to the language areas and the patient cannot read.
Syndromes Caused by Lesions of the Frontal Lobes
The frontal lobes lie anterior to the central or rolandic sulcus and superior to the sylvian fissure (see Fig. 22-1). They are larger in humans (30 percent of the cerebrum) than in any other primate (9 percent in the macaque). Several systems of neurons are located here, and they subserve different functions. Brodmann areas 4, 6, 8, and 44 relate specifically to motor activities. The primary motor cortex, i.e., area 4, is directly connected with somatosensory neurons of the anterior part of the postcentral gyrus as well as with other parietal areas, thalamic and red nuclei, and the reticular formation of the brainstem. The supplementary motor cortex, a portion of area 6, shares most of these connections. As pointed out in earlier chapters, all motor activity requires sensory guidance, and this comes from the somesthetic, visual, and auditory cortices and from the cerebellum via the ventral tier of thalamic nuclei.
Area 8 is concerned with turning the eyes and head contralaterally. Area 44 of the dominant hemisphere (Broca area) and the contiguous part of area 4 are “centers” of motor speech and related functions of the lips, tongue, larynx, and pharynx. Left-sided lesions cause a distinctive articulatory and language syndrome, and bilateral lesions in these areas cause paralysis of articulation, phonation, and deglutition. The medial-orbital gyri and anterior parts of the cingulate and insular gyri, which are the frontal components of the limbic system, take part in the control of respiration, blood pressure, peristalsis, and other autonomic functions. The most anterior parts of the frontal lobes (areas 9 to 12 and 45 to 47), sometimes referred to as the prefrontal areas, are particularly well developed in human beings but have imprecisely determined functions. They are not, strictly speaking, parts of the motor cortex in the sense that electrical stimulation evokes no direct movement (the prefrontal cortex is said to be inexcitable). Yet these areas are involved in the initiation of planned action and executive control of all mental operations, including emotional expression.
The frontal agranular cortex (areas 4 and 6) and more specifically, pyramidal cells of layer V of the pre- and postcentral convolutions provide most of the cerebral efferent motor system that forms the pyramidal, or corticospinal, tract (see Figs. 3-2 and 3-3). Another massive projection from these regions is the frontopontocerebellar tract. In addition, there are several parallel fiber systems that pass from frontal cortex to the caudate and putamen, subthalamic and red nuclei, brainstem reticular formation, substantia nigra, and inferior olive, as well as to the ventrolateral, mediodorsal, and dorsolateral nuclei of the thalamus. Areas 8 and 6 are connected with the ocular and other brainstem motor nuclei and with identical areas of the other cerebral hemisphere through the corpus callosum. A tract, the fronto-occipital fasciculus, connects the frontal with the occipital lobe and the uncinate bundle connects the orbital part of the frontal lobe with the temporal lobe.
The granular frontal cortex has a rich system of connections both with lower levels of the brain (medial and ventral nuclei and pulvinar of the thalamus) and with virtually all other parts of the cerebral cortex, including its limbic and paralimbic parts. As to its limbic connections, the frontal lobe is unique among cerebrocortical areas in that electrical stimulation of the orbitofrontal cortex and cingulate gyrus has manifest effects on respiratory, circulatory, and other vegetative functions. These parts of the frontal cortex also receive major afferent projections from other parts of the limbic system, presumably to mediate the emotional responses to sensory experiences; they, in turn, project to other parts of the limbic and paralimbic cortices (hippocampus, parahippocampus, anterior pole of the temporal lobe), amygdala, and midbrain reticular formation. Chapter 25 describes these frontal–limbic connections in greater detail.
Most of the popular notions relating to the function of the frontal lobes are oversimplified. In the frontal lobe, are presumed to reside the mechanisms that govern personality, character, motivation, and our unique capacities for abstract thinking, introspection, and planning. These qualities and traits do not lend themselves to easy definition and study and certainly not to discrete localization. Most are too subtle to isolate or even to measure accurately. Except for the more posterior frontal mechanisms subserving motility, motor speech, and certain behaviors relating to impulse (conation), neurologists recognize that the other features of frontal lobe disease are more abstruse.
Blood is supplied to the medial parts of the frontal lobes by the anterior cerebral artery and to the convexity and deep regions, by the superior (rolandic) division of the middle cerebral artery. The underlying deep white matter is supplied by a series of small penetrating arteries, called lenticulostriate vessels that originate directly from the initial portion (stem) of the middle cerebral artery, as detailed in Chap. 34.
