Diseases of the Peripheral Nerves: Introduction
In this single chapter, an attempt is made to provide an overview of the very large and difficult subject of peripheral nerve disease. Because the structure and function of the peripheral nervous system are relatively simple, one might suppose that our knowledge of its diseases would be fairly complete. Such is not the case. For example, when a group of patients with chronic polyneuropathy were investigated intensively in a highly specialized center for the study of peripheral nerve diseases several decades ago, a suitable explanation for their condition could not be found in 24 percent (Dyck et al, 1981) and even more discouraging figures prevail in our clinics today. Moreover, the physiologic basis of many neuropathic symptoms continues to be elusive and in several of the neuropathies the pathologic changes have not been fully determined.
There has, however, been a surge of interest in diseases of the peripheral nervous system (PNS), which promises to change this state of affairs. Rapidly advancing techniques in the fields of immunology and molecular genetics are now clarifying entire categories of neuropathic disease. Also, in recent years, effective forms of treatment for several peripheral neuropathies have been introduced, making accurate diagnosis imperative. For these reasons, clinicians now find the peripheral neuropathies among the most challenging and gratifying categories of neurologic disease.
General Considerations
It is important to have a clear concept of the extent of the PNS and the mechanisms by which it is affected by disease. The PNS includes all neural structures lying outside the pial membrane of the spinal cord and brainstem with the exception of the optic nerves and olfactory bulbs, which are special extensions of the brain. The nerves within the spinal canal and attached to the ventral and dorsal surfaces of the cord are the spinal roots; those attached to the ventrolateral surface of the brainstem are the cranial nerve roots, or cranial nerves.
The dorsal, or posterior (afferent, or sensory), spinal roots consist of central axonal processes of the sensory and cranial ganglia. On reaching the spinal cord and brainstem, the roots extend for variable distances into the dorsal horns and posterior columns of the cord and into the spinal trigeminal and other tracts in the medulla and pons before synapsing with secondary sensory neurons, as described in Chaps. 8 and 9 that are devoted to the neurology of pain and sensation. The peripheral axons of the dorsal root ganglion cells are the sensory nerve fibers. They terminate as freely branching or specialized corpuscular endings—i.e., the sensory receptors—in the skin, joints, and other tissues. The sensory nerve fibers vary greatly in size and in the thickness of their myelin covering; based on these dimensions, they are classified as type A, B, or C, as discussed in Chap. 8.
The ventral, or anterior (efferent, or motor), roots are composed of the emerging axons of anterior and lateral horn cells and motor nuclei of the brainstem. Large, heavily myelinated fibers terminate on muscle fibers and smaller unmyelinated ones terminate in sympathetic or parasympathetic ganglia. From these autonomic ganglia issue the axons that terminate in smooth muscle, heart muscle and conducting system, and glands. Traversing the subarachnoid space, where they lack well-formed epineurial sheaths, the cranial and spinal roots (both sensory and motor) are bathed in and are susceptible to substances in the cerebrospinal fluid (CSF), the lumbosacral roots having the longest exposure (Fig. 46-1).
Figure 46-1.
Diagram showing the relationships of the peripheral nerve sheaths to the meningeal coverings of the spinal cord. The epineurium (EP) is in direct continuity with the dura mater (DM). The endoneurium (EN) remains unchanged from the peripheral nerve and spinal root to the junction with the spinal cord. At the subarachnoid angle (SA), the greater portion of the perineurium (P) passes outward between the dura mater and the arachnoid (A), but a few layers appear to continue over the nerve root as part of the root sheath (RS). At the subarachnoid angle, the arachnoid is reflected over the roots and becomes continuous with the outer layers of the root sheath. At the junction with the spinal cord, the outer layers of the root sheath become continuous with the pia mater (PM). (From Haller FR, Low FM: The fine structure of the peripheral nerve root sheath in the subarachnoid space in the rat and other laboratory animals. Am J Anat 131:1, 1971, by permission.)
The vast extent of the peripheral ramifications of cranial and spinal nerves is noteworthy, as are their thick protective and supporting sheaths of perineurium and epineurium that are endowed with a vascular supply through longitudinal arrays of richly anastomosing nutrient arterial branches. The perineurium comprises the connective tissue sheaths that surround and separate each bundle of nerve fibers (fascicles) of varying size, each fascicle containing several hundred axons. The sheath that binds and surrounds all the fascicles of the nerve is the epineurium. As the nerve root approaches the cord, the epineurium blends with the dura (see Fig. 46-1). The fine connective tissue covering of individual nerve fibers is the endoneurium. Longitudinally oriented and widely anastomotic endoneural vessels also nourish the nerve fibers and are susceptible to disease.
The nerves traverse narrow foramina (intervertebral and cranial) and a few pass through tight channels peripherally in the limbs (e.g., the median nerve between the carpal ligament and tendon sheaths of flexor forearm muscles that make up the carpal tunnel; the ulnar nerve in the cubital tunnel). These anatomic features explain the sites of susceptibility of certain nerves to compression and entrapment and also to ischemic damage.
The axons themselves contain a complex internal microtubular apparatus for maintaining the integrity of their membranes and for transporting substances such as neurotransmitters over long distances between the nerve cell body and the distant reaches of the nerve fiber. As discussed in Chap. the long axons of sensory nerves can properly be considered to be dendrites but we use the term “axon” in this and other chapters to denote all the neuronal processes of peripheral enrves. Nerve fibers (axons) are coated with short segments of myelin of variable length (250 to 1,000 μm), each of which is enveloped by a Schwann cell and its membrane that constitute the myelin sheath. In fact, the PNS may be accurately defined as the part of the nervous system that is invested by the cytoplasm and membranes of Schwann cells. Each myelin segment and Schwann cell has a symbiotic relationship to the axon but is morphologically independent. The structure of the axonal membrane in the gaps between segments of the myelin sheaths (nodes of Ranvier) is specialized, containing a high concentration of sodium channels and permitting the saltatory electrical conduction of nerve impulses as described in Chap. 45. Unmyelinated fibers, more numerous in peripheral nerves than myelinated ones, also arise from cells in dorsal root and autonomic ganglia. Small bundles of these naked (unmyelinated) axons are enveloped by a single Schwann cell; delicate tongues of Schwann cell cytoplasm partition these bundles and separate individual axons. Each sensory nerve fiber terminates in a specialized ending which is designed to be especially sensitive to certain natural stimuli as discussed in Chaps. 8 and 9.
The features described previously enable one to conceptualize the possible avenues by which disease may affect the peripheral nerves. Pathologic processes may be directed at any one of the several groups of nerve cells whose axons constitute the nerves, i.e., the cells of the anterior or lateral horns of the spinal cord, the dorsal root ganglia, or the sympathetic and parasympathetic ganglia. Each of these cell types exhibits specific vulnerabilities to disease, and if destroyed—as, for example, the motor nerve cells in poliomyelitis—there is secondary degeneration of the axons and myelin sheaths of the peripheral fibers of these cells. Neuropathic symptoms are also induced by alterations of function and structure of the ventral and dorsal columns of the spinal cord, which contain the fibers of exit and entry of anterior horn and dorsal root ganglion cells, respectively. The myelin of these centrally located fibers is constituted differently from that of the peripheral nerves, being enveloped by oligodendrocytes rather than Schwann cells and the nerve fibers are supported by astrocytes rather than fibroblasts.
Because of the intimate relation of the nerve roots to the CSF and to specialized arachnoidal cells (the arachnoidal villi), a pathologic process in the CSF or leptomeninges may damage the exposed spinal roots. Diseases of the connective tissues affect the peripheral nerves that lie within their sheaths. Diffuse or localized arterial diseases may injure nerves by occluding their nutrient arteries. In a large category of immune-mediated neuropathies, the damage is the result of a cellular or humoral attack on various components of myelin. A subset of these is characterized by the binding of circulating antibodies to the specialized regions at the nodes of Ranvier, causing a block of electrical conduction. A complement-dependent humoral immune reaction against the radicular or peripheral axon is also known. Toxic or immunologic agents that selectively damage the Schwann cells or their membranes cause demyelination of peripheral nerves, leaving axons relatively intact, or a toxin may specifically affect axons and dendrites by poisoning their cell bodies, the axolemma, or the lengthy and complex axonal transport apparatus.
Finally, one might correctly suppose that axons of the motor or sensory nerves, sympathetic fibers of varying diameter and length, or the end organs to which they are attached would each have its own particular liability to disease. At present we can cite only a few examples of diseases that cause disease through these mechanisms exclusively: e.g., diphtheria, in which the bacterial toxin acts directly on the membranes of the Schwann cells near the dorsal root ganglia and adjacent parts of motor and sensory nerves (the most vascular parts of the peripheral nerve); polyarteritis nodosa, which causes occlusion of vasa nervorum, resulting in multifocal nerve infarction; tabes dorsalis, in which there is a treponemal meningoradiculitis of the posterior roots (mainly of the lumbosacral segments); doxorubicin toxicity, wherein protein synthesis of dorsal root ganglion cells is blocked with subsequent neuronal destruction; poisoning by arsenic, which combines with the axoplasm of the largest sensory and motor nerves via sulfhydryl bonds; and vincristine toxicity, which damages the microtubular transport system. Analogous anatomic pathways are probably implicated in other diseases by mechanisms that remain to be discovered.
Among the genetically determined neuropathies, the altered gene products are now known in some cases to lead to defective myelination, which greatly slows conduction along nerves. In other genetic diseases it is known that structural components of the axon are disrupted, leading to axonal degeneration and impaired electrical conduction.
Several distinct histopathologic changes are recognized in the peripheral nerve, although they are not disease-specific and they may be present in varying combinations in any given case. The three main ones are segmental demyelination, wallerian degeneration, and axonal degeneration (diagrammatically illustrated in Fig. 46-2).
Figure 46-2.
Diagram of the basic pathologic processes affecting peripheral nerves. In wallerian degeneration, there is degeneration of the axis cylinder and myelin distal to the site of axonal interruption (arrow) and central chromatolysis. In segmental demyelination, the axon is spared. In axonal degeneration, there is a distal degeneration of myelin and the axis cylinder as a result of neuronal disease. Both wallerian and axonal degeneration cause muscle atrophy. Further details are found in the text. (Courtesy of Dr. Arthur Asbury.)
The myelin sheath is the most susceptible element of the nerve fiber, for it may break down as part of a primary process involving the Schwann cells or of the myelin itself, or it may be damaged secondarily as a consequence of disease affecting its axon. Focal degeneration of the myelin sheath with sparing of the axon is called segmental demyelination. The characteristic change of segmental demyelination is the disappearance of the sheath over segments of variable length, bounded on each end by one side of a node of Ranvier and an adjacent preserved segment of myelin. This exposes long segments of the axon to the interstitial environment. Myelin may also degenerate from axonal disease in a general process that may occur either proximal or distal to the site of axonal interruption.
Common to many lesions of the peripheral nerve is wallerian degeneration, a reaction of both the axon and myelin distal to the site of disruption of an axon. Wallerian degeneration might be described as “dying forward,” a process in which the nerve degenerates from the point of axonal damage outward. In contrast, when the axon degenerates as part of a “dying-back” phenomenon in a more generalized metabolically determined polyneuropathy, it is termed axonal degeneration. Here, the axon is affected progressively from the distal-most site to the proximal, with dissolution of myelin that occurs roughly in parallel with the axonal change. One possible explanation for this process is that the primary damage is to the neuronal cell body, which fails in its function of synthesizing proteins and delivering them to the distal parts of the axon. Certain toxic and metabolic processes affect axons uniformly along their length or impair anterograde axonal transport to the periphery; the functional impairment is then proportional to the size and length of the blocked axons.
Destruction of a proximal spinal motor root results in a gradual dissolution of the distal motor nerve and its myelin sheath (a form of wallerian degeneration). The neuronal motor cell body that gives rise to the motor fiber undergoes characteristic retrograde morphologic changes described below but does not die. Similar destruction of the dorsal spinal root produces secondary wallerian degeneration of the posterior columns of the spinal cord, but not of the peripheral sensory nerve because the dorsal root ganglion cell maintains the integrity of the distal axon. In other words, destruction of axons results within several days in wallerian degeneration of the myelin distal to the point of injury but not transgressing the neuronal cell body. The myelin breaks down into blocks or ovoids in which lie fragments of axons (digestion chambers of Cajal). The myelin fragments are then converted, through the action of macrophages, into neutral fats and cholesterol esters and carried by these cells to the bloodstream.
