Abstract
Purine metabolism encompasses the metabolic pathways involved in the synthesis, interconversion, salvage, and degradation of purine-based nucleosides and nucleotides. These metabolic pathways are involved in many essential cellular processes, including energy transfer, oxidative phosphorylation, synthesis of DNA and RNA, and signal transduction. A nucleoside is a nitrogenous base linked to a 5-carbon sugar (either ribose or deoxyribose). For purines, this nitrogenous base is either adenine, guanine, or hypoxanthine. When nucleosides are covalently linked to one or more phosphate groups, they are referred to as nucleotides.
Purine Metabolism
Purine metabolism encompasses the metabolic pathways involved in the synthesis, interconversion, salvage, and degradation of purine-based nucleosides and nucleotides. These metabolic pathways are involved in many essential cellular processes, including energy transfer, oxidative phosphorylation, synthesis of DNA and RNA, and signal transduction. A nucleoside is a nitrogenous base linked to a 5-carbon sugar (either ribose or deoxyribose). For purines, this nitrogenous base is either adenine, guanine, or hypoxanthine. When nucleosides are covalently linked to one or more phosphate groups, they are referred to as nucleotides.
In the purine biosynthetic (“de novo”) pathway, the high-energy compound phosphoribosyl pyrophosphate (PRPP) is first converted to inosine monophosphate (IMP) in 10 steps (Figure 27.1). Subsequently, IMP can be interconverted to either adenosine monophosphate (AMP) or guanosine monophosphate (GMP). These ribonucleotide monophosphates may be converted to their di- and triphosphate counterparts by nucleoside mono- and diphosphate kinase, to form adenosine diphosphate (ADP) and adenosine triphosphate (ATP) as well as guanosine diphosphate (GDP) and guanosine triphosphate (GTP), respectively. The deoxyribonucleotides, i.e. deoxyadenosine diphosphate (dADP) and deoxyguanosine diphosphate (dGDP), can be formed by converting the ribose of ADP and GDP to deoxyribose. This reaction is catalyzed by the enzyme ribonucleotide reductase.
Figure 27.1 Overview of purine metabolism. Abbreviations: 5′NT, 5′-nucleotidase; AICAR, aminoimidazolecarboxamide ribotide; ADA, adenosine deaminase; AK, adenosine kinase; ADP, adenosine diphosphate; ADSL, adenylosuccinate lyase; AMP, adenosine monophosphate (or adenylic acid); AMPD, adenylate deaminase; AMPRT, amidophosphoribosyltransferase; APRT, adenine phosphoribosyltransferase; ASS, adenylosuccinate synthetase; ATIC, AICAR transformylase–IMP cyclohydrolase; ATP, adenosine triphosphate; FAICAR, 5-formamidoimidazole-4-carboxamide ribotide; GA, guanase; GDP, guanosine diphosphate; GMP, guanosine monophosphate (or guanylic acid) ; GR, GMP reductase; GS, GMP synthase; GTP, guanosine triphosphate; HGPRT, hypoxanthine–guanine phosphoribosyltransferase; IDH, IMP dehydrogenase; IMP, inosine monophosphate (or inosinic acid); NDK, nucleoside diphosphate kinase; NMK, nucleoside monophosphate kinase; PPi, inorganic pyrophosphate; PRPP, phosphoribosyl pyrophosphate; PRS, PRPP synthetase; RR, ribonucleotide reductase; SAICAR, succinyl-aminoimidazolecarboxamide ribotide; S-AMP, succinyl-AMP or adenylosuccinate; XMP, xanthine monophosphate or xanthylic acid; XOR, xanthine oxidoreductase.
In purine catabolism, the nucleotides are hydrolyzed and phosphorolyzed to their nucleosides, and ultimately converted to xanthine, which is oxidized to uric acid (Figure 27.1). For salvaging purine bases, two phosphoribosyltransferases catalyze the transfer of a ribose-5-phosphate from PRPP to the base, yielding the respective nucleotide. As such, adenine phosphoribosyltransferase (APRT) salvages adenine to AMP, while hypoxanthine–guanine phosphoribosyltransferase (HGPRT) regenerates IMP and GMP from hypoxanthine and guanine, respectively (Figure 27.1). In humans, adenine is only produced in small amounts, as AMP and adenosine are catabolized mainly through interconversion to IMP first. Therefore, HGPRT is also important in the recycling of adenine nucleosides.