For descriptive purposes, the clinical effects of frontal lobe lesions can be grouped under the following categories: (1) motor abnormalities related to the prerolandic motor cortex; (2) speech and language disorders related to the dominant frontal lobe, which are described in the next chapter; (3) incontinence of bladder and bowel; (4) impairment of capacity for goal-directed sustained mental activity, and the ability to shift from one line of thought or action to another, i.e., aspects of attention manifest as impersistence and perseveration; (5) akinesia and lack of initiative and spontaneity (apathy and abulia); (6) changes in personality, particularly in mood and self-control (disinhibition of behavior); and (7) an abnormality of gait that has proved difficult to characterize (see also Chap. 7 on disorders of gait).
With regard to behavior and the frontal lobe, the anterior half of the brain is in a general sense committed to the planning, initiation, monitoring, and execution of all cerebral activity. This was aptly summarized by Luria (1966 and 1973) as “goal-directed behavior.” Of necessity in such a scheme, there must also be inhibitory mechanisms that control or modulate behavior. Thus, aside from the overt abnormalities of motor, speech, and voluntary movement, lesions of the frontal lobes give rise to a loss of drive, impairment of consecutive planning, an inability to maintain serial relationships of events, and to shift easily from one mental activity to another. These are combined with sucking, grasping, and groping reflexes and other obligate behaviors. In the emotional sphere, frontal lobe lesions may cause anhedonia (lack of pleasure), apathy, loss of self-control, disinhibited social behavior, and euphoria, as described further on.
Voluntary movement involves the motor cortex in its entirety or at least large parts of it, and of the various effects of frontal lobe lesions, most is known about the motor abnormalities. Electrical stimulation of the motor cortex elicits contraction of corresponding muscle groups on the opposite side of the body; focal seizure activity has a similar effect. Stimulation of Brodmann area 4 produces movement of discrete muscle groups or, if sufficiently refined, of individual muscles. Repertoires of larger coordinated movements are evoked by stimulation of area 6, the premotor and supplementary motor cortices.
Lesions in the posterior part of the frontal lobe cause spastic paralysis of the contralateral face, arm, and leg. Motor impulses from the frontal lobe are conducted by the direct corticospinal tract and by tracts that descend from the motor, premotor, supplementary motor, and anterior parietal cortex to the spinal cord, either directly or via the red and reticular nuclei in the brainstem. Lesions of the more anterior and medial parts of the motor cortex result in less paralysis and more spasticity, as well as a release of sucking, groping, and grasping reflexes, the actual mechanisms for which probably reside in the parietal lobe and which, as conceptualized by Denny-Brown, are tropisms or automatisms that are normally inhibited by the frontal cortex. When the lesions of the motor parts of the frontal lobe are bilateral, there is a tetraparesis in which the weakness is not only more severe but also more extensive than in unilateral lesions, affecting both spinal and cranial muscles (pseudobulbar palsy).
Ablation of the right or left supplementary motor areas (the parts of area 6 that lie on the medial surfaces of the cerebral hemispheres) was found by Laplane and colleagues (1977b) to cause mutism, contralateral motor neglect, and impairment of bibrachial coordination. On the basis of blood flow studies, Roland and colleagues and Fuster suggest that an important function of the supplementary motor area is the ordering of motor tasks or the recall of memorized motor sequences, further evidence of the executive functions of the frontal lobes. Some insight into organization in supplementary motor cortex is given by seizures originating there; they give rise to curious postures such as a fencing position or flailing of the opposite arm.
Temporary paralysis of contralateral eye turning and sometimes of head turning follows a destructive lesion in area 8, on the dorsolateral aspect (convexity) of the cerebral cortex, often referred to as the frontal eye field (see Fig. 22-1). Seizure activity in this area causes a tonic deviation of the head and eyes to the opposite side.
Destruction of the Broca convolution (areas 44 and 45) and the adjacent insular and motor cortex of the dominant hemisphere result in a reduction or loss of motor speech, and of agraphia, and apraxia of the face, lips, and tongue, as described in Chap. 23.
The gait condition described by Bruns that is caused by a frontal lobe lesion was designated by him as an ataxia of gait; he made no reference to an ataxia of limb movements. This disorder is often also referred to now as an apraxia of gait, inappropriately in our opinion, because the term apraxia is best used to describe an inability to carry out a commanded or learned motor task, not an ingrained one (see Chap. 3). What is meant by these terms in application to gait has never been clearly specified, but broadly speaking, they signify a loss of the ability to use the lower limbs in the act of walking that cannot be explained by weakness, loss of sensation, or ataxia. The patient may retain these fundamental motor and sensory functions when examined in bed and can even make motions that simulate walking while seated or reclining. As detailed in Chap. 7, the resultant pattern is a slowed, slightly imbalanced, and short-stepped gait with the torso and legs not properly in phase when placed in motion, to which may be added the feature of “magnetic” gait, where one or both feet appear to be stuck to the ground as the body moves forward. Probably the basal ganglia and their connections to the frontal lobes are involved in these cases. The steps are shortened to a shuffle and balance is precarious; with further deterioration, the patient can no longer walk or even stand. Cerebral paraplegia in flexion is the most advanced stage; the affected individual lies curled up in bed, unable even to turn over (see Chap. 7 for further discussion).