Certain diseases affect the neuron primarily rather than the axon and cause either a motor or sensory neuronopathy. In the former case, the anterior horn cell is affected by a disease process (motor neuron disease, or motor neuronopathy) and in the latter, the sensory ganglion cell (ganglionopathy) is destroyed. A type of wallerian distal degeneration of the respective nerve fibers follows.
Some of these pathologic reactions are more easily understood if one considers certain features of cytoskeletal structure and function of nerve cells and their axons. The axon contains longitudinally oriented neurofilaments and microtubules, which are separated but interconnected by cross-bridges. Their main function involves the transport of substances from nerve cell body to axon terminal (anterograde transport) and from the distal axon back to the cell body (retrograde transport). Thus, when the axon is severed, organelles cannot be transmitted to the distal axon for the purpose of renewing membrane and neurotransmitter systems. By means of retrograde axonal transport, the cell bodies receive signals to increase their metabolic activity and to produce growth factors and other materials needed for axonal regeneration. In an incompletely defined way, the axon also creates a local environment that allows the Schwann cell to maintain the integrity of the adjacent myelin sheath. Loss of this trophic influence leads to dissolution of the myelin sheath, but not of the Schwann cell itself.
There are also highly characteristic histopathologic changes in the nerve cell body termed chromatolysis as a secondary consequence of axonal interruption. These retrograde changes consist of swelling of the cell cytoplasm and marginalization and dissolution of the Nissl substance. The important point again is that despite the destructive changes in the nerve fibers, the nerve cells, while altered in histologic appearance, are left intact with preservation of the apparatus required for recovery.
In segmental demyelination, recovery of function may be rapid because the intact but denuded axon needs only become remyelinated. The newly formed internodal segments are initially thinner than normal and of variable length. By contrast, recovery is much slower with wallerian or axonal degeneration, often requiring months to a year or more because the axon must first regenerate and then reinnervate the muscle, sensory organ, or blood vessel before function returns. When the regenerating axon first becomes myelinated, the internodal myelin segments are short, the length of one normal internode being replaced by three or four shorter new ones. Recurrent demyelination and remyelination lead to “onion bulb” formations and enlargement of nerves, the result of proliferating Schwann cells and fibroblasts that encircle the axon and its thin myelin sheath. If nerve cells are destroyed, no recovery of function is possible except by collateral regeneration of axons from intact nerve cells. Interruption of a nerve fiber by severing or by crude destruction usually prevents continuity from being reestablished. Regenerating axon filaments take aberrant courses and, with fibroblastic scar formation, they may form a disorganized clump of tissue termed pseudoneuroma.
These relatively few pathologic reactions do not, in themselves, differentiate the many dozens of diseases of the peripheral nerves, but when they are considered in relation to the selective effects on various types and sizes of fibers, the topography of the lesions, and the time course of the process, they furnish criteria for fairly accurate diagnosis. Moreover, the identification of these basic reactions is of great value in the inspection of pathologic material obtained from biopsy or autopsy.
There are additional special pathologic changes, not specifically neural in nature that characterize certain diseases of the peripheral nervous system. These involve inflammatory or vascular changes or deposition of material in the interstitium of the nerve. For example, acute demyelinative polyneuritis of the Guillain-Barré type is characterized by endoneurial infiltrations of lymphocytes and other mononuclear cells in the nerves, roots, and sensory and sympathetic ganglia. Deposition of amyloid in the endoneurial connective tissue and walls of vessels affecting the nerve fibers are the distinctive features of inherited and acquired amyloid polyneuropathy. Diphtheritic polyneuropathy is typified by the demyelinative character of the nerve fiber change, the location of this change in and around the roots and sensory ganglia, the subacute course, and the lack of inflammatory reaction. A number of neuropathies are characterized by the deposition of antibodies and complement on the myelin sheath or on elements of the axon. These changes can be demonstrated by immunohistopathologic techniques. Many other polyneuropathies (paraneoplastic, nutritional, porphyric, arsenical, and uremic) are topographically symmetrical and represent forms of axonal degeneration but cannot be easily distinguished from one another on histopathologic grounds.
Concerning the pathology of the mononeuropathies, our knowledge is somewhat more complete. Compression of nerve or nerve roots, local or segmental ischemia, stretch, and laceration of nerves are understandable mechanisms and their pathologic changes can be reproduced experimentally. Tumor infiltration and importantly, vasculitis with ischemic infarction of nerve account for a proportion of cases. Of infections and granulomas localized to single nerves, leprosy, sarcoid, and herpes zoster represent identifiable disease states. For most of the acute mononeuropathies that are a result of transient compression, the pathologic changes have yet to be fully defined, as they are usually reversible states that provide no opportunity for complete pathologic examination. Experimental models of nerve compression indicate disruption of tubular transport and local demyelination. The common symptoms of compression such as paresthesias are explained, as discussed further on, by exposure of sodium channels along denuded axons and spontaneous and ectopic electrical discharges.
Symptomatology of Peripheral Nerve Disease
There are a number of motor, sensory, reflex, autonomic, and trophic symptoms and signs that are typical of peripheral nerve disease. Grouping them into syndromes based on their temporal and topographic features has proved to be of great value in clinical diagnosis. Although motor, sensory, reflex, and trophic changes are taken together to determine specific diagnosis, each element of the neuropathic diseases is first given in detail further on.
It is not surprising that weakness in various patterns and degrees is a feature of almost all neuropathies. The degree of weakness is proportional to the number of axons or motor neurons affected. Polyneuropathies that are the result of axonal damage are characterized foremost by a relatively symmetric distribution of weakness that is, moreover, distal because the pathologic changes begin in the far distal parts of the largest and longest nerves and advance along the affected fibers toward their nerve cell bodies (dying-back neuropathy, or “distal axonopathy”). The muscles of the feet and legs are typically affected earlier and more severely than those of the hands and forearms. In milder forms of axonal disease, only the feet and lower legs are involved. Truncal and cranial muscles are usually the last to yield, and then only in severe cases. This represents the “length-dependent” pattern that is typical of axonal degeneration. The nutritional, metabolic, and toxic neuropathies assume this predominantly distal “axonal” pattern. An exception is porphyria, an axonal process in which there may be mainly proximal weakness. By contrast, in demyelinating polyneuropathies, the multifocal nature of lesions and blockage of electrical conduction often leads to weakness of proximal limb and facial muscles before or at the same time as distal parts are affected.
Another pattern of neuropathic weakness is one in which all the muscles of the limbs, trunk, and neck are involved almost simultaneously, often including respiratory paralysis, therefore making it impossible to determine if the axons or myelin, or both, have been damaged. The best characterized of these processes is the Guillain-Barré syndrome (GBS). Less common causes of generalized paralysis include diphtheria, tick paralysis, and certain toxic polyneuropathies. Fatalities, when they occur, are usually a result of respiratory failure.
A predominantly bibrachial paralysis is an unusual presentation of neuropathic disease but may occur in the inflammatory-demyelinating polyneuropathies, as well as in Sjögren syndrome, chronic immune or paraneoplastic neuropathies, lead neuropathy, Tangier disease, and in a familial type of brachial neuritis. (A more frequent cause of bibrachial palsy is disease of the motor neurons themselves namely, motor system disease, or a lesion placed centrally in the cervical cord that damages these same neurons.) Paraparesis is not typical of the generalized polyneuropathies, but it is observed with infections and inflammations of the cauda equina, as occurs with Lyme disease, cytomegalovirus, herpes simplex, and with neoplastic infiltration of the nerve roots. Bifacial and other cranial nerve paralyses are likely to occur in GBS, neoplastic invasion, with connective tissue diseases, HIV and herpes virus infection, sarcoidosis, Lyme disease, or one of the rare metabolic neuropathies (Refsum, Bassen-Kornzweig, Tangier, and Riley-Day). These are discussed in Chap. 47 on diseases of the cranial nerves and in respective chapters on infections and metabolic diseases of the nervous system.
Atrophy of weak or paralyzed muscles is characteristic of chronic disease of the motor neuron or motor axon and conversely, demyelinating neuropathies relatively spare muscle bulk because of the absence of denervation. Atrophy proceeds slowly over several weeks and months, the degree being proportional to the number of damaged motor nerve fibers. The maximum degree of denervation atrophy after an acute injury to the axons occurs in 90 to 120 days and reduces muscle volume by 75 to 80 percent. Atrophy may also be a consequence of disuse; it occurs over many weeks but in itself does not reduce muscle volume by more than 25 to 30 percent. In chronic axonal neuropathies, the degrees of paralysis and atrophy tend to correspond. As mentioned previously, atrophy does not coincide with weakness in acute paralysis caused by the demyelinative neuropathies in which the nerve fiber is relatively less affected than is the myelin. Ultimately in muscle atrophy, there is degeneration and loss of the denervated muscle fibers. This process begins in 6 to 12 months; in 3 to 4 years, most of the denervated fibers will have degenerated. If reinnervation takes place within a year or so, motor function and muscle volume may be restored.
As a rule, neuropathies are associated with a reduction or loss of tendon reflexes. Most often, this is the result of an interruption of the afferent (sensory) portion of the monosynaptic reflex arc. The reflexes may be diminished if muscular function is impaired, but this occurs mainly in the case of extreme atrophy, in which there are too few muscle fibers to manifest a contraction. There are, of course, many other processes that reduce the tendon reflexes, but it is the neuropathies with which loss of reflexes is most closely associated. An exception is the group of small-fiber neuropathies, in which tendon reflexes may be retained, even with marked loss of perception of painful stimuli. This discrepancy is attributable to the dependence of the afferent component of the tendon reflex arc on the large, heavily myelinated fibers that originate in muscle spindles. Conversely, in neuropathies that affect the largest diameter, heavily myelinated fibers, the tendon reflexes are diminished early and disproportionately to weakness. Slowing of conduction in sensory fibers may also abolish the reflex by dispersing the afferent volley of impulses initiated by the tendon tap. There is generally a concordance between areflexia and a loss of proprioceptive and joint-position senses; i.e., the large nerve fibers from spindle afferents are of the same type and size as those mediating these forms of sensation. Furthermore, loss of sensory functions that are dependent on these large fibers in the presence of preserved reflexes implicates the central projections of the sensory ganglion cells i.e., a lesion in the posterior columns of the spinal cord that does not interrupt the afferent tendon reflex arc. Regional loss of a reflex is usually a sign of a radiculopathy.
Most polyneuropathies cause impairment of both motor and sensory functions, but one is often affected more than the other. In the toxic and metabolic neuropathies, sensory loss usually exceeds weakness. These differences are emphasized in the descriptions of individual peripheral nerve diseases in later parts of the chapter.
In the axonal polyneuropathies, sensation is affected symmetrically in the distal segments of the limbs and more in the legs than in the arms, owing to the length-dependent nature of most diseases that affect peripheral nerves. In most types, all sensory modalities (touch-pressure, pain and temperature, vibratory and joint position senses) are impaired or eventually lost, although one modality is affected disproportionately to the others, or pain and temperature sensation (small afferent fibers) may be impaired more than joint position and vibration (larger fibers). As an axonal neuropathy worsens, there is spread of sensory loss from the distal to more proximal parts of the limbs and eventually, to the anterior abdomen, thorax, and the face. An “escutcheon” pattern of sensory loss over the abdomen and thorax in severe axonal neuropathy may be mistaken for the sensory level of a spinal cord lesion. Another characteristic form of sensory loss affects the trunk, scalp, and face; this is the pattern of a sensory ganglionopathy that is the result of simultaneous dysfunction of all parts of the sensory nerve.
Most often, universal sensory loss is attributable to an acquired disease affecting the sensory ganglia (sensory neuronopathy); a paraneoplastic process, certain toxic or immune diseases are usually responsible (e.g., Sjögren disease, scleroderma).