Purine Functions
Purines are key players in multiple fundamental cellular processes that are ubiquitously present in cells of the human body. For example, the adenine-based nucleotide ATP is the principal cellular carrier of free energy and is essential for muscle contraction and other cellular movements, active transport of molecules, and biosynthesis of (macro-) molecules from simple precursors [1]. It should be noted, however, that in addition to being synthesized and salvaged through the metabolic pathways described above, ATP is primarily synthesized during cellular respiration. Second, the purinergic ribonucleotides (i.e. ATP and GTP) and deoxyribonucleotides (i.e. dATP and dGTP) are two of the four substrates for RNA and DNA synthesis, respectively. Third, GTP and GDP are essential in signal transduction, particularly pathways involving G proteins coupled with a cell-membrane-bound receptor [2]. In this mechanism, binding of a ligand to the G-protein-coupled receptor complex triggers an allosteric change in the G protein, causing a bound GDP to be replaced by GTP. Subsequently, the GTP activates the alpha subunit of the G protein, causing it to dissociate from the G protein and act as a downstream effector. A final generic function of purines has been proposed for the purine breakdown product, uric acid, which may act as an endogenous antioxidant.
Obviously, these universal purine functions are important for the nervous system as well. For example, the brain’s energy demand is disproportionally high, and therefore ATP consumption is large [3, 4]. In addition, many different G-protein-coupled receptors regulate synaptic communication, and the antioxidant uric acid may be relevant in brain damage. But in addition to these more common functions, purines play also very specific roles in the nervous system, as adenine nucleotides and nucleosides mediate intercellular signaling [5]. As such, ATP has been identified as a co-neurotransmitter in both sympathetic and parasympathetic nerves. Moreover, multiple purinergic receptor subtypes are widely distributed throughout the central nervous system, both in neurons and glia. Four G-protein-coupled receptors for adenosine have been recognized, as well as multiple ATP receptors that include both classic cationic ligand-operated channels for Na+, K+, and Ca2+ and G-protein-coupled receptors. Over the last decades, it has become clear that these purinergic signaling pathways are involved in many functional aspects of the nervous system, including the interaction between neuronal and glial networks, neural development and plasticity, regeneration, as well as specialized sensory mechanisms in the retina, inner ear, olfactory and taste system, and in pain transmission [5]. Consequently, purinergic pathways have been associated with multiple aspects of behavior, including feeding, locomotion, sleep, mood, and motivation.
Neurological Phenotypes in Disorders of Purine Metabolism
Enzyme defects in purine metabolism are known to be associated with clinical disorders that often involve neurological dysfunction. However, there does not appear to be a general neurological phenotype associated with purine disorders, as – depending on the specific enzyme that is deficient, and sometimes also on residual enzyme activity – both central and peripheral nervous systems may be involved, or muscle function, to a variable degree. Perhaps the most particular clinical phenotype associated with a purine disorder is Lesch–Nyhan disease (LND). LND patients suffer from a movement disorder dominated by dystonia, specific cognitive deficits, and behavioral abnormalities including self-injury. LND is caused by a deficiency of the purine salvage enzyme HGPRT due to a mutation in the HPRT1 gene. HPRT1 was among the first genes to be cloned for any human disease, and it is one of the most intensively studied loci in human genetics. Over the last decades, it has become apparent that the characteristic motor and behavioral abnormalities in LND are due to dysfunction of specific brain circuitry, associated with abnormal development of the brain’s dopamine system. The resultant complex clinical phenotype of LND often imposes a challenge on clinical management.
In this section, the other monogenetic purinergic disorders where neurological manifestations are part of the clinical phenotype, are briefly summarized first (Table 27.1). Several of these disorders have been reviewed more extensively elsewhere [6–8]. In the following sections, the clinical spectrum, current understanding of the pathogenesis, clinical diagnosis and work-up, as well as possible treatment strategies of HGPRT deficiency, are discussed in more detail.