Damage to the cortices anterior to areas 6 and 8, i.e., areas 9, 10, 45, and 46, the prefrontal cortex, and also the anterior cingulate gyri, has less easily defined effects on motor behavior. The prefrontal cortex is heteromodal and has strong reciprocal connections with the visual, auditory, and somatosensory cortices. Of these, the visuomotor relationships are the most powerful. These frontal areas as well as the supplementary motor areas are involved in the planning and initiation of sequences of movement, as indicated in Chap. 4. In the monkey, for example, when a visual signal evokes movement, some of the prefrontal neurons become active immediately preceding the motor response; other prefrontal neurons are activated if the response is to be delayed. With prefrontal lesions on one side or the other, a series of motor abnormalities occur, for example, slight grasping and groping responses, a tendency to imitate the examiner’s gestures and to compulsively manipulate objects that are in front of the patient (imitation and utilization behavior described by Lhermitte [1983]), reduced and delayed motor and mental activity (abulia), motor perseveration or impersistence (with left and right hemispheric lesions, respectively), and paratonic rigidity on passive manipulation of the limbs (oppositional resistance, or gegenhalten).
Incontinence is another manifestation of frontal lobe disease. Right- or left-sided lesions involving the posterior part of the superior frontal gyrus, the anterior cingulate gyrus, and the intervening white matter result in a loss of control of micturition and defecation (Andrew and Nathan). There is no warning of fullness of the bladder or of the imminence of urination or bowel evacuation, and the patient is surprised at suddenly being wet or soiled. Less-complete forms of the syndrome are associated with frequency and urgency of urination during waking hours. The patient is embarrassed unless an element of indifference is added when the more anterior (nonmotor) parts of the frontal lobes are the sites of disease.
In the spheres of speech and language, a number of abnormalities other than Broca’s aphasia appear in conjunction with disease of the frontal lobes: laconic speech, lack of spontaneity of speech, telegrammatic speech (agrammatism), loss of fluency, perseveration of speech, a tendency to whisper instead of speaking aloud, and dysarthria. These are more prominent with left-sided lesions and are fully described in Chap. 23.
In general, when one speaks of cognitive and behavioral aspects of frontal lobe function, reference is made to the more anterior (prefrontal) parts rather than the motor and linguistic parts. These most recently developed parts of the human brain, called the “organ of civilization” by Halstead and repeated by Luria, have the most elusive functions.
The effects of lesions of the frontal lobes were nicely divulged by Harlow’s famous case of Phineas Gage, published in 1868; it has been the subject of numerous monographs ever since. His patient was a capable foreman of a railroad gang who became irreverent, dissipated, irresponsible, and vacillating (he also confabulated freely) following an injury in which an explosion drove a large iron-tamping bar into his frontal lobes. In Harlow’s words, “he was no longer Gage.” Another similarly dramatic example was Dandy’s patient (the subject of a monograph by Brickner), who underwent a bilateral frontal lobotomy during the removal of a meningioma. Feuchtwanger, in a clinical study of 200 cases of frontal lobe injury, was impressed most with the lack of initiative, changes in mood (euphoria), and inattentiveness, without intellectual and memory deficits. Rylander, in a classic monograph, described similar changes in patients with unilateral and bilateral frontal lobectomies (see later). Kleist (1934), under the heading of alogia, stressed the importance of loss of capacity for abstract thought, as shown in tests of analogies, proverbs, definitions, etc. In chimpanzees, Jacobsen observed that the removal of the premotor parts of the frontal lobes led to social indifference, tameness, placidity, forgetfulness, and difficulty in problem solving, findings that led Egas Moniz, in 1936, to perform prefrontal lobotomies on psychotic patients (see Damasio). This operation and its successor, prefrontal leukotomy (undercutting of the prefrontal white matter) reached their height of popularity in the 1940s and (tragically) provided the opportunity to study the effects of a wide range of frontal lobe lesions in a large number of patients.
The findings in patients who underwent frontal leukotomy have been the subject of endless controversy. Some workers claimed that there were few or no discernible effects of the operation, even with bilateral lesions. Others insisted that if the proper tests were used, a series of predictable and diagnostic changes in cognition and behavior could be demonstrated. The arguments pro and con and the inadequacies of many of the studies, both in methods of testing and in anatomic verification of the lesions (the extent and location of the lesions varied considerably, and this influenced the clinical effects), have been well summarized by Walsh. Admittedly, in patients who underwent bilateral frontal lobotomy, there was little if any impairment of memory function or of cognitive function as measured by intelligence tests, and certainly no loss of alertness and orientation. And some patients who had been disabled by schizophrenia, anxious depression, obsessive–compulsive neurosis, or a chronic pain syndrome did improve with respect to their psychiatric and pain symptoms. However, many were left with changes in personality, much to the distress of their families. They were indifferent to the feelings of others; gave no thought to the effects of their conduct; were tactless, distractible, and socially inept; and were given to euphoria and emotional outbursts. El-Hai has written a fascinating historical account of the procedure in the United States and a portrait of its main proponent at the time, Dr. Walter Freeman. Although no longer undertaken, the procedure must be viewed in the context of the understanding of, and limited options for, psychiatric disease at that time.