These symptoms were described in Chaps. 8 and 9. Sensory symptoms tend to be especially marked in the hands and feet. “Pins and needles,” “falling asleep,” “stabbing,” “tingling,” “prickling,” “electrical,” and “Novocain-like” are the adjectives chosen by patients to describe these positive sensory experiences. In some neuropathies, paresthesias and numbness are the only symptoms and objective sensory loss is lacking or minimal. Certain neuropathies characteristically cause pain, which is described as burning, aching, sharp and cutting, or crushing and at times may resemble the lightning pains of tabes dorsalis. Perversion of sensation (allodynia) is also commonplace in some polyneuropathies—e.g., tingling, burning, stabbing pain, or just an uncomfortable dysesthesia is induced by tactile stimuli. Under these conditions a stimulus induces not only an aberrant sensation but also one that radiates to adjacent areas and persists after the stimulus is withdrawn. As remarked in Chap. 9, the reactions of a patient with allodynia may seem to indicate hypersensitivity (“hyperesthesia”), but more often the sensory threshold is actually raised and it is the sensory experience or response that is exaggerated (hyperpathia).
Painful paresthesias and dysesthesias are particularly common in diabetic, alcoholic–nutritional, and amyloid neuropathies. Mainly they affect the feet (“burning feet”) and less often the hands. In herpes zoster, they are confined to dermatomal regions of the body. A particularly intense form of burning pain typifies the causalgia of a partial nerve lesion (usually traumatic) of the ulnar, median, posterior tibial, peroneal, or occasionally some other nerve (see Chap. 8 and further on in this chapter).
The mechanism of thermal and painful dysesthesias is not fully understood. It has been theorized that a loss of large touch-pressure fibers disinhibits the pain-receiving nerve cells in the posterior horns of the spinal cord. An argument against this explanation is the lack of pain in Friedreich ataxia, in which the larger neurons degenerate, and also in certain purely sensory polyneuropathies, where only the perception of tactile stimuli (large fibers) is lost. A more likely explanation, supported by microneurographic recordings, is that dysesthetic pain results from ectopic discharges arising at many sites along surviving intact or regenerating nerve fibers or their terminal receptors. It has been postulated, on uncertain grounds, that the deep, aching neuropathic pain of sciatica or brachial neuritis (nerve trunk pain) arises from irritation of the normal endings (nervi nervorum) in the sheaths of the nerve trunks themselves (Asbury and Fields). These considerations are discussed in Chap. 8.
Proprioceptive deafferentation with retention of a reasonable degree of motor function may give rise to ataxia of gait and of limb movement as discussed in Chap. 9. Dysfunction of the spinocerebellar fibers of the peripheral nerves is probably the source of the ataxia. Some of the most severe ataxias of this type occur with sensory ganglionopathy, as commented further on.
Ataxia without weakness is also characteristic of tabes dorsalis, a purely posterior root disease, but this syndrome is duplicated by diabetic polyneuropathy, which may affect posterior roots (diabetic pseudotabes) and by a variant of GBS (termed Fisher syndrome). The ataxia is indistinguishable from that caused by cerebellar diseases, but other features of cerebellar dysfunction such as dysarthria and nystagmus are lacking. Characteristic of the sensory-ataxic gait are brusque, flinging, slapping movements of the legs. Loss of proprioception may also give rise to small wavering, fluctuating movements of the outstretched fingers—called pseudoathetotic, or “dancing fingers.”
An action tremor of fast-frequency type may also appear during certain phases of a polyneuropathy; Shahani and coworkers had the impression that it is a result of loss of input from the muscle-spindle afferents. Corticosteroid therapy enhances this fast tremor. A particularly severe form of slower action tremor is combined with clumsiness of movement in the neuropathies caused by the autoimmune, anti-myelin-associated glycoprotein (anti-MAG) polyneuropathy and in some cases of chronic inflammatory demyelinating polyneuropathy (CIDP). The tremor may be so coarse as to resemble the intention tremor of cerebellar disease and all movements are rendered useless. However, a tremor at rest is not found in these afferent-sensory neuropathies. The neuropathic type of tremor is also discussed in Chap. 6.
In a few of the chronic polyneuropathies, the feet, hands, and even the spine may become progressively deformed. This is most likely to occur when the disease begins during childhood. Austin pointed out that foot deformity is found in 30 percent of patients with hereditary polyneuropathy, and spine curvature is found in 20 percent. In early life, the feet are pulled into a position of talipes equinus (plantar deviation) because of disproportionate weakness of the pretibial and peroneal muscles and the unopposed action of the calf muscles. Weakness of the intrinsic foot muscles during the period of life when the bones are forming allows the long extensors of the toes to dorsiflex the proximal phalanges and the long flexors to shorten the foot, heighten the arch, and flex the distal phalanges. The result is the claw foot—le pied en griffe—or pes cavus (high arches) when the process is less severe. These changes in the structure of the foot are valuable diagnostic indicators that a neuromuscular disease originated in early childhood or during intrauterine development. A congenital claw hand has a similar implication. Unequal weakening of the paravertebral muscles on the two sides of the spine during early development leads to kyphoscoliosis.
Denervation atrophy of muscle can be considered the main trophic disturbance resulting from interruption of the motor nerves. However, there are numerous other changes. Analgesia of distal limb parts makes them susceptible to burns, pressure sores, and other forms of injury that are easily infected and heal poorly. In an anesthetic and immobile limb, the skin becomes tight and shiny, the nails curved and ridged, and the subcutaneous tissue thickened (“trophic changes”). Hair growth is diminished in denervated areas. If the autonomic fibers are also interrupted, the limb becomes warm and pink. Repeated injuries and chronic subcutaneous and osteomyelitic infections result in a painless loss of digits and the formation of plantar ulcers (mal perforant du pied). These are prominent features of the recessive form of hereditary sensory neuropathy and we have observed them in dominant forms as well. In tabes dorsalis and syringomyelia as well as certain familial and other chronic polyneuropathies analgesic joints, when chronically traumatized, may first become deformed and then actually disintegrate in a process called Charcot arthropathy (“Charcot joint”).
Apart from analgesia, a critical factor in these trophic changes may be aberrant neural regulation of the distal vasculature, which interferes with normal tissue responses to trauma and infection. Ali and colleagues have related the ulcer formation to loss of C fibers, which mediate both pain and autonomic reflexes. However, paralyzed limbs, even in hysteria, if left dependent, are often cold, swollen, and pale or blue. These are probably secondary effects of immobilization, as pointed out long ago by Lewis and Pickering. Erythema and edema, burning pain, and cold sensations surely can be evoked by peripheral nerve irritation, particularly of C and A-δ fibers as discussed in Chap. 8.
Anhidrosis and orthostatic hypotension, two of the most frequent manifestations of autonomic failure, predominate in certain types of polyneuropathies. They occur frequently in amyloidosis and in other small-fiber polyneuropathies, especially diabetic, and in several congenital types. These are also the main features of an acute autonomic polyneuropathy called pandysautonomia (Young et al; Adams et al; Low et al) and can be prominent in some cases of GBS. The neuropathic dysautonomic conditions are described in detail in Chap. 26 and later in this chapter.
Other manifestations of autonomic paralysis are small or medium-sized unreactive pupils that are unusually sensitive to certain drugs (see Chap. 14); lack of sweat, tears, and saliva; erectile dysfunction; weak bowel and bladder sphincters with urinary retention or overflow incontinence; and weakness and dilation of the esophagus and colon. As a result of vagal and other parasympathetic dysfunction, the normal variability of heart rate with respiration (sinus arrhythmia) is lost and there may be paralytic ileus or dyscoordinated peristalsis, as well as achlorhydria and hyponatremia. Some of these abnormalities are found in diabetic and amyloid polyneuropathy. They correspond to degeneration of small unmyelinated autonomic fibers in the peripheral nerves.
In any neuropathy involving sensory nerves, there is loss of autonomic function in the same zones as sensory loss. This is not true of radicular diseases because the autonomic fibers join the spinal nerves from the sympathetic chain and parasympathetic ganglia more distally. Changes in sweating and cutaneous blood flow may be demonstrated by a number of special tests described in Chap. 26.
Fasciculations and cramps are not prominent features in most polyneuropathies and in this respect there is a difference from diseases of the anterior horn cells where they are important features. There are exceptions, however. Chronic spinal motor root compression leads to fasciculations or painful spasms in the innervated muscles. Occasionally one observes a state of mild motor polyneuropathy that, upon recovery, leaves the muscles in a state variably referred to as myokymia, continuous muscular activity, and neuromyotonia as discussed in Chaps. 45 and 50. The affected muscles ripple and quiver and occasionally cramp. Use of the muscles increases this activity and there is a reduction in their contractile efficiency, which the patient senses as a stiffness and heaviness. In some instances this apparently constitutes the entire neuropathic syndrome and may be relieved by carbamazepine or phenytoin.
Other closely related phenomena are spasms or involuntary movements of the toes and feet. The latter, when the sole manifestation of disease, was referred to by Spillane and colleagues as the syndrome of painful legs and moving toes. It has been attributed by Nathan to ectopic discharges in sensory roots, ganglia, or nerves, evoking both pain and organized movements. This is but one of many causes of the nocturnal restless leg syndrome, but it does not explain the more common type of idiopathic restless leg nocturnal syndrome described in Chap. 19. Other possible mechanisms for cramps and spasms are ephaptic cross-transmission between adjacent axons denuded of myelin, segmental hyperactivity from deafferentation, and neuronal sprouting during reinnervation. Infrequently, the muscle activity induces odd postures or slow writhing movements that Jankovic and van der Linden have likened to dystonia. The pathophysiology of these asynchronous activities of motor neurons is not known. Stimulation of a motor nerve in these cases, instead of causing a brief burst of action potentials in the muscle, results in a prolonged or dispersed series of potentials lasting several hundred milliseconds. Evidently, branched axons involved in collateral innervation have unstable polarization that may last for years.
Approach to the Patient with Peripheral Neuropathy
The clinician is faced initially with several problems that can be solved sequentially when dealing with this group of diseases: (1) establishing the existence of disease of the peripheral nervous system and differentiating it from a process of the central nervous system, neuromuscular junction or the muscles; (2) distinguishing by clinical examination which of the main topographic syndromes it being displayed; (3) determining by examination and nerve conduction studies) if the problem is predominantly of a motor or sensory or autonomic in nature or is of mixed type and whether the myelin sheath, the axon, or cell body (motor or sensory neurons) is the target of disease; and (4) assessing if the neuropathy is acquired or hereditary in nature. When taken together, these features limit the likely etiologic diagnoses from a vast list of possibilities.