Primary defect | Key metabolic effects | Key neurological symptoms | Other symptoms |
---|---|---|---|
PRPP synthetase (PRS) deficiency |
|
| Recurrent respiratory infections in Arts syndrome |
PRPP synthetase (PRS) superactivity | Uric acid ↑ | Developmental delay/intellectual disability, hypotonia, and sensorineural deafness | Gout, urolithiasis, nephropathy, urinary tract calculi, and gouty arthritis |
Adenylosuccinate lyase (ADSL) deficiency |
| Microcephaly, developmental delay/intellectual disability, epilepsy, autistic features, hypotonia | Secondary feeding problems |
AICAR transformylase–IMP cyclohydrolase (ATIC) deficiency |
| Psychomotor delay, congenital blindness, epilepsy | Dysmorphic features |
Deoxyguanosine kinase (dGK) deficiency | Nystagmus, cerebral atrophy, microcephaly, hypotonia | Liver dysfunction | |
Myoadenylate deaminase (mAMPD) deficiency |
| Myalgia, muscle cramps, exercise intolerance; often asymptomatic | |
Adenosine deaminase (ADA) deficiency |
| Neurobehavioral difficulties including attention deficits, hyperactivity, aggression and social problems, as well as sensorineural deafness | Severe combined immunodeficiency disease (SCID) |
Purine nucleoside phosphorylase (PNP) deficiency |
| Variable phenotype, ranging from severe psychomotor retardation to mild motor or cognitive abnormalities | Severe deficiency of cellular immunity |
Hypoxanthine guanine phosphoribosyltransferase (HGPRT) deficiency | Uric acid ↑ | Dystonia, cognitive impairments, self-injury | Gout, urolithiasis nephropathy, urinary tract calculi, and gouty arthritis |
Phosphoribosyl Pyrophosphate Synthase 1 Deficiency and Superactivity
PRS-1 (EC 2.7.6.1), one of three PRPP synthetases in humans, is encoded by the PRPS1 gene. PRS-1 catalyzes the first step of nucleotide synthesis, i.e. the formation of PRPP from ATP and ribose-5-phosphate.
Deficiency and superactivity of PRS-1 may result in different clinical syndromes that appear to depend on residual enzyme activity, with an X-linked recessive inheritance [9]. Overall, the mutations resulting in the severest symptoms are predicted to have the most impact on the protein structure. Mutations in the PRPS1 gene that lead to a complete absence of PRS-1 activity have been linked with Arts syndrome, characterized by developmental delay, intellectual disability, early-onset hypotonia, ataxia, hearing impairment, and optic atrophy. In addition, PRPS1 mutations that cause a partial reduction in PRS-1 activity have been linked with X-linked sensorineural deafness (DFNX1) and X-linked recessive Charcot–Marie–Tooth disease-5 (CMTX5). DFNX1 patients have mild to moderate hearing impairment, or prelingual-onset hearing loss. CMTX5 patients have peripheral sensorimotor neuropathy, sensorineural hearing loss and optic neuropathy. Finally, mutations in the PRPS1 gene that increase the activity of PRS-1, due to the lack of regulatory control, lead to hyperuricemia and sensorineural hearing loss, with or without intellectual disability to varying degrees.
It has been hypothesized that the clinical features due to PRPS1 mutations primarily result from reduced levels of GTP and possibly other purine nucleotides including ATP. However, the neurological manifestations linked with PRS may result from, perhaps additional, alterations in non-purine pathways, as PRPP is an important cofactor in pyrimidine and pyridine nucleotide metabolism as well.
Adenylosuccinate Lyase Deficiency
ADSL (EC 4.3.2.2) deficiency, with autosomal-recessive inheritance, is caused by a mutation in the ADSL gene that often causes enzyme instability [10]. ADSL catalyzes two steps in the purine biosynthetic pathway, as it converts succinyl-aminoimidazolecarboxamide ribotide (SAICAR) into aminoimidazolecarboxamide ribotide (AICAR), as well as adenylosuccinate (S-AMP) into AMP (Figure 27.1). The metabolic hallmark of ADSL deficiency is the accumulation of the succinyl purines SAICA-riboside and succinyladenosine (S-Ado) in the cerebrospinal fluid and urine, i.e. the dephosphorylated substrates of the enzyme.
The clinical presentations of ADSL deficiency are diverse [7], and their severity tends to correlate with residual activity. Patients may have intrauterine growth impairment, microcephaly, developmental delay, intellectual disability, epilepsy, autistic features, hypotonia, feeding problems, and occasional growth retardation with muscle wasting. Rarely, patients are less intellectually impaired and may display only delayed motor development with hypotonia, high-functioning autism with epilepsy, or a behavioral phenotype resembling Angelman syndrome. The prognosis of ADSL-deficient patients is variable, ranging from early death to satisfactory performance in a protected environment.