Luria (1973) had another interesting conception of the role of the frontal lobes in intellectual activity. He postulated that problem solving of whatever type (perceptual, constructive, arithmetical, psycholinguistic, or logical, definable also as goal-related behavior) proceeds in four steps: (1) the specification of a problem (in other words, a goal is perceived and the conditions associated with it are set); (2) formulation of a plan of action or strategy, requiring that certain linguistic activities be initiated in orderly sequence; (3) execution, including implementation and control of the plan; and (4) checking or comparing the results against the original plan to see if it was adequate.
Obviously, such complex psychologic activity must implicate many parts of the cerebrum and will suffer to some extent from a lesion in any of the parts that contribute to the functional system. Luria found that when the frontal lobes are injured, there was not only a general psychomotor slowing and easy distractibility but also an erroneous analysis of the above-listed conditions of the problem. “The plan of action that is selected quickly loses its regulating influence on behavior as a whole and is replaced by a perseveration of one particular link of the motor act or by the influence of some connection established during the patient’s past experience.” Furthermore, there was a failure to distinguish the essential sequences in the analysis and to compare the final solution with the original conception of the problem. Plausible as this scheme appears, like Goldstein’s “loss of the abstract attitude” (the patient thinks concretely, i.e., he reacts directly to the stimulus situation), such psychophysiologic analyses of the mental processes are highly theoretical, and the factors to which they refer are not easily measured.
Finally, a lesion that includes the frontal eye field may engender a type of reduced attention to the contralateral visual environment. This probably is the result of a defect in visually guided attention and it is seen only irregularly in clinical practice. The degree of neglect seen with a nondominant parietal lobe lesion is not observed with frontal lobe lesions, and it is difficult to differentiate the frontal defect from the simple impediment of being unable to direct gaze in one direction.
In modern parlance, the frontal lobe, particularly its prefrontal components, is said to exert an executive function, referring here to the overall control and sequencing of other cognitive functions. This allows for a type of self-monitoring that guides the selection of strategies to solve problems, the inhibition of incorrect responses, the ability to deal with change in focus and novelty in tasks, and probably to be able to generalize from experience. Indeed, all ability to adapt to changes in circumstance and to learn from experience requires this executive function. Unlike some of the psychic properties mentioned above, these are subject to measurement by testing and they are observable during the clinical examination as deterioration in problem solving, by repetitiousness and stereotypes, and by ineptitude in managing simple social situations. Probably, the trouble all individuals experience in maintaining a stream of thought when interrupted, a type of loss of attention, tests this function.
A lack of initiative and spontaneity is the most common effect of frontal lobe disease, and it is much easier to observe than to quantitate. With relatively mild forms of this disorder, patients exhibit an idleness of thought, speech, and action, and they lapse into this state without complaint. They are tolerant of most conditions in which they are placed, although they may act unreasonably for brief periods if irritated, seemingly unable to think through the consequences of their actions. They let members of the family answer questions and “do the talking,” interjecting a remark only rarely and unpredictably. Questions directed to such patients may evoke only brief, unqualified answers. Once started on a task, they may persist in it (“stimulus bound”); i.e., they tend to perseverate. Fuster, in his studies of the prefrontal cortex, emphasizes the failure over time to maintain events in serial order (impairment of temporal grading) and to integrate new events and information with previously learned data. Placidity is a notable feature of the behavior. Worry, anxiety, self-concern, hypochondriasis, complaints of chronic pain, and depression are all reduced by frontal lobe disease, as they were to some extent by frontal lobotomy.
Extensive and bilateral frontal lobe disease is accompanied by a quantitative reduction in all psychomotor activity. The number of movements, spoken words, and thoughts per unit of time diminish. Milder degrees of this state, associated with only a delay in responses, are called abulia as described earlier. The most severe degrees take the form of akinetic mutism wherein a nonparalyzed patient, alert and capable of movement and speech, lies or sits motionless and silent for days or weeks on end. It has been attributed to bilateral lesions in the ventromedial frontal regions or frontal-diencephalic connections (but focal lesions in the upper midbrain do the same). Laplane found that the lack of motivation of the patient with bifrontal lesions and bipallidal lesions to be the same, although one would expect the latter to manifest more as a bradykinesia than as a bradyphrenia (slowness of thinking).