ACTION TESTED | ROOTSa | NERVES | MUSCLES |
---|---|---|---|
Cranial | |||
Closure of eyes, pursing of lips, exposure of teeth | Cranial 7 | Facial | Orbicularis oculi Orbicularis oris |
Elevation of eyelids, movement of eyes | Cranial 3, 4, 6 | Oculomotor, trochlear, abducens | Levator palpebrae, extraocular |
Closing and opening of jaw | Cranial 5 | Motor trigeminal | Masseters |
Pterygoids | |||
Protrusion of tongue | Cranial 12 | Hypoglossal | Lingual |
Phonation and swallowing | Cranial 9, 10 | Glossopharyngeal, vagus | Palatal, laryngeal, and pharyngeal |
Elevation of shoulders, anteroflexion and turning of head | Cranial 11 and upper cervical | Spinal accessory | Trapezius, sternomastoid |
Brachial | |||
Adduction of extended arm | C5, C6 | Brachial plexus | Pectoralis major |
Fixation of scapula | C5, C6, C7 | Brachial plexus | Serratus anterior |
Initiation of abduction of arm | C5, C6 | Brachial plexus | Supraspinatus |
External rotation of flexed arm | C5, C6 | Brachial plexus | Infraspinatus |
Abduction and elevation of arm up to 90° | C5, C6 | Axillary nerve | Deltoid |
Flexion of supinated forearm | C5, C6 | Musculocutaneous | Biceps, brachialis |
Extension of forearm | C6, C7, C8 | Radial | Triceps |
Extension (radial) of wrist | C6 | Radial | Extensor carpi radialis longus |
Flexion of semipronated arm | C5, C6 | Radial | Brachioradialis |
Adduction of flexed arm | C6, C7, C8 | Brachial plexus | Latissimus dorsi |
Supination of forearm | C6, C7 | Posterior interosseous | Supinator |
Extension of proximal phalanges | C7, C8 | Posterior interosseous | Extensor digitorum |
Extension of wrist (ulnar side) | C7, C8 | Posterior interosseous | Extensor carpi ulnaris |
Extension of proximal phalanx of index finger | C7, C8 | Posterior interosseous | Extensor indicis |
Abduction of thumb | C7, C8 | Posterior interosseous | Abductor pollicis longus and brevis |
Extension of thumb | C7, C8 | Posterior interosseous | Extensor pollicis longus and brevis |
Pronation of forearm | C6, C7 | Median nerve | Pronator teres |
Radial flexion of wrist | C6, C7 | Median nerve | Flexor carpi radialis |
Flexion of middle phalanges | C7, C8, T1 | Median nerve | Flexor digitorum superficialis |
Flexion of proximal phalanx of thumb | C8, T1 | Median nerve | Flexor pollicis brevis |
Opposition of thumb against fifth finger | C8, T1 | Median nerve | Opponens pollicis |
Extension of middle phalanges of index and middle fingers | C8, T1 | Median nerve | First, second lumbricals |
Flexion of terminal phalanx of thumb | C8, T1 | Anterior interosseous nerve | Flexor pollicis longus |
Flexion of terminal phalanx of second and third fingers | C8, T1 | Anterior interosseous nerve | Flexor digitorum profundus |
Flexion of distal phalanges of ring and little fingers | C7, C8 | Ulnar | Flexor digitorum profundus |
Adduction and opposition of fifth finger | C8, T1 | Ulnar | Hypothenar |
Extension of middle phalanges of ring and little fingers | C8, T1 | Ulnar | Third, fourth lumbricals |
Adduction of thumb against second finger | C8, T1 | Ulnar | Adductor pollicis |
Flexion of proximal phalanx of thumb | C8, Tl | Ulnar | Flexor pollicis brevis |
Abduction and adduction of fingers | C8, T1 | Ulnar | Interossei |
Crural | |||
Hip flexion from semiflexed position | L1, L2, L3 | Femoral | Iliopsoas |
Hip flexion from externally rotated position | L2, L3 | Femoral | Sartorius |
Extension of knee | L2, L3, L4 | Femoral | Quadriceps femoris |
Adduction of thigh | L2, L3, L4 | Obturator | Adductor longus, magnus, brevis |
Abduction and internal rotation of thigh | L4, L5, S1 | Superior gluteal | Gluteus medius |
Extension of thigh | L5, S1, S2 | Inferior gluteal | Gluteus maximus |
Flexion of knee | L5, S1, S2 | Sciatic | Biceps femoris |
Semitendinosus | |||
Semimembranosus | |||
Dorsiflexion of foot (medial) | L4, L5 | Peroneal (deep) | Anterior tibial |
Dorsiflexion of toes (proximal and distal phalanges) | L5, S1 | Peroneal (deep) | Extensor digitorum longus and brevis |
Dorsiflexion of great toe | L5, S1 | Peroneal (deep) | Extensor hallucis longus |
Eversion of foot | L5, S1 | Peroneal (superficial) | Peroneus longus and brevis |
Plantar flexion of foot | S1, S2 | Tibial | Gastrocnemius, soleus |
Inversion of foot | L4, L5 | Tibial | Tibialis posterior |
Flexion of toes (distal phalanges) | L5, S1, S2 | Tibial | Flexor digitorum longus |
Flexion of toes (middle phalanges) | S1, S2 | Tibial | Flexor digitorum brevis |
Flexion of great toe (proximal phalanx) | S1, S2 | Tibial | Flexor hallucis brevis |
Flexion of great toe (distal phalanx) | L5, S1, S2 | Tibial | Flexor hallucis longus |
Contraction of anal sphincter | S2, S3, S4 | Pudendal | Perineal muscles |
At the outset it must be determined whether the neurologic findings correspond to one of the following syndromic patterns:
Polyneuropathy
Radiculopathy or polyradiculopathy
Neuronopathy—motor or sensory
Mononeuropathy
Multiple mononeuropathies (mononeuropathy multiplex)
Plexopathy (involvement of multiple nerves in a plexus)
A discussion of these patterns is given in Chap. 9, but the main facts are repeated here.
In polyneuropathy, a generalized process affecting the peripheral nerves, weakness is relatively symmetrical from the beginning and progresses bilaterally; reflexes are lost in affected parts but particularly at the ankles; sensory complaints and loss of sensation are most pronounced distally, and in the feet before the hands in most cases.
Polyradiculopathy, a disease of multiple spinal roots, differs from polyneuropathy in that the neurologic signs are asymmetrical, with an erratic distribution that may, for example, be proximal in one limb and distal in another. Weakness and zones of sensory loss correspond to involvement of one or more spinal or cranial roots. Pain in the sensory distribution of the roots is a common feature. The common single radiculopathy, most often the result of root compression by disease of the spinal column, is identified by pain, sensory, motor, and reflex change solely in the distribution of one nerve root. The distinction from mononeuropathy (see later) is not always apparent and one must resort to a reference or to memorized knowledge of the motor and sensory innervation patterns of roots and nerves as given in Figs. 9-1, 9-2, and 9-3 and on the overleafs. Most helpful is the limitation of sensory loss to one of the dermatomes, but it so happens that there is overlap between adjacent dermatomes and such a pattern is not easily discerned.
Mononeuropathy is the most circumscribed form of peripheral nerve disease. It is reflected by weakness and sensory loss in the territory of a single peripheral nerve. Specific features serve to differentiate mononeuropathy from a radiculopathy—for example, weakness in dorsiflexion and eversion of the foot is referable either to the peroneal nerve or to the L5 nerve root; however, if there is weakness of inversion of the foot, innervated by the tibial nerve, the fault must be with the L5 root, not with the peroneal nerve. Conversely, if inversion is spared in a foot drop, the lesion ins in the peroneal nerve. The distribution of sensory loss also aids in distinguishing the two processes; for example, in the aforementioned case the region of sensory change corresponding to the L5 root extends almost up to the knee on the anterior surface of the foreleg whereas it ends a limited distance above the ankle in the case of a peroneal nerve lesion (see the sensory maps in Figs. 9-1, 9-2, and 9-3).
At times, particularly in advanced stages, the accumulation of multiple mononeuropathies, termed mononeuropathy multiplex, may be difficult to differentiate from polyneuropathy as discussed further on.
Plexopathies (brachial or lumbosacral) create the most confusing patterns of motor and sensory involvement; only one limb is affected, but the motor, sensory, and reflex loss does not conform to a pattern of several adjacent nerve roots or nerves. Knowledge of the innervation of the involved muscles at the level of the plexus usually clarifies the situation.
In sensory neuronopathy, the ganglion cells rather than the peripheral sensory nerves are predominantly affected. This gives rise to symptoms and signs of sensory loss in both a proximal and distal distribution, including the scalp, thorax, abdomen, and buttocks as well as the extremities; sensory ataxia is a common accompaniment. There is no weakness, but movements may be awkward as a result of a sensory ataxia. Motor neuronopathy is essentially the obverse condition, a disorder of the anterior horn causing weakness, fasciculations, and atrophy in a widespread distribution and, therefore, not properly included as a process of the peripheral nerves.
The apparent complexity of peripheral nerve disease is greatly simplified by recognizing that, of the multitude of diseases, each manifests itself by one or another of above-described topographic and sensory-motor patterns for which reason the pattern of neuropathy sets limits on the etiologic possibilities.
In the analysis of a polyneuropathy, it is of further value to determine whether the process is predominantly motor with less sensory involvement or the converse, or purely sensory, motor, or mainly autonomic. The time course of the disease also informs diagnosis. An acute onset (i.e., rapid evolution) is nearly always an inflammatory, immunologic, toxic, or vascular polyneuropathy. The other extreme, a polyneuropathy evolving over many years, is indicative of a hereditary or, rarely, a metabolic disease. Most of the toxic, nutritional, and systemic diseases of nerve develop subacutely over several weeks and months. In addition to the patient’s report of the progress of symptoms, signs such as muscle atrophy signify a process of relatively long-standing, at least several months in duration.
The etiologic diagnosis of polyneuropathy is next guided by deducing whether the myelin sheath or the axon is primarily involved (i.e., demyelinating or axonal neuropathy). The neurologic examination alone may be sufficient to make this distinction, but greater precision is attained from nerve conduction studies and needle examination of muscles (EMG). The latter test also helps separate primary disorders of muscle (myopathies) and neurogenic denervation of muscle or neuromuscular block (myasthenia). The electrical examinations of nerve and muscle described in Chap. 45 greatly reduce the number of possible diagnoses. These EMG and nerve conduction abnormalities may be so characteristic as to virtually define a neuropathy, e.g., chronic demyelinative motor neuropathy with multifocal conduction block.
Other useful laboratory procedures are (1) biochemical tests to identify metabolic, nutritional, or toxic states; (2) CSF examination (increase in protein and in cells that indicate radicular or meningeal involvement); (3) nerve, and occasionally accompanying muscle biopsy (the latter aids in the diagnosis of vasculitic causes of neuropathy); (4) measurement of immunoglobulins and antineural antibodies that relate to immune-mediated neuropathies; and (5) genetic testing for several of the inherited neuropathies. These are discussed in the context of each of the main diseases of nerve and in the later parts of Chap. 45.
Once having established that the patient has a disease of the peripheral nerves and having ascertained its clinical and electrophysiologic pattern and time course, one is usually able to determine its cause. This is accomplished most readily by allocating the case in question to one of the categories listed in Table 46-2, which classifies the peripheral nerve diseases syndromically according to their mode of evolution and clinical presentation. Our use of the terms acute, subacute, and chronic neuropathy must be explained. By acute, we mean evolution in terms of days, and by subacute, evolution in terms of weeks. Chronic is divided into two groups: one in which the neuropathy has progressed for a period of several months to a few years and another in which progression is over many years, most of which prove to have a genetic cause. It can be restated that these temporal properties are, with the topographic pattern, the main determinants in the categorization of neuropathy.
|
Diseases of the peripheral nerves are considered in a more comprehensive fashion in the two-volume Peripheral Neuropathy, edited by Dyck and colleagues and in the text by Amato and Russell cited in the references. Also recommended are more concise monographs by Schaumburg and associates and by Asbury and Thomas, and the atlas on the pathology of peripheral nerve by King.
Syndrome of Acute Motor Paralysis with Variable Disturbance of Sensory and Autonomic Function
A number of differences separate the polyneuropathies in this category: (1) acute inflammatory demyelinating or axonal polyneuropathy (GBS), (2) vasculitic polyneuropathies, (3) porphyria, (4) certain toxic polyneuropathies, and (5) acute sensory and autonomic polyneuropathies. Of these various acute polyneuropathic diseases, the Guillain-Barré demyelinative syndrome, because of its frequency and gravity, is most demanding of the physician’s attention.
Guillain-Barré Syndrome (Landry-Guillain-Barré-Strohl Syndrome, Acute Inflammatory Demyelinating Polyneuropathy, AIDP)
This is the most common cause of acute or subacute generalized paralysis in practice. (During certain past epochs it was exceeded in frequency by polio.) GBS occurs in all parts of the world and in all seasons, affecting children and adults of all ages and both sexes. A mild respiratory or gastrointestinal infection or immunization precedes the neuropathic symptoms by 1 to 3 weeks in approximately 60 percent of cases. Typical is a nondescript upper respiratory infection, but almost every known febrile infection and immunization has at one time or another been reported to precede GBS (some probably coincidentally). In recent years, it has been appreciated from serologic studies that the enteric organism Campylobacter jejuni is the most frequent identifiable antecedent infection, but it accounts for only a relatively limited proportion of cases. Other common antecedent events or associated illnesses include viral exanthems in children and numerous other viral illnesses in adults and children, particularly the large viruses of the herpes family (cytomegalovirus [CMV], Epstein-Barr virus [EBV], HIV), and less often, bacterial infections other than Campylobacter (Mycoplasma pneumoniae, Lyme disease). There are less certain associations with lymphoma (particularly Hodgkin disease) and with the systemic autoimmune diseases.