The exact mechanism by which ADSL deficiency leads to neurobehavioral dysfunction remains uncertain, although it has been hypothesized that neurotoxic effects of accumulating succinyl purines are an important etiological factor.
Myoadenylate Deaminase Deficiency
In humans, three genes have been identified that encode AMP deaminase (AMPD, EC 3.5.4.6) that catalyzes the irreversible deamination of AMP to IMP, freeing ammonia (NH3). Deficiency of myoadenylate deaminase (mAMPD) is an inherited autosomal-recessive disorder caused by mutations in the AMPD1 gene, the isoform predominantly involved in skeletal muscle purine metabolism [11].
The vast majority of individuals with mAMPD deficiency are asymptomatic, but non-progressive exercise intolerance accompanied by myalgia and cramping that begins in late childhood or adulthood may occur [7]. Serum creatine kinase can be increased, sometimes only after exercise. Electromyography may be normal. Mutations in the AMPD1 gene may coincide with other genetic metabolic deficiencies, having a synergistic effect on myopathic symptoms.
The myopathic symptoms are thought to arise from interruption of the purine nucleotide cycle between AMP and IMP. During exercise, the flux through this metabolic cycle is increased in skeletal muscle. mAMPD deficiency is thought to disturb one or more of the proposed functions of this cycle, including maintaining the myocytic adenylate energy charge, enhancing the glycolysis rate, providing citric acid cycle intermediates, and preserving the IMP pool to replenish ATP during recovery [12].
Deficiency of mAMPD is often diagnosed with a forearm lactate-ammonia blood test, in which skeletal muscle does not accumulate NH3 as occurs in normal subjects. Additionally, histochemical staining of biopsied skeletal muscle, genotyping, and enzyme assay can also be used for diagnosis or confirmation.
There is currently no reliable effective treatment for symptomatic mAMPD deficiency. Avoidance of triggers such as excessive exercise is generally recommended.
Other Purine Enzyme Deficiencies with Neurological Sequelae
Several other, often very rare, purine enzyme deficiencies have been reported, for which the neurological manifestations are less well characterized (Table 27.1).
First, purine nucleoside phosphorylase (PNP, EC 2.4.2.1) deficiency is a rare autosomal-recessive immunodeficiency disorder, with decreased T-cell function. Approximately half of the patients exhibit neurological features with a broad range of severity, ranging from severe psychomotor retardation to mild motor or cognitive abnormalities.
Second, adenosine deaminase (ADA, EC 3.5.4.4) deficiency most often presents with severe combined immunodeficiency, with lethal infections in infancy or early childhood. Children who survive may develop neurobehavioral difficulties such as attention deficits, hyperactivity, aggression and social problems, as well as bilateral sensorineural hearing loss.
Third, deficiency of the mitochondrial enzyme deoxyguanosine kinase (DGK, EC 2.7.1.113) causes a severe form of mitochondrial DNA depletion. Patients typically present with liver failure, and have severe psychomotor retardation with nystagmus, cerebral atrophy, microcephaly, and hypotonia. Fatal multi-organ system failure is common in the first year of life.
Fourth, AICAR transformylase (EC 2.1.2.3) and IMP cyclohydrolase (EC 3.5.4.10) enzymes are present in one protein (ATIC). ATIC deficiency is associated with intellectual deficiency, epilepsy, dysmorphic features, and congenital blindness. The enzyme’s substrate AICAR and its dephosphorylated form, AICA-riboside, are increased in patients’ cells and urine, respectively.
Finally, mutations in the gene encoding IMP dehydrogenase (IDH, EC 1.1.1.205) have been linked with retinitis pigmentosa type 10 and some cases of Leber congenital amaurosis.
The Clinical Spectrum of the HGPRT-Deficient Phenotype
HGPRT deficiency is associated with a phenotypic continuum (Table 27.2). Classic LND patients exhibit the full phenotype with uric acid overproduction, neurological, cognitive, and behavioral features. Some patients display a Lesch–Nyhan variant (LNV) phenotype characterized by overproduction of uric acid, with or without neurological dysfunction, but no self-injurious behavior (Table 27.2). Although the eponym Kelley–Seegmiller syndrome has been applied to LNV, this term is probably best avoided as its definition is unclear and it suggests another disease entity, but is merely a variant of LND.