The opposite state, in a sense, is a behavioral disinhibition that in extreme form becomes a hyperactivity syndrome, or “organic drivenness,” described by von Economo in children who had survived an attack of encephalitis lethargica. Disinhibition occurs largely with dorsolateral frontal lesions. In our patients, this syndrome has been produced most often by combined frontal and temporal lobe lesions, usually traumatic but also encephalitic, although exact clinicopathologic correlations could not be made. Such patients may also exhibit brief but intense involvement with some meaningless activity, such as sorting papers in an attic or hoarding objects or food. Possibly, compulsive behavior is related in some manner to this state and more particularly to lesions damaging the caudate-frontal connections. Combativeness and extreme insomnia or an otherwise disrupted sleep cycle are often part of the syndrome.
Pathological collecting behavior (hoarding) may be related to this type of drivenness and has been attributed to medial frontal lobe damage, including the cingulate gyri, by Anderson and colleagues based on a series of 13 patients. These patients, otherwise displaying mental clarity and despite negative personal and social consequences, collect massive amounts of useless items such as newspapers, junk mail, catalogs, food, clothing, and appliances, often encompassing several categories.
In addition to the disorders of initiative and spontaneity, frontal lobe lesions result in a number of other changes in personality and behavior. These, too, are easier to observe in the patient’s natural environment than to measure by psychologic tests. It has been difficult to find a term for all these personality changes. Some patients, particularly those with inferofrontal lesions, feel compelled to make silly jokes that are inappropriate to the situation, witzelsucht or moria; they are socially uninhibited and lack awareness of their behavior. The patient is no longer the sensitive, compassionate, effective human being that he once was, having lost his usual ways of reacting with affection and consideration to family and friends. In more advanced instances, there is an almost complete disregard for social conventions and an interest only in immediate personal gratification. The patient at the same time seems to lose an appreciation of the motivations and thought processes of other sapient persons (“theory of mind”); this results in the inability to incorporate these factors into his responses. These changes, observed characteristically in lobotomized patients, came to be recognized as too great a price to pay for the loss of anxiety, pain, depression, and “tortured self-concern,” hence the procedure became obsolete.
In general, the greatest cognitive-intellectual deficits relate to lesions in the dorsolateral parts of the prefrontal lobes and that the greatest personality, mood, and behavioral changes stem from lesions of the medial-orbital parts, although the two disorders often merge with one another. Benson (and Kleist and others before him) related the syndrome of apathy and lack of initiative to lesions in the dorsolateral frontal cortex, and a facetious, unguarded, and socially inappropriate state (see below) to orbital and medial frontal lesions. This distinction has held up only broadly in our experience. Some studies of penetrating brain injuries have reported an inconsistent but interesting relationship between left dorsal frontal lesions and anger with hostility, and right side orbitofrontal lesions, with anxiety and depression. Again, in clinical work, few lesions have this degree of localizability, making conclusions about emotional states somewhat uncertain.
Although the frontal lobes are the subject of a vast literature and endless speculation (see reviews of Stuss and Benson and of Damasio), a unified concept of their function has not emerged, probably because they are so large and include several heterogeneous systems. There is no doubt that the mind is greatly altered by disease of the prefrontal parts of the frontal lobes, but often it is difficult to say exactly how it is changed. Perhaps at present it is best to regard the frontal lobes as the part of the brain that quickly and effectively orients and drives the individual, with all the percepts and concepts formed from past life experiences, toward action that is projected into the future.
These are of particular value in establishing the presence of frontal lobe disease and are generally constructed to detect the ability to persist in a task and the opposite, to switch mental focus on demand. They include the Wisconsin card-sorting test, the Stroop color-naming test, sequencing of pictures, “trail making” (a two-part test in which the patient draws lines, first connecting randomly arrayed numbers on a paper in order and then connecting numbers and letters that correspond in order), the verbal equivalent of trail making, and the “go-no-go” test, both of which are used regularly in the mental status examination (see below), and the three-step hand posture test of Luria. The alphabet-number verbal trailmaking test requires the patient to give each letter of the alphabet followed by the corresponding number (A-1, B-2, C-3, etc.). In the Luria test and its variants, the patient is, for example, asked to imitate, then reproduce, a sequence of three hand gestures, typically making a closed fist, holding the open hand on its side, and then opening an outstretched palm. Patients with frontal lesions on either or both sides have difficulty performing the test in correct sequence, often perseverating, balking, or making unwanted gestures. Luria suggested testing this with the sequence of arm thrusting forward, clenching the fist, and forming a ring with the first two fingers—derivatives of this test are now used. He also pointed out (Luria, 1969) that the natural kinetic “melody,” or smoothness of transition from one hand position to the next is disrupted and there is a tendency to perseverate. This has been termed “kinetic limb apraxia” by some behavioral neurologists.