The earliest description of an afebrile generalized paralysis is probably that of Wardrop and Ollivier, in 1834. Important landmarks were Landry’s report (1859) of an acute, ascending, predominantly motor paralysis with respiratory failure leading to death among peasants on his land; Osler’s (1892) description of “febrile polyneuritis”; and the account by Guillain, Barré, and Strohl (1916) of a benign polyneuritis with albuminocytologic dissociation in the CSF (increase in protein without cells). The first comprehensive account of the pathology of GBS was that of Haymaker and Kernohan (1949), who stressed that edema of the nerve roots was an important change in the early stages of the disease. Subsequently, Asbury and colleagues (1969) established that the essential lesion, from the beginning of the disease, was perivascular mononuclear inflammatory infiltration of the roots and nerves. More recently, it has been found that complement deposition on the myelin surface may be the earliest immunologic event. For details of the historical and other aspects of this disease, see the monographs by Ropper and colleagues (1991) and by Hughes (1990).
The incidence rate of GBS has varied between 0.4 and 1.7 cases per 100,000 persons per year; the median taken from several studies is 1.1 and may be most dependable. It is generally a nonseasonal and nonepidemic disease, but outbreaks have been recorded in rural China following exposure of children to C. jejuni through chicken feces deposited in rice paddies. Women appear to be slightly more susceptible. The age range in our series has been 8 months to 81 years, with attack rates highest in persons 50 to 74 years of age. Cases are known in infants and in the very aged.
In addition to a seasonal increase in incidence after natural influenza outbreaks, the administration of the A/New Jersey (swine) influenza vaccine, given in the United States in late 1976, brought attention to a slight increase in the incidence of GBS and several, but not most subsequent influenza vaccination programs have been associated with a marginal increase in cases. Representative was the widely publicized worldwide H1N1 vaccination program that was studied in Quebec, where the calculated risk of developing GBS after vaccination was in the range 2 cases per 1 million doses of vaccine, barely above the baseline rate and appearing mostly in individuals over 50 years (De Wals et al). GBS appears in temporal relationship to almost all other vaccinations, but the association in these instances may be idiosyncratic and infrequent. Trauma and surgical operations may precede the neuropathy, but a causal association to them also remains uncertain.
The typical case is readily identified. Paresthesias and slight numbness in the toes and fingers are the earliest symptoms; only infrequently are they absent throughout the illness. The major clinical manifestation is weakness that evolves more or less symmetrically over a period of several days to a week or two, or somewhat longer. The proximal as well as distal muscles of the limbs are involved, usually the lower extremities before the upper (thus the older term Landry ascending paralysis); the trunk, intercostal, neck, and cranial muscles may be affected later. Weakness progresses in approximately 5 percent of patients to total motor paralysis with respiratory failure within a few days. In severe cases, the ocular motor nerves are paralyzed and even the pupils may be unreactive.
More than half of the patients complain of pain and an aching discomfort in the muscles, mainly those of the hips, thighs, and back. These symptoms precede weakness and may be mistaken for lumbar disc disease, back strain, and orthopedic diseases. A few patients describe burning in the fingers and toes, and if this appears as an early symptom, it may become a persistent problem. Sensory loss is variable during the first days and may initially be barely detectable so that the typical case has the character of a predominantly motor neuropathy. By the end of a week, vibration and joint position sense in the toes and fingers are usually reduced; when such loss is present, deep sensibility (touch-pressure-vibration) tends to be more affected than superficial (pain-temperature).
Reduced and then absent tendon reflexes are consistent findings. Only the ankle reflexes may be lost during the first week of illness. At an early stage, the arm muscles are usually stronger than the leg muscles, and in a few cases, they are spared almost entirely. Facial diplegia occurs in more than half, sometimes bilaterally at the same time or sequentially over days. Other cranial nerve palsies, if they occur, usually come later, after the arms and face are affected; they are the initial signs in a variant pattern of disease as described further on. At the onset, there is no fever, and if lymphadenopathy or splenomegaly occurs, they are related to a preceding viral infection.
Disturbances of autonomic function include sinus tachycardia and, less often, bradycardia, facial flushing, fluctuating hypertension and hypotension, loss of sweating, or episodic profuse diaphoresis; one or more are common in minor form and infrequently do they become pronounced or persist for more than a week. Urinary retention occurs in approximately 15 percent of patients soon after the onset of weakness, but catheterization is seldom required for more than a few days. Numerous medical complications follow in severe cases as a result of immobilization and respiratory failure, as discussed further on under “Treatment.”
The archetypical illness described in the preceding paragraphs is typically a result of the widespread inflammatory-demyelinating process within peripheral nerves. This is contrasted with an axonal form of GBS described just below.
Attention was drawn by Feasby and colleagues (1986) to an acute areflexic polyneuropathy clinically similar to typical GBS but characterized pathologically by widespread and severe axonal degeneration. In their initial report they described 5 patients with a rapid evolution of polyneuropathy and slow and poor recovery. Unlike the common form of demyelinating GBS, muscle atrophy became apparent relatively early in the axonal form (within weeks). The defining feature was the presence of numerous electrically inexcitable motor nerves and signs of extensive denervation. This finding could also signify a distal demyelinating block from which complete recovery is possible (Triggs et al). Nevertheless, most cases of abrupt and severe denervating paralysis, particularly if postinfectious, are caused by the axonal form of GBS (Ropper, 1986b).
Postmortem examinations have disclosed severe axonal degeneration in nerves and roots with minimal inflammatory changes and little demyelination, even early in the disease. Based on the deposits of complement and the presence of macrophages in the periaxonal space, a humoral antibody directed against some component of the axolemma was postulated by Griffin and associates (1995). Visser and colleagues reported similar findings in a series of acute motor polyneuropathies. The outbreaks of motor neuropathy that occur seasonally in rural China have many of the same characteristics. These cases appear to be triggered largely by C. jejuni infections. Some, but not all, sporadic instances of acute axonal GBS have been preceded by the same infection. It is noteworthy that infection with the same bacteria can also induce a typical demyelinating form of GBS.
A proportion of axonal cases, perhaps up to one-fifth, are associated with circulating antibodies to the GM1 ganglioside of peripheral nerve, and some of these reflect recently preceding infection with C. jejuni. The acronyms AMAN (acute motor) and AMSAN (acute motor-sensory axonal neuropathy) are equivalents to axonal GBS. Another variant of this illness, of which we have seen several instances, has been an acute multifocal neuropathy with electrophysiologic motor conduction block that leaves the reflexes unaltered and has high titers of anti-GM1 antibody (Capasso et al). Most experience with the generalized axonal form of GBS indicates that recovery is prolonged and incomplete.
Regional |
Fisher syndrome of ophthalmoplegia, ataxia, and areflexia |
Cervico-brachial-pharyngeal weakness, often with ptosis |
Oculopharyngeal weakness |
Predominant paraparesis |
Bilateral facial or abducens weakness with distal paresthesias |
Ophthalmoplegia with GQ1b autoantibodies |
System specific |
Generalized ataxia without dysarthria or nystagmus |
Pure sensory |
Pure motor |
Pandysautonomia |
Axonal (AMAN) |
Portions of the clinical picture of GBS appear in isolated or abortive form and are a source of diagnostic confusion. Whereas in most patients the paralysis ascends from legs to trunk, to arms, and then to cranial regions, and reaches a peak of severity within 10 to 14 days, the pharyngeal-cervical-brachial muscles may be affected first or constitute the entire illness, causing difficulty in swallowing with neck and proximal arm weakness (Ropper, 1986a). Ptosis, often with ophthalmoplegia, may be added. The differential diagnosis then includes myasthenia gravis, diphtheria, and botulism and a lesion affecting the central portion of the cervical spinal cord and lower brainstem.
A syndrome comprising virtual or complete ophthalmoplegia with ataxia and areflexia represents a variant of GBS described by Fisher (and is called Fisher syndrome). A purely ophthalmoplegic form also exists; it may be coupled with the pharyngeal-cervical-brachial pattern mentioned earlier. Ophthalmoplegia, whether occurring alone or with weakness or ataxia of other parts, is almost uniformly associated with a specific antineural antibody, anti-GQ1b. The ophthalmoplegic pattern raises the diagnostic possibilities of myasthenia gravis, botulism, diphtheria, tick paralysis, and basilar artery occlusion. Bilateral but asymmetrical facial and abducens weakness coupled with distal paresthesias or with proximal leg weakness is other variants in our experience (Ropper, 1994). The tendon reflexes may be absent only at the ankles or at the knees. Lyme disease and sarcoidosis are then considerations in diagnosis. Whether bifacial palsy alone represents a variant of GBS is uncertain, but almost every case in our experience has had an alternative explanation.
Paraparetic, ataxic, and purely motor or purely sensory forms of the illness have also been observed. Less difficulty attends the correct diagnosis of GBS if paresthesias in the acral extremities, progressive reduction or loss of reflexes, and relative symmetry of weakness appear after several days. The laboratory tests, particularly nerve conduction studies that affirm the diagnosis of typical GBS, give similar but generally milder abnormalities if they are carefully sought in all these variant forms. In a few patients, the weakness continues to evolve for 3 to 4 weeks or longer. From this group, a chronic form of demyelinative neuropathy (CIDP) may emerge and an intermediate group that progresses for 4 to 8 weeks and then improves can be identified (see further on).
The most important laboratory aids are the electrodiagnostic studies and CSF examination. The CSF is under normal pressure and is acellular or contains only a few lymphocytes in all but 10 percent of patients; in the latter group, 10 to 50 cells (rarely more) per cubic millimeter, predominantly lymphocytes, may be found. The number of cells then decreases in a matter of 2 to 3 days; persistent pleocytosis suggests an alternative or additional process producing aseptic meningitis such as neoplastic infiltration, HIV, sarcoidosis, or Lyme infection. We have been unable to relate pleocytosis in the spinal fluid with any of the clinical features of GBS or to the severity of illness. The protein content is usually normal during the first few days of illness, but then it rises, reaching a peak in 4 to 6 weeks and persisting at a variably elevated level for many weeks. The increase in CSF protein is probably a reflection of widespread inflammatory disease of the nerve roots, but high values have had no clinical or prognostic significance in our material, apart from a few exceptional cases of pseudotumor cerebri (Ropper and Marmarou). In a few patients (fewer than 10 percent), the CSF protein values remain normal throughout the illness. From our experience, there is a higher proportion of patients with normal or only slightly elevated protein values among those with Fisher syndrome and other restricted or axonal forms of GBS.
Abnormalities of nerve conduction are early and dependable diagnostic indicators of GBS. In cases with a typical clinical and EMG/NCS presentation, one can probably dispense with the CSF analysis as a confirmatory test. The most frequent early electrodiagnostic findings are a reduction in the amplitude of muscle action potentials, slowed conduction velocity, and conduction block in motor nerves, singly or in combination (see Chap. 45). Prolonged distal latencies and reduced distal amplitudes (reflecting distal conduction block) and prolonged or absent F responses (indicating involvement of proximal parts of motor nerves and roots) are other important diagnostic findings, all reflecting focal areas of demyelination. The H reflex is almost always much delayed, or more often absent, but this does little more than confirm the loss of ankle reflexes. Although a limited electrodiagnostic examination may be normal early in the illness, a thorough study, which includes measurement of late responses, invariably shows disordered conduction in an affected limb within days of the first symptom. Features that indicate widespread axonal damage portend a poor and protracted recovery in both young and old patients as discussed above.
The clinical, CSF, and electrodiagnostic criteria for GBS were assessed by Asbury and Cornblath and are discussed in detail in the monograph by Ropper and colleagues. Many patients with acute GBS have shown gadolinium enhancement of the cauda equina roots on magnetic resonance imaging (21 of 24 patients in our study) and this may serve as a useful test in complicated cases (Gorson et al, 1996).
Beyond the close association between autoantibodies to GQ 1b and Fisher syndrome or other variants that include ophthalmoplegia as mentioned previously other anti-ganglioside antibodies have become of interest in GBS. The acute motor axonal variety has a tendency to be associated with antibodies to GM1 or GD1a and the pharyngeal-cervical-brachial syndrome, to GT1a. Much of this work comes from the laboratory of Yuki, and his review article with Hartung is recommended for further explanations of potential autoimmune mechanisms.