In HGPRT deficiency, the overproduction of uric acid results in hyperuricemia and an increased urinary uric acid excretion [13]. The hyperuricemia may cause subsequent problems, as beyond the limit of solubility, uric acid may precipitate. In the urogenital system, this may result in nephrolithiasis with subsequent urinary obstruction and renal failure. In addition, uric acid crystal deposition in the joints can cause gouty arthritis, and in subcutaneous tissues it forms tophi.
The least severe form of HGPRT deficiency is characterized by the overproduction of uric acid and associated problems only, i.e. without clinically overt neurological or behavioral abnormalities. This mild phenotype is most often designated as HGPRT-related hyperuricemia (HRH, Table 27.2) [14]. It should be noted that these patients often have minor motor clumsiness or mild cognitive difficulties that may go unnoticed without specific neurological or psychometric testing.
Table 27.2 Spectrum of disease in HGPRT deficiency, in relation to HGPRT activity*
HRH | HND | LND | |
---|---|---|---|
Clinical features: | |||
Hyperuricemia | Yes | Yes | Yes |
Motor disorder | None | Variable degree | Yes |
Cognitive problems | None | Variable degree | Yes |
Self-injury | None | None | Yes |
Mean HGPRT activity | 12% | 7% | <1% |
* Abbreviations: HND, HGPRT-related neurological disease; HRH, HGPRT-related hyperuricemia; LND Lesch–Nyhan disease.
Movement Disorder in HGPRT Deficiency
The neurobehavioral phenotype of classic LND includes a characteristic motor syndrome with only minor phenotypic variability. The most prominent feature of the motor syndrome, universally present in all patients, is a generalized action dystonia (Figure 27.2) [15, 16]. Dystonia of the upper limbs usually prevents their functional use for activities of daily living, and patients use wheelchairs because lower limb dysfunction prohibits walking or standing unassisted. Some patients have severe opisthotonus or truncal arching. Although dystonia is universal and always the most severe extrapyramidal disorder in LND, many patients also have other extrapyramidal movement disorders, including choreoathetosis or ballismus (Figure 27.2). In virtually all patients, these motor features are superimposed on hypotonia when fully relaxed [15]. Corticospinal signs may sometimes occur, such as pathological reflexes or spasticity, but they are typically mild and often limited to the legs.
Figure 27.2 The movement disorder associated with HGPRT deficiency. The prevalence of both extrapyramidal and pyramidal signs in patients with classic LND and those with partial enzyme defects (LNV) are expressed as a percentage of the patient population studied. Data from Jinnah HA, Ceballos-Picot I, Torres RJ, Visser JE, Schretlen DJ, Verdu A, et al. Attenuated variants of Lesch-Nyhan disease. Brain. 2010;133(Pt 3):671–89; and from Jinnah HA, Visser JE, Harris JC, Verdu A, Larovere L, Ceballos-Picot I, et al. Delineation of the motor disorder of Lesch–Nyhan disease. Brain. 2006;129(Pt 5):1201–17.
If hyperuricemia exists along with some degree of neurological dysfunction and/or cognitive deficits, hence without self-injury, patients are described as having HGPRT-related neurological disease (HND, Table 27.2) [14]. The motor features of HND can vary among these partial phenotypes, from mild clumsiness to severe handicap similar to classic LND.
Non-Motor Features in HGPRT Deficiency
Most LND patients also have a mild or moderate intellectual disability. Reliable cognitive testing is challenging in this population, due to the motor handicap, behavioral disorder with variable cooperation, and usually limited education. With these challenges in mind, IQ scores have been estimated to fall in the 60–80 range. The most affected domains are attention and mental flexibility [17, 18]. Significant generalized cognitive impairment is not an invariable characteristic of LND, and severe intellectual deficiency is uncommon.
In HND patients, some cognitive impairment may be present that is usually less severe than in classic LND patients. The majority of HND patients have some type of learning impairment, and difficulties with attention – similar to LND – is usually the most prominent problem.