It should be kept in mind that similar impairments of performance may occur with all manner of confusional and inattentive states so that no conclusion can be made if the patient is less than fully attentive. More complex mental acts that may be easily tested and betray frontal lobe disease but are less specific, in that they are also disordered by lesions in other brain regions, include serial subtraction (“working memory”), interpretation of proverbs, tests of rapid motor response, and others.
Effects of frontal lobe disease may be summarized as follows:
Effects of unilateral frontal disease, either left or right
Contralateral spastic hemiplegia
Contralateral gaze paresis
Apathy and loss of initiative or its opposite, slight elevation of mood, increased talkativeness, tendency to joke inappropriately (witzelsucht), lack of tact, difficulty in adaptation
If entirely prefrontal, no hemiplegia; but grasp and suck reflexes or instinctive grasping may be released
Anosmia with involvement of orbital parts
Effects of right frontal disease
Left hemiplegia
Changes as in I.B, C, and D
Effects of left frontal disease
Right hemiplegia
Broca’s aphasia with agraphia, with or without apraxia of the lips and tongue (see Chap. 23)
Sympathetic apraxia of left hand (see “Apraxia” in Chap. 3)
Changes as in I.B, C, and D
Effects of bifrontal disease
Bilateral hemiparesis
Spastic bulbar (pseudobulbar) palsy
If prefrontal, abulia or akinetic mutism, lack of ability to sustain attention and solve complex problems, rigidity of thinking, bland affect, social ineptitude, behavioral disinhibition, inability to anticipate, labile mood, and varying combinations of grasping, sucking, obligate imitative movements, utilization behavior
Decomposition of gait and sphincter incontinence
Syndromes Caused by Lesions of the Temporal Lobes
The sylvian fissure separates the superior surface of each temporal lobe from the frontal lobe and anterior parts of the parietal lobe. There is no natural anatomic boundary between the temporal lobe and the occipital or the parietal lobe but the angular gyrus serves as a landmark for the latter. Figure 22-1 indicates the boundaries of the temporal lobes. The inferior branch of the middle cerebral artery supplies blood to the convexity of the temporal lobe, and the temporal branch of the posterior cerebral artery supplies the medial and inferior aspects, including the hippocampus.
The temporal lobe includes the superior, middle, and inferior temporal, lateral occipitotemporal, fusiform, lingual, parahippocampal, and hippocampal convolutions and the transverse gyri of Heschl. The last of these constitutes the primary auditory receptive area and is located within the sylvian fissure. It has a tonotopic arrangement: fibers carrying high tones terminate in the medial portion of the gyrus and those carrying low tones, in the lateral and more rostral portions (Merzenich and Brugge). The planum temporale (area 22), an integral part of the auditory cortex, lies immediately posterior to the Heschl convolutions, on the superior surface of the temporal lobe. The left planum is larger in right-handed individuals. There are rich reciprocal connections between the medial geniculate bodies and the Heschl gyri. These gyri project to the unimodal association cortex of the superior temporal gyrus, which, in turn, projects to the paralimbic and limbic regions of the temporal lobe and to temporal and frontal heteromodal association cortices and the inferior parietal lobe. There is also a system of fibers that project back to the medial geniculate body and to lower auditory centers. The cortical receptive zone for labyrinthine impulses is less well demarcated than the one for hearing but is probably situated on the inferior bank of the sylvian fissure, just posterior to the auditory area. Least well delimited is the role of the medial parts of the temporal lobe in olfaction and gustatory perception, although seizure foci in the region of the uncus (uncinate seizure) often excite hallucinations of these senses.
The middle and inferior temporal gyri (areas 21 and 37) receive a massive contingent of fibers from the striate cortex (area 17) and the parastriate visual association areas (areas 18 and 19). These temporal visual areas make abundant connections with the medial limbic, rhinencephalic (olfactory), orbitofrontal, parietal, and occipital cortices, allowing for an intimate interconnection between the cortices subserving vision and hearing.
The superior part of the dominant temporal lobe is concerned with the acoustic or receptive aspects of language, as discussed in Chap. 23, which is devoted to this subject. The middle and inferior convolutions are sites of visual discriminations; they receive fiber systems from the striate and parastriate visual cortices and, in turn, project to the contralateral visual association cortex, the prefrontal heteromodal cortex, the superior temporal cortex, and the limbic and paralimbic cortex. Presumably, these systems subserve such functions as spatial orientation, estimation of depth and distance, stereoscopic vision, and hue perception. Similarly, the unimodal auditory cortex is closely connected with a series of auditory association areas in the superior temporal convolution, and the latter are connected with prefrontal and temporoparietal heteromodal areas and the limbic areas (see Mesulam, 1998). Most of these auditory connections have been worked out in the macaque but the limited number of well-studied lesions in patients suggests that they are also involved in complex verbal and nonverbal auditory discriminations in humans.