Abnormalities of liver function occur in fewer than 10 percent of patients, probably reflecting a recent or ongoing viral hepatitis, usually as a result of CMV or EBV infections (rarely one of the hepatitis viruses). T-wave and other electrocardiographic changes of minor degree are reported frequently but tend to be evanescent. The sedimentation rate is normal unless there is an additional process of infectious, neoplastic, or autoimmune nature, any of which can occasionally coexist with GBS. Hyponatremia occurs in a proportion of cases after the first week, but particularly in ventilated patients. This is usually attributable to the syndrome of inappropriate antidiuretic hormone secretion (SIADH), but a natriuretic type also occurs, from an excess of atrial natriuretic factor (Wijdicks et al). Transient diabetes insipidus is a rare and unexplained complication. With regard to proteinuria due to glomerulonephritis reported by several groups in cases of GBS, we have found it infrequently.
These have had a relatively consistent pattern and form. Even when the disease is fatal within a few days, most cases show endoneural perivascular (mainly perivenous) lymphocytic infiltrates. Later, there is segmental demyelination and a variable degree of wallerian degeneration. The cellular infiltrates are scattered throughout the cranial nerves, ventral and dorsal spinal roots, dorsal root ganglia, and along the entire length of the peripheral nerves. Swelling of nerve roots at the site of their dural exit has been emphasized by some authors and theorized to cause root damage.
Variations of this pattern have been observed, each perhaps representing a different immunopathology. For example, there may be widespread demyelinative changes and only a paucity of perivascular lymphocytes (Ropper and Adelman). In patients whose electrophysiologic tests display severe axonal damage early in the illness as discussed earlier the pathologic findings corroborate the predominantly axonal nature of the disease with secondary myelin damage and usually little inflammatory response. An occasional case has shown an inflammatory process with primary axonal damage rather than demyelination (Honovar et al).
Most evidence supports a cell-mediated immunologic reaction directed at peripheral nerves. Waksman and Adams demonstrated that experimentally induced peripheral nerve disease (experimental allergic neuritis [EAN]), clinically and pathologically indistinguishable from GBS, develops in animals 2 weeks after immunization with peripheral nerve homogenates. Brostoff and colleagues suggested that the antigen in this reaction is a basic protein, designated P2, found only in peripheral nerve myelin. Subsequent investigations by these authors indicated that the neuritogenic factor might be a specific peptide in the P2 protein. However, it has become evident that there is no dominant antigen–antibody reaction in GBS and it is likely that any number of myelin and axonal elements may be involved in inciting the immune reaction. Figure 46-3 diagrammatically illustrates the pathologic steps in this proposed reaction. As noted further on, complement also seems to be a necessary factor in the initial attack on myelin.
Figure 46-3.
Diagram of probable cellular events in acute inflammatory polyneuropathy (Guillain-Barré syndrome). A. Lymphocytes attach to the walls of endoneurial vessels and migrate through the vessel wall, enlarging and transforming as they do so. At this stage no nerve damage has occurred. B. More lymphocytes have migrated into the surrounding tissue. The first effect on the nerve is breakdown of myelin, the axon being spared (segmental demyelination). This change appears to be mediated by the mononuclear exudate, but the mechanism is uncertain. C. The lesion is more intense, polymorphonuclear leukocytes being present as well as lymphocytes. There is interruption of the axon in addition to myelin sheath damage; as a result, the muscle undergoes denervation atrophy and the nerve cell body shows central chromatolysis. If the axonal damage is distal, the nerve cell body will survive, and regeneration and clinical recovery are likely. If, as in D, axonal interruption has occurred proximally because of a particularly intense root or proximal nerve lesion, the nerve cell body may die and undergo dissolution. In this situation, there is no regeneration, only the possibility of collateral reinnervation of muscle from surviving motor fibers. (From Asbury et al [1969], by permission.)
Although the transmission of EAN by T cells sensitized to myelin is strong evidence of their role in GBS, antimyelin antibodies are probably involved in the initial part in the disease. The serum from patients with GBS damages myelin in tissue cultures and induces a characteristic (“vesicular”) form of myelin destruction. Subepineural injection of serum from GBS patients into the sciatic nerve of rats leads to local demyelination and electrical conduction block. The studies by Koski and associates of complement-dependent myelin damage by immunoglobulin (Ig) M antimyelin antibodies in GBS provided evidence that antimyelin antibodies are able to initiate myelin destruction even through T cells and that macrophages are the ultimate effectors of the damage. Indeed, the very earliest change that could be detected by Hafer-Macko and colleagues was the deposition of complement on the inner layer of myelin.
As mentioned earlier, circulating autoantibodies directed at components of nerve ganglioside are detected but only inconsistently in patients with GBS, the most important being anti-GQ1b, which is found in almost all patients with ophthalmoplegia. Approximately one-fifth of patients have anti-GM1 antibodies early in their course, corresponding in most instances to a predominantly motor presentation and to axonal damage, the highest titers being associated with cases that follow Campylobacter infections. Antibodies directed against GD1a or GT1b are associated in some cases with the pharyngeal-brachial-cervical variant. Thus it would seem that casting GBS exclusively as a humoral or as a cellular immune process is an oversimplification.
An unanswered question is what incites the immune reaction isolated to peripheral nerves in humans. All attempts to identify a virus or microbial agent within nerves have failed and it is likely that a variety of agents—viral, bacterial (particularly C. jejuni), certain vaccines, and perhaps neural injury itself—are each capable, in susceptible individuals, of precipitating an immune response against components of autologous peripheral nerve. The occurrence of GBS in patients with AIDS or with EBV or CMV infections simply indicates that these agents too induce such an autoimmune response without implicating a direct viral infection of nerve. The observation that only one of many individuals who are infected with a particular pathogen go on to develop GBS suggests that host factors are significant (there is, however, little consistency of human leukocyte antigen [HLA] types in GBS patients). Whether the aforementioned antibodies against various gangliosides of peripheral nerve are pathogenically active is also uncertain.
Several animal diseases—namely coonhound paralysis of dogs, Marek disease of chickens (a viral neuritis), and cauda equina neuritis of horses—resemble GBS superficially but do not share its main clinical or pathologic features.
GBS is not only the most frequent acute generalized polyneuropathy seen in general hospitals but also the most rapidly evolving and potentially fatal form. Any polyneuropathy that brings the patient to the brink of death or to respiratory failure within a few days will usually be of this variety. Most cases, however, are of the more limited variety with paresthesias, limb weakness, and areflexia. Several other conditions must be considered. The immediate problem is to differentiate GBS from acute spinal cord disease marked by sensorimotor paralysis with a defined spinal level and prominent sphincter disturbances. There may be diagnostic difficulty in the case of an acute lesion of the cord in which tendon reflexes are initially lost (spinal shock), or with necrotizing myelopathy, where a permanent loss of tendon reflexes follows extensive destruction of spinal gray matter. Early and transient urinary retention occurs in a proportion of patients with GBS and causes diagnostic confusion with spinal disease. Several features are useful in distinguishing GBS from a cervical myelopathy: in GBS, the facial and respiratory muscles are usually involved if there is generalized paralysis; the fingertips should be paresthetic once sensory symptoms have ascended to the level of the midcalves; marked sensory loss proximal to the hands or feet or only of the trunk is unusual early in the illness; and tendon reflexes are almost invariably lost in limbs that are too weak to resist gravity. Of course, careful testing of sensation on the trunk and limbs will expose the cause of paralysis as spinal in origin.
Tick paralysis, a disease of children in the United States but affecting both children and adults in Australia and elsewhere, may be nearly impossible to distinguish from GBS unless one finds the tick (see Chap. 43). In addition to an ascending generalized paralysis, both may cause ataxia and may paralyze eye movements, but sensory loss is not usually a feature of tick paralysis and the CSF protein is normal. Episodes of painful paralytic porphyria also bear a superficial resemblance to GBS. Predominant motor features in comparison to sensory ones is the major characteristic of GBS, for which reason the differential diagnosis also includes poliomyelitis, caused by the West Nile virus and by enteroviruses other than the polio agent. In these infectious cases, the illness is marked by fever, meningoencephalitic symptoms, early pleocytosis in the spinal fluid, and purely motor and usually asymmetrical areflexic paralysis, all unusual in GBS.
Several times we were misled by cases of carcinomatous meningitis with polyradiculopathy that caused a painless, subacute, and fairly symmetric but mainly distal weakness, similar to GBS. An irregular distribution of weakness between proximal and distal parts, the absence of facial weakness, and the appearance of symptoms sequentially in one limb after another suggest the presence of this type of neoplastic polyradiculopathy. Sciatica may occur as an early feature with either process but radicular pain in the arms is unusual in GBS. Examination of the spinal fluid usually settles the matter.
Another problem arises in distinguishing generalized GBS with ophthalmoparesis or the Fisher variant from basilar artery thrombosis. The presence of reactive pupils, areflexia, and F-wave abnormalities in GBS, and of lively reflexes and Babinski signs in the case of brainstem infarction, dependably separate the disorders. Ptosis and oculomotor weakness in GBS causes confusion with myasthenia gravis, but there are no sensory symptoms and the tendon reflexes are unimpaired in the latter disease. The mandibular muscles remain relatively strong in GBS, whereas the exercised jaw hangs open in myasthenia. Botulism also simulates this cranial variant of GBS, but pupillary reflexes are lost early in botulism (pupillary paralysis occurs mainly in advanced cases of GBS) and there is usually a bradycardia, which is unusual for GBS. Ingestion of shellfish or reef fish contaminated with saxitoxin, ciguatoxin, or tetrodotoxin (ciguatera, neurotoxic shellfish poisoning) is another cause of facial-brachial paresthesias, weakness, tachypnea, and iridoplegia lasting up to a few days—symptoms that resemble the cranial nerve variants of GBS.
A number of neuromuscular disorders in critically ill patients with systemic medical conditions are difficult to distinguish from GBS. These include the polyneuropathy of critical illness (see further on in the chapter); an accelerated neuropathy of renal failure that is seen mainly in diabetic patients receiving peritoneal dialysis (both discussed further on); acute hypophosphatemia induced by hyperalimentation; polymyopathy produced by the administration of high-dose corticosteroids; and the prolonged effects of neuromuscular blocking drugs, resulting in the accumulation of their metabolites in patients under conditions of renal failure and acidosis.
In severe cases, respiratory assistance and assiduous nursing are paramount, because the disease remits naturally and the outlook for recovery is favorable in the majority of patients. About one-quarter of our patients have required mechanical ventilation. Because a patient’s condition may deteriorate unpredictably and rapidly in the first days of illness, virtually all but the mildest cases should be admitted to the hospital for observation of respiratory, autonomic, and motor function. The comments that follow are applicable to most other forms of acute and subacute neuromuscular respiratory failure, including myasthenia gravis and high spinal cord injury.
Measurement of maximal inspiratory force and expiratory vital capacity suffices for the bedside estimation of diaphragmatic strength and respiratory function. The trend of these measurements is a guide to the likelihood of respiratory failure. As had been observed in poliomyelitis, the strength of the neck muscles and trapezii, which share the same segmental innervation as the diaphragm, tends to parallel diaphragmatic power. A rough estimate of breathing capacity may be obtained by having the patient count quickly on one deep breath. The ability to reach 20 generally corresponds to a vital capacity of greater than 1.5 L. If a downward trend in these measurements is recognized and the vital capacity diminishes to below about 10 mL/kg, endotracheal intubation and mechanical ventilation are usually necessary (see further on). However, a fairly severe impairment of ventilation may occur before the first sign of dyspnea appears and before there is elevation of arterial carbon dioxide content. Incipient respiratory failure may be evident by tachypnea and a decrease in arterial oxygen tension (PO2 less than 85 mm Hg) reflecting pulmonary atelectasis. When respiratory failure arises gradually as the patient weakens over days, there is slight tachycardia, diaphoresis, restlessness, and tachypnea. Attempts to forestall intubation and positive-pressure ventilation by using negative-pressure cuirass-type devices have been unsatisfactory in our experience. Patients with oropharyngeal weakness require intubation even earlier so as to prevent aspiration, but full mechanical ventilation is not always necessary at the same time. Patients in these circumstances should obviously be admitted to an intensive care unit staffed by personnel skilled in maintaining ventilation and airway patency.