The neurobehavioral phenotype of LND includes recurrent severe self-injurious behavior, such as self-biting, self-hitting, eye poking, and others [19, 20]. This behavior is universal in LND, and considered a hallmark of the disease (Figure 27.3). Self-injurious behavior usually emerges before 4 years of age, but may not be present before the second decade of life. In addition to self-injury, patients may exhibit other difficult behaviors, such as spitting at or hitting others, use of foul language, or oppositional defiant behavior. The nature of these disinhibited behaviors is enigmatic, but appears different from purposeful aggression, as patients often apologize afterwards.
Figure 27.3 Self-injury in LND. Characteristic self-inflicted tissue damage to the lip (a) and the fingers (b) in LND patients. The challenging management of self-injury in LND requires covering any hard surfaces or sharp parts of the wheelchair with soft padding, in combination with protective restraints to prevent hitting or biting. (c) Reproduced with permission from Visser JE, Bar PR, Jinnah HA. Lesch–Nyhan disease and the basal ganglia. Brain Res Brain Res Rev. 2000;32(2–3):449–75.
By definition, HND patients do not exhibit self-injurious behavior. However, they may have impulsivity and other undesirable behaviors.
Pathogenesis of LND
Genetic Background
LND was the first neurogenetic disorder for which the responsible gene, HPRT1, located in the q26-27 region on the X chromosome, was identified [21]. Since then, many different HPRT1 mutations causing disease have been reported, and these may appear throughout the gene. The mutations are heterogeneous, including missense, nonsense and splicing mutations, deletions, insertions, partial duplications, non-coding regulatory mutations, and more complex changes [22]. The list of known mutations in the HPRT1 gene is maintained at www.lesch-nyhan.org. It is generally believed that the majority of mutations occur de novo, since classic LND males do not reproduce.
Several comprehensive studies of genotype–phenotype correlations suggest that the most relevant factor for disease severity is the effect of the HPRT1 mutation on the residual HGPRT enzyme activity, rather than specific mutation types or sites [22, 23]. As such, less severe clinical manifestations result from mutations predicted to allow some degree of residual enzyme function. These less severely affected patients with HGPRT deficiency typically have the LNV (i.e. HRH or HND) phenotype, rather than the more severe classic LND phenotype.
Biochemical Background
HGPRT drives the recycling of hypoxanthine and guanine into their respective nucleotide pools (Figure 27.1), using the high-energy phosphate bonds of PRPP to catalyze the reaction. HGPRT pyrophosphorylates hypoxanthine into IMP, the central precursor for both adenine and guanine nucleotides, and also guanine directly into GMP. In the absence of HGPRT, hypoxanthine and guanine are degraded to uric acid. The reduced purine salvage, together with an accompanying activation of de novo purine synthesis, causes the uric acid overproduction. The subsequent hyperuricemia, if untreated, is the cause of the nephrolithiasis, gouty arthritis and tophi that is seen in HGPRT deficiency, as explained above.
Neuropathological Findings
Overall, the most frequent abnormality among imaging and autopsy studies that has been reported is a relatively small reduction in brain volume, that may escape notice in routine studies. Quantitative MRI studies showed a 17% reduction of total cerebral volume and 12–34% reduction in volumes of the basal ganglia [24]. In another cross-sectional voxel-based morphometric MRI study, LND patients showed a 17% volume reduction in gray matter compared to controls, particularly in the basal ganglia, frontotemporal, and limbic regions [25]. Moreover, the white matter volume was reduced in LND by 26%, particularly frontal white matter adjoining limbic and temporal regions and the motor cortex [26].
Autopsy studies have not revealed any signs suggestive of a degenerative process or other consistent abnormalities in any brain region. However, neurons of the substantia nigra from the LND cases showed reduced melanization and reduced immunoreactivity for tyrosine hydroxylase, the rate-limiting enzyme in dopamine synthesis [27]. These findings suggest an important relationship between purine recycling pathways and the neurochemical integrity of the dopamine neurons.