The most important functions of the hippocampus and other structures of the hippocampal formation (dentate gyrus, subiculum, entorhinal cortex, and parahippocampal gyrus) are learning and memory, already discussed in Chap. 21. There is an abundance of connections between the medial temporal lobe and the entire limbic system. For this reason, MacLean referred to these parts as the “visceral brain,” and Williams, as the “emotional brain.” Also included in this anatomic concept are the hippocampus, the amygdaloid nuclei, the fornices and limbic portions of the inferior and medial frontal regions, the cingulate cortices, and the septal and associated subcortical nuclei referred to as the limbic system (see Chap. 25).
Most of the temporal lobe cortex, including Heschl gyri, has nearly equally developed pyramidal and granular layers. In this respect, it resembles more the granular cortex of the frontal and prefrontal regions and inferior parts of the parietal lobes. Unlike the six-layered neocortex, the hippocampus and dentate gyrus are typical of the phylogenetically older three-layered allocortex.
A massive fiber system projects from the striate and parastriate zones of the occipital lobes to the inferior and medial parts of the temporal lobes. The temporal lobes are connected to one another through the anterior commissure and middle part of the corpus callosum; the inferior or uncinate fasciculus connects the anterior temporal and orbital frontal regions. The arcuate fasciculus connects the posterosuperior temporal lobe to the motor cortex and Broca area.
Physiologically, the temporal lobe is an integrator of “sensations, emotions, and behavior” in so far as it relates the organism’s sensory experiences to emotional meaning thought it’s proximity to the limbic system. Similar integrative mechanisms are operative in the parietal lobe, but only in the temporal lobe are they brought into close relationship to one’s instinctive and emotional life. Self-awareness also requires a coherent and sequential stream of thought. Where the inner “stream of thought” (William James’ term for constant thinking) is perceived is still an open question. Given the requirement that it be close to other integrated sensory experiences and that it incorporate the temporal lobe functions of both language and memory, a locus in the temporal lobes seems likely. Some hint of the role of the temporal lobe in our personal and emotional lives was suggested by Hughlings Jackson in the nineteenth century, derived from his insightful analysis of the psychic states accompanying temporal lobe seizures. Later, the observations of Penfield and his collaborators on the effects of stimulating the temporal lobes in the conscious patient undergoing surgical correction of epilepsy revealed something of its complex functions. The seminal writings on this subject include Williams’ chapter on temporal lobe syndromes in the Handbook of Clinical Neurology and the monographs by Penfield and Rasmussen (The Cerebral Cortex of Man) and by Alajouanine and colleagues (Les Grandes Activités du Lobe Temporale).
The symptoms that arise as a consequence of disease of the temporal lobes may be divided into disorders of: (1) special senses (visual, auditory, olfactory, and gustatory), (2), language, (3) memory and time perception, (4) emotion, and behavior. Of central importance also are the roles of the superior part of the dominant (usually left) temporal lobe in language and handedness. Several of these functions and their derangements are of such scope and importance that they are accorded separate chapters. Language is discussed in Chap. 23, memory in Chap. 21, and the neurology of emotion and behavior in Chap. 25; these subjects are omitted from further discussion here.
In Chap. 13 (on vision), it was pointed out that lesions of the white matter of the central and posterior parts of the temporal lobe characteristically involve the lower arching fibers of the geniculocalcarine pathway (Meyer loop). This results in an upper homonymous quadrantanopia, usually not perfectly congruent. However, there is considerable variability in the arrangement of visual fibers as they pass around the temporal horn of the lateral ventricle, accounting for the smallness of the field defect in some patients after temporal lobectomy or stroke and extension into the inferior field in others. Quadrantanopia from a dominant (left-sided) lesion is often combined with aphasia.
Bilateral lesions of the temporal lobes render a monkey psychically blind. It can see and pick up objects but does not recognize them until they are explored orally. Natural emotional reactions such as fear are lost. This syndrome, named for Klüver and Bucy, has been identified only in partial form in humans. Using special tests, lesser degrees of visual imperception were uncovered in patients by Milner (1971) and by McFie and colleagues. This syndrome is further discussed in Chap. 25.
Visual hallucinations of complex form, including ones of the patients himself (autoscopy), appear during temporal lobe seizures. Penfield was able to induce what he called “interpretive illusions” (altered impressions of the present) and to reactivate past experiences completely and vividly in association with their original emotions. Temporal lobe abnormalities may also distort visual perception; seen objects may appear too large (macropsia) or small (micropsia), too close or far away, or unreal. Some visual hallucinations have an auditory component: an imaginary figure may speak and move and, at the same time, arouse intense emotion in the patient. The entire experience may seem unnatural and unreal to the patient.