The other major aspects of the treatment in severely affected patients involve the management of autonomic instability and the prevention of the many general medical problems that attend any immobilizing critical illness. Hypotension from dysautonomia, which occurs in approximately 10 percent of paralyzed patients and a smaller proportion with lesser degrees of weakness, is treated by intravenous infusions of saline and by the use of vasopressor agents for brief periods. Extremes of hypertension are managed by short-acting and titratable antihypertensive medications, such as intravenous labetalol. The choice and dosing of an antihypertensive drug is important, as episodes of hypertension may be rapidly succeeded by precipitous declines in pressure. Severe autonomic problems are difficult to anticipate, but provocative maneuvers such as ocular pressure to elicit heart block are used in some units to identify patients at risk.
In patients who are bedbound, prevention of electrolyte imbalances, gastrointestinal hemorrhage, and particularly pulmonary embolism (by the use of subcutaneous heparin or pneumatic compression boots) requires careful attention. Adynamic ileus is a problem in some cases, manifest by abdominal pain coincident with nasogastric tube feeding and by bloating; it may lead to bowel perforation even if feeding is discontinued. As mentioned, a number of patients become hyponatremic, usually from SIADH but occasionally from a natriuresis, and the drop in sodium is exaggerated by positive-pressure mechanical ventilation. The distinction between the two conditions that cause hyponatremia determines the course of treatment: fluid restriction in the case of SIADH or salt replacement in the case of sodium loss. Many patients have bizarre waking dreams or hallucinations after weeks of immobilization (oneiric hallucinations). A dependable mode of communication should be established by the nursing staff, preferably before the patient is intubated. A Plexiglas or opaque board with letters and phrases is useful for this purpose.
Failure to effectively clear the tracheobronchial airways and the need for prolonged mechanical ventilation are the usual indications for tracheostomy. In most cases, this procedure can be postponed until the third week of intubation. However, patients who become rapidly quadriplegic and ventilator-dependent benefit from tracheostomy earlier. Once tracheostomy is performed, careful tracheal toilet and treatment of pulmonary and urinary tract infections by the use of appropriate antibiotics are required; prophylactic antibiotic treatment is not recommended. With tracheostomy and intensive care, the mortality from the disease can be reduced to approximately 3 percent (Ropper and Kehne; see further on under “Prognosis”).
The decisions to wean and then discontinue respiratory aid and to remove the endotracheal or tracheostomy tube are based on the degree and timing of recovery of respiratory function. The weaning process generally begins when the vital capacity reaches approximately 10 mL/kg and comfortable breathing can be sustained for a few minutes. The relative merits of the numerous methods of delivering positive-pressure volume-cycled ventilation and its gradual withdrawal are not covered here, but there is little to favor one over the other and the reader is referred to the monograph Neurological and Neurosurgical Intensive Care by Ropper and colleagues.
Physical therapy (passive movement and positioning of limbs to prevent pressure palsies and, later, mild resistance exercises) can begin once they can be comfortably undertaken.
Specific treatment of the presumed immune disorder that underlies GBS includes plasma exchange and IVIg. Our practice has been to observe patients who are still able to walk unaided rather than institute treatment immediately. If the patient becomes unable to walk, shows a reduction in vital capacity, or signs of oropharyngeal weakness, plasma exchange or IVIg is instituted promptly. This typically occurs at the fifth to tenth day after the appearance of the first symptoms, but may be as early as 1 day or as late as 3 weeks.
Three large randomized trials comprising more than 500 patients have established the efficacy of plasma exchange administered during the evolving phase of GBS. In patients who are treated within 2 weeks of onset, there is an approximate halving of the period of hospitalization, of the duration of mechanical ventilation, and of the time required to achieve independent ambulation. However, in the largest trial, if the first plasma exchange was delayed for 2 weeks or longer after the onset of the disease, the procedure was of little value. Nonetheless, if a patient continues to progress in the third or fourth week of illness, it is probably still appropriate to institute the exchanges. The most important predictors of responsiveness to plasma exchange treatment are the same as for the overall prognosis, namely the patient’s age (responders are younger) and the preservation of motor compound muscle action potential amplitudes prior to instituting treatment (McKhann et al). One study has found that the condition of patients was better at 6 and 12 months after treatment as compared to untreated patients; other studies have been equivocal on this point and demonstrated mainly accelerated improvement.
The advised regimen of plasma exchange removes a total of 200 to 250 mL/kg of plasma in 4 to 6 treatments on alternate days, or over a shorter period if there is no coagulopathy. The replacement fluid is saline combined with 5 percent albumin. The need for large-bore venous access usually requires the insertion of a double-lumen subclavian or internal jugular catheters and this may be the main source of complications (pneumothorax, infection, hemorrhage). In some patients, treatment can be instituted, and sometimes the entire course completed, through the antecubital veins. During and after the procedure, hypotension, hypoprothrombinemia with bleeding and cardiac arrhythmias may occur. Some units measure the level of fibrinogen, which is greatly reduced by exchanges, before the next exchange to gauge to the risk of potential hemorrhage. Reactions to the citrate that is used to prevent blood from clotting in the plasma exchange machine are common but can be obviated by the cautious addition of calcium to the intravenous return line. Hepatitis and AIDS are not risks if plasma is replaced with albumin and saline rather than with pooled plasma.
As effective as plasma exchange is, IVIg (0.4 g/kg per day for 5 consecutive days) is both easier to administer and probably safer because there is no need for large intravenous access. The results of the first trial conducted by van der Meché and colleagues were corroborated in an international study led by Hughes, in which we participated (see Plasma Exchange/Sandoglobulin Guillain-Barré Syndrome Trial Group). That trial compared plasma exchange to IVIg and also evaluated their use sequentially. There was a tenuous trend toward a better outcome in patients who received plasma exchange and results were perhaps slightly better in a group who were treated with plasma exchange followed immediately by 5 days of immune globulin infusions; in both instances, however, the differences failed to attain statistical significance and the three modes of treatment were said to be equivalent. Renal failure, proteinuria, and aseptic meningitis, manifested most often by severe headache, are infrequent complications of IVIg. The only serious reactions we have encountered have been in a very few patients who congenitally lacked IgA and in whom pooled gamma globulin caused anaphylaxis, and a few cases of inflammatory local venous thrombosis in the region of the infusion site. The pharmacokinetics of IVIg are highly variable among individuals and some groups have found an association between a high rate of clearance of agent and poorer clinical outcome (see Kuitwaard and colleagues). This group has suggested that patients who show only a small increment in serum IgG levels might benefit from higher doses or a second course of IVIg.
After the use of either plasma exchange or IVIg, 5 to 10 percent of patients who initially improve will have a relapse that becomes apparent several days or up to 3 weeks after completion of treatment. If there had been a response to the initial therapy, the same treatment may be repeated or the alternative treatment may be tried; either can be successful. A few such patients relapse repeatedly and have the course of chronic inflammatory demyelinating polyneuropathy (see further on). In some patients under our care, this form of the disease stabilized after several months in response to the administration of corticosteroids, with very gradual tapering of the dose over several months, or in combination with repeated courses of IVIg or plasma exchanges.
The clinical improvement that follows the administration of IVIg or plasma exchange usually cannot be readily discerned in an individual patient; i.e., it is apparent only by comparing large groups of treated and untreated patients. For this reason it is not possible to judge that a patient who fails to improve or who worsens through the period of treatment has derived no benefit from therapy. The question nevertheless arises regarding further plasma exchanges or continued infusion of immune globulin in cases of continued worsening or lack of improvement. Further complicating the matter are the limited expectations for early improvement in cases of axonal GBS. Our advice has been to repeat either of the two immune treatments if a patient is clearly declining, particularly if there is evidence of demyelinating neuropathy on the NCS, and if the illness is not much longer than 4 weeks in duration. Performing plasma exchanges after the use of IVIg does not make sense to us (but this notion has not been tested); therefore, we either follow a series of exchanges with IVIg or, more often, repeat a course of IVIg as suggested by Farcas and colleagues.
The value of corticosteroids alone in the treatment of GBS has been disputed for decades. Many clinicians were persuaded of their benefit; however, two randomized controlled trials, one with conventional-dose prednisolone and the other with high-dose methylprednisolone, have failed to demonstrate beneficial effect (Hughes et al, 1991). Although corticosteroids can no longer be recommended as routine treatment for acute GBS, we have observed a few instances in which the administration of intravenous high-dose corticosteroids seemingly halted the progress of an acute case.
As already indicated, approximately 3 to 5 percent of patients do not survive the illness, even in the best equipped hospitals. In the early stages, death is most often a result of cardiac arrest, sometimes related to dysautonomia, adult respiratory distress syndrome, pneumo- or hemothorax, or some type of accidental machine failure. Later in the illness, pulmonary embolism and infectious complications of prolonged immobilization and respiratory failure are the main causes of death.
The majority of patients recover with mild motor deficits or sensory complaints in the feet or legs. In approximately 10 percent, however, the residual disability is pronounced; this occurs in those with the most severe and rapidly evolving form of the disease, when there has been evidence of widespread axonal damage and in those requiring early and prolonged mechanical ventilatory assistance. A fairly consistent predictor of residual weakness and muscle atrophy is the finding of greatly reduced amplitudes of muscle action potentials and widespread denervation, both indicative of axonal damage.
In patients with respiratory failure, the average period of machine-assisted respiration has been 22 days and the period of hospitalization approximately 50 days (these were twice as long prior to the introduction of plasma exchange and IVIg). As a rule, older adults recover more slowly than younger ones and children and have more residual weakness.
The most common remaining difficulties are weakness of the lower leg muscles, numbness of the feet and toes, and mild bifacial weakness. A few patients are left with sensory ataxia that tends to be severe and quite disabling. Distal neuropathic pain and persistent autonomic problems occur but are also infrequent. All manner of other late symptoms are attributed with little evidence to the illness and should be addressed on their own merits—fatigue and asthenia, muscle cramps, dizziness, pain, and breathlessness. Depression has not been frequent.
The speed of recovery varies, but its pace is steady. Often, it occurs within a few weeks or months; however, if axons have been damaged, their regeneration may require 6 to 18 months or longer. In our experience, little improvement can be expected in disabilities that have lasted 2 or more years.
Some 5 to 10 percent of patients encounter one or more recurrences of the acute polyneuropathy. An illness that in the beginning appeared to be an acute inflammatory polyradiculoneuropathy may fail to stabilize and continue to progress steadily, or there may be an incomplete remission followed by a chronic, fluctuating, slowly progressive neuropathy. These more chronic forms of inflammatory neuropathy are described in a later section of this chapter.
An acute or subacute symmetrical polyneuropathy is a frequent development in critically ill and septic patients, particularly in those with failure of multiple organs (Zochodne et al). This neuropathy causes difficulty in weaning a patient from the ventilator, even as the underlying critical illness comes under control. The neuropathic process, predominantly of motor type, varies in severity from an electrophysiologic abnormality without overt clinical signs, to quadriparesis with respiratory failure. Sensory symptoms and signs are variable but tend to be mild. Usually the cranial nerves are spared and there are few or no dysautonomic manifestations. In general, this type of polyneuropathy appears after several days or more of bacterial sepsis or other overwhelming infection (now called systemic inflammatory response syndrome [SIRS]) and multiple organ failure, and is preceded in most instances by a confusional state or a depressed state of consciousness (“septic encephalopathy”).
The EMG and NCS findings of a primary axonal process with early denervation and a normal CSF distinguish this entity from the typical demyelinative form of GBS. Autopsy material has usually disclosed little or no inflammatory changes in the peripheral nerves. Differentiating critical illness polyneuropathy from critical illness myopathy (see just below) and from the axonal form of GBS is difficult and depends on the context in which the illness occurs. All of these processes that occur in the intensive care unit, when extreme, can eliminate the motor nerve action potentials and when this configuration is found, the problem is most often attributed to the neuropathy, although this is not always correct. The toxic effects of drugs and antibiotics and nutritional deficiency must be considered in causation, but rarely can they be established. The many systemic mediators of sepsis are toxic to the peripheral nervous system; tumor necrosis factor has been proposed as one such endogenous toxin in causing neuropathy.