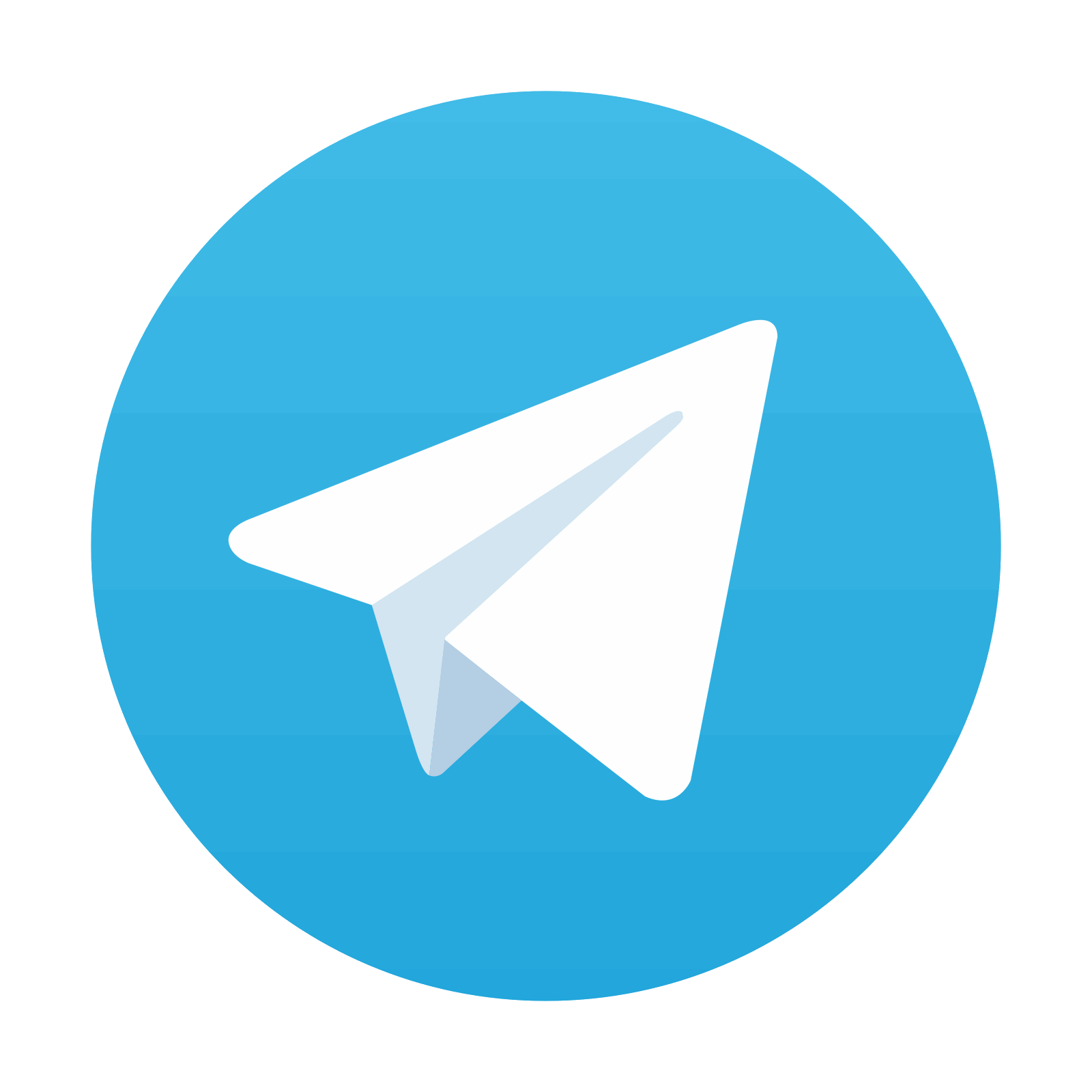
Stay updated, free articles. Join our Telegram channel

Full access? Get Clinical Tree
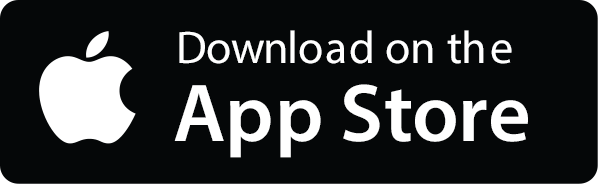
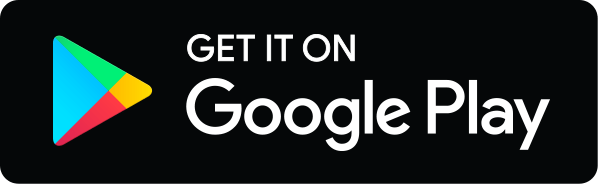