Bilateral lesions of the transverse gyri of Heschl, while rare, are known to cause a central deafness. Henschen
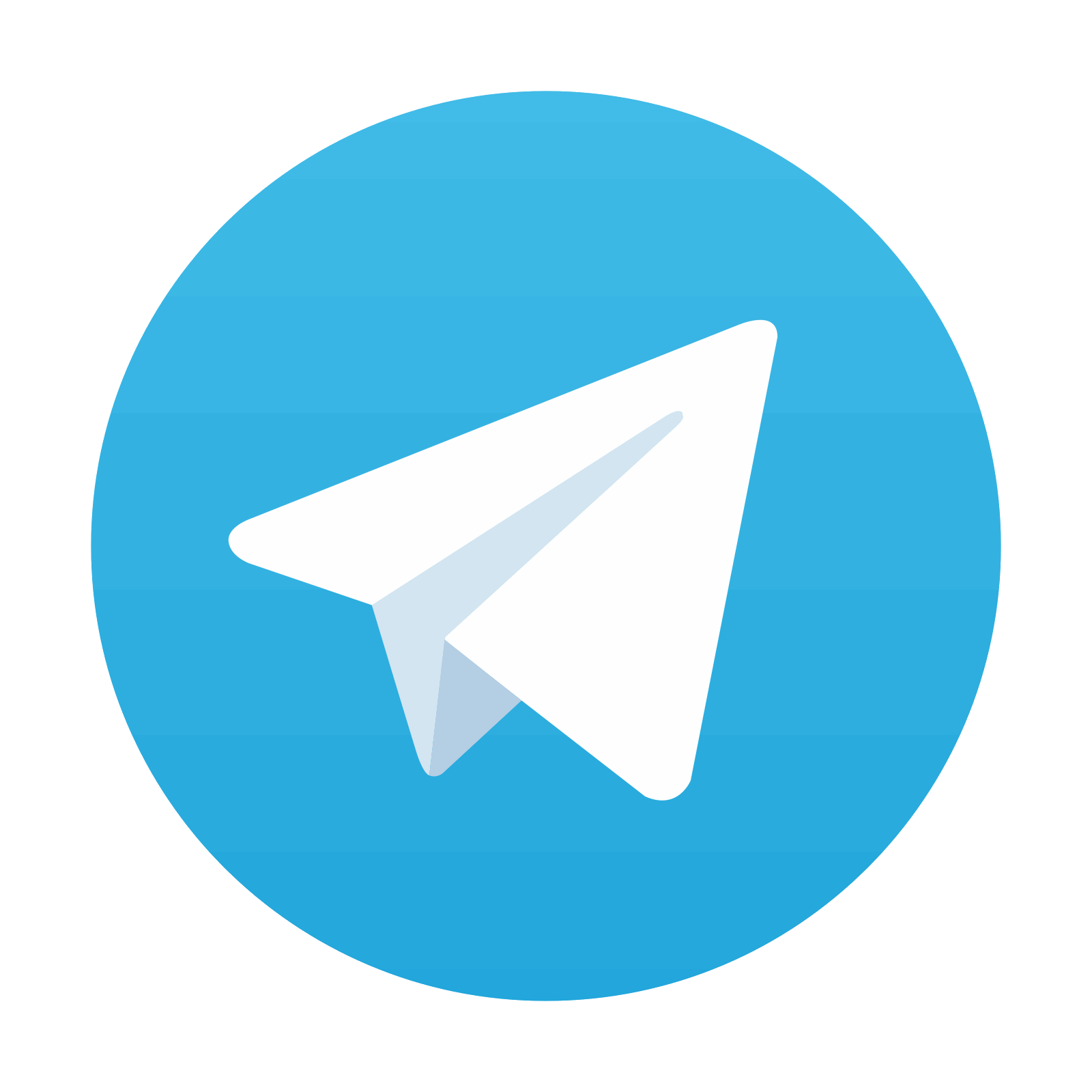
Stay updated, free articles. Join our Telegram channel

Full access? Get Clinical Tree
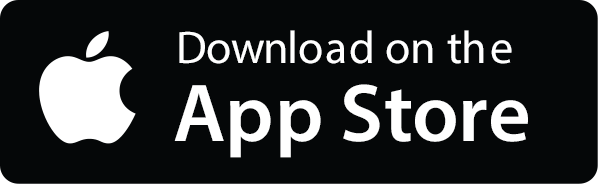
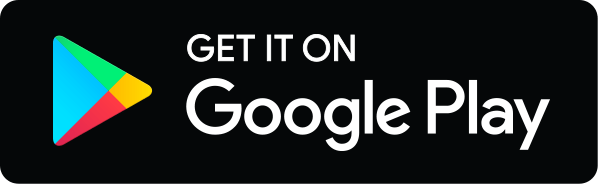