Critical illness polyneuropathy must also be distinguished from a poorly understood acute quadriplegic myopathy (critical illness myopathy) that also complicates critical illness (see Chap. 48). High doses of corticosteroids, particularly in combination with neuromuscular blocking agents, have been implicated. The acute myopathy, which affects both distal and proximal muscles, is sometimes heralded by an elevation in the serum creatine kinase (CK) concentration (at times up to several thousand units) and myopathic potentials in the EMG, and a unique degeneration of myofilaments in all the muscles is found. This illness is described in more detail in Chap. 48.
In addition to the well-known chronic sensory polyneuropathy associated with chronic renal failure that is discussed later in the chapter, there is a more rapid (“accelerated”) process that has not been widely appreciated as a cause of acute and subacute weakness. Most patients in our series were diabetics with stable end-stage renal failure who had been treated by peritoneal dialysis for their long-standing kidney disease (Ropper, 1993). In contrast to the better characterized and less severe chronic uremic neuropathy, generalized weakness and distal paresthesias progress over 1 or more weeks until a bedbound state is reached. The illness simulates subacute GBS. More aggressive dialysis or a change to hemodialysis has little immediate effect, although kidney transplantation is curative. Electrophysiologic studies show demyelinating features (slowing of conduction velocity), but usually not a conduction block. There is raised CSF protein concentration (not unexpectedly, for there is usually an element of diabetic neuropathy). A few reported cases have responded to plasma exchange or gamma globulin. As with the more common chronic uremic neuropathy, the cause of the acute form is unknown.
Attention was initially drawn to this entity by Sterman and colleagues in a report of 3 adult patients with rapidly evolving sensory ataxia, areflexia, numbness, and pain, beginning in the face and spreading to involve the entire body. In each instance, the symptoms began within 4 to 12 days following the institution of penicillin therapy for a febrile illness (antibiotics were subsequently shown not to be associated). Proprioception was profoundly reduced, but there was no weakness or muscle atrophy, despite generalized areflexia. The sensory deficit attained its maximum severity within a week, after which it stabilized and improved very little.
Electrophysiologic studies showed absent or slowed sensory conduction, but there were no abnormalities of motor nerve conduction or signs of denervation. In two patients, the CSF protein content was elevated to 126 and 175 mg/dL. Followup observations (for up to 5 years) disclosed no neoplastic or immunologic disorder, the usual identifiable causes of such a sensory neuronopathy. Lacking pathologic material, it was assumed from the permanence of the condition that sensory neurons were destroyed (sensory neuronopathy). A subsequent series of 42 patients reported by Windebank and colleagues emphasized an asymmetrical and brachial pattern of symptoms in some patients and initial involvement of the face in others. In contrast to Sterman’s cases, the CSF was usually normal and most patients had some improvement or a spontaneous resolution of symptoms. These authors viewed the process as a sensory neuropathy. In this and subsequent reports, as mentioned, antibiotics were not implicated.
This clinical pattern should be viewed as a syndrome rather than as a disease. There are two main presentations: with limb ataxia that does not have accompanying dysarthria or nystagmus, thus distinguishing it from a cerebellar disorder; and with generalized facial and truncal numbness that involves proximal and distal sensory areas and may include the top of the head, trunk, buttocks, scrotum, and oral mucosal membranes. The latter syndrome must be delineated from an evolving polyneuropathy, the early proximal symptoms being the most salient identifying feature for a ganglionopathy as mentioned in the introductory sections of this chapter. All the just described processes are accompanied by areflexia, but this may not be fully developed in the case of ganglionopathy for several days or longer.
Probably, most instances are immune and postinfectious in nature. The same pattern of sensory loss evolving in a subacute or chronic manner is well known to occur as a paraneoplastic illness, described further on in this chapter, or in association with the Sjögren syndrome, scleroderma, lupus erythematosus, paraproteinemia, HIV and human T-cell lymphotropic virus type I (HTLV-I) infection. Certain drugs and other agents, especially cisplatin and excessive intake of pyridoxine, are also causes of a sensory neuronopathy. These are discussed again later, under “Drug-Induced Neuropathies and Neuronopathies.” A rare form of GBS involves solely the large sensory fibers and produces ataxia, thereby simulating an acute sensory neuronopathy. In GBS, however, there is usually some degree of proximal weakness and the sensory changes do not extend to the face and trunk.
The neurotoxic effects of Corynebacterium diphtheriae and the mode of action of the exotoxin elaborated by the bacillus are described in Chap. 43. Local action of the exotoxin may paralyze pharyngeal and laryngeal muscles (dysphagia, nasal voice) within 1 or 2 weeks after the onset of the infection and shortly thereafter may cause blurring of vision because of paralysis of accommodation, but these and other cranial nerve symptoms may be overlooked. At this stage, the cranial neuropathy must be distinguished from that of GBS, botulism, and most of all, from myasthenia gravis.
A polyneuropathy, appearing 5 to 8 weeks later, takes the form of an acute or subacute limb weakness with paresthesias and distal loss of vibratory and position sense. The weakness characteristically involves all extremities at the same time or may descend from arms to legs. The patient may be unable to stand or walk and occasionally the paralysis is so extensive as to impair respiration. The CSF protein is usually elevated (50 to 200 mg/dL). Deaths that occur after the pharyngeal infection has subsided are a result of cardiomyopathy or, less often, of severe polyneuropathy with respiratory paralysis. This type of polyneuropathy, now quite rare, should be suspected in the midst of an outbreak of diphtheritic infection, as occurred in Russia (Logina and Donaghy).
The important pathologic change is one of segmental demyelination without inflammatory reaction of spinal roots, sensory ganglia, and adjacent spinal nerves. Anterior horn cells, axons, peripheral nerves distally, and muscle fibers remain normal (Fisher and Adams).
Diphtheria antitoxin, given within 48 h of the onset of the infection, reduces the incidence and severity of neuropathic complications. Antitoxin is probably of little value once the polyneuropathy begins. Thereafter, treatment is purely symptomatic, along the lines indicated for GBS. The prognosis for full recovery is excellent once respiratory paralysis is circumvented.
A severe, rapidly advancing, more or less symmetrical and mainly motor polyneuropathy—often with abdominal pain, psychosis (delirium or confusion), and convulsions—may be a manifestation of acute intermittent porphyria. This type of porphyria is inherited as an autosomal dominant trait and is not associated with cutaneous sensitivity to sunlight. The metabolic defect is in the liver and is marked by increased production and urinary excretion of porphobilinogen and of the porphyrin precursor Δ-aminolevulinic acid. The peripheral and central nervous systems may also be affected in another hepatic type of porphyria (the variegate type). In the latter, the skin is markedly sensitive to light and trauma, and porphyrins are at all times found in the stools. Both of these hepatic forms of porphyria must be distinguished from the rarer erythropoietic (congenital photosensitive) porphyria, in which the nervous system is not affected.
The classic study of acute intermittent porphyria was made by Waldenstrom in 1957. The initial and often the most prominent symptom is moderate to severe colicky abdominal pain. It may be generalized or localized and is unattended by rigidity of the abdominal wall or tenderness. Constipation and intestinal distention (ileus) are frequent. Attacks last for days to weeks and repeated vomiting may lead to inanition. In latent forms, the patient may be asymptomatic or complain only of slight dyspepsia.
The disease can be identified after some time by its characteristic recurrent attacks, often precipitated by drugs such as sulfonamides, griseofulvin, estrogens, barbiturates, phenytoin, and the succinimide anticonvulsants. The possibility of sensitivity to these drugs must always be kept in mind when convulsions are being treated in the porphyric patient. The first attack rarely occurs before puberty, and the disease is most likely to threaten life during adolescence and early adulthood. In contrast, acute polyneuropathy that appears for the first time in mid- or late adult life is not likely to be porphyric.
The neurologic manifestations are usually those of an acute polyneuropathy involving the motor nerves more severely than the sensory ones; less often, both sensory and motor nerves are affected more or less equally and sometimes autonomic nerves as well. The symptoms may begin in the feet and legs and ascend, or they may begin in the hands and arms (sometimes asymmetrically) and spread in a few days to the trunk and legs. Often, the weakness predominates in the proximal muscles of the limbs and limb girdle muscles, in which case there is loss of knee jerks with preservation of reflexes at the ankles. Sensory loss, often extending to the trunk, is present in half the cases. Facial paralysis, dysphagia, and ocular palsies are features of only the most severe cases. The CSF protein content is normal or slightly elevated.
The course of the polyneuropathy is variable. In mild cases the symptoms regress in a few weeks. Severe cases may progress to a fatal respiratory or cardiac paralysis in a few days, or the symptoms may advance in a saltatory fashion over several weeks, resulting in a severe sensorimotor paralysis that improves only after many months.
A disturbance of cerebral function (confusion, delirium, visual field defects, and convulsions) is likely to precede the severe, but not always the mild, forms of polyneuropathy, or there may be none of these central features. Cerebral manifestations subside in a few days or weeks, although one of our patients was left with a lasting homonymous hemianopia. Tachycardia and hypertension are frequent in the acute phase of the disease and fever and leukocytosis may occur in severe cases. In general, the prognosis for recovery is excellent, although relapse of the porphyria may result in cumulative damage to the peripheral nervous system (see discussion further on under “Diagnosis of Recurrent or Relapsing Polyneuropathy”).
In summary, the most characteristic features of porphyric neuropathy are the relapsing nature, acute onset, abdominal pain, psychotic symptoms, predominant motor neuropathy, often with an early bibrachial distribution of weakness, truncal sensory loss, and tachycardia. Rarely, the neuropathy develops without other symptoms.
The pathologic findings in the peripheral nervous system vary according to the stage of the illness at which death occurs. In the first few days, the myelinated fibers may appear entirely normal, despite almost complete paralysis. If symptoms had been present for weeks, degeneration of both axons and myelin sheaths are found in most of the peripheral nerves. The relation between the abnormality of porphyrin biosynthesis in the liver and nervous dysfunction has never been explained satisfactorily.
The diagnosis is confirmed by the demonstration of large amounts of porphobilinogen and Δ-aminolevulinic acid in the urine. The urine turns dark when standing as a consequence of the formation of porphobilin, an oxidation product of porphobilinogen.
The use of intravenous glucose and intravenous hematin (4 mg/kg daily for 3 to 14 days) is recommended as the most effective therapy. Other aspects of treatment include respiratory support, use of beta-blocking agents (labetalol) if tachycardia and hypertension are severe, continued intravenous glucose to suppress the heme biosynthetic pathway, and pyridoxine (100 mg bid) on the supposition that vitamin B6 depletion has occurred. Attempted prevention is of the utmost importance, since attacks can be precipitated by the aforementioned drugs as well as numerous others that are porphyrinogenic.
As indicated in Chap. 43, the peripheral nerves may be affected by a wide variety of toxins including metals, drugs, organophosphates, and industrial solvents. As a rule, the neuropathies induced by these agents fall into the subacute and chronic categories (to be discussed further on). However, certain drugs—notably triorthocresyl phosphate (TOCP) and other organophosphates (see Chap. 40), thallium and rarely, arsenic—produce a polyneuropathy that may be fatal in a matter of days. It should be stressed that organophosphate neuropathy can be identified in almost all instances by the severe anticholinergic effects that are apparent immediately after exposure. Severe and permanent motor paralysis is caused by TOCP; this ultimately proves to be a result of involvement of both upper and lower motor neurons.
Thallium salts, when taken in sufficient amount, produce a clinical picture resembling that of GBS or an acute sensory polyneuropathy. If the salts are taken orally, there is first abdominal pain, vomiting, and diarrhea, followed within a few days by pain and tingling in the toes and fingertips and then rapid weakening of muscles of the limbs, initially the distal ones. As the weakness progresses, the tendon reflexes diminish. Pain sensation is reduced more than tactile, vibratory, and position sense. Persistent acral pain with allodynia has been a major feature in 3 of the 5 patients we have examined; in 2 of our patients there was no weakness, only sensory loss and ataxia. All cranial nerves except the first and eighth may be affected; facial palsies, ophthalmoplegia, nystagmus, optic neuritis with visual impairment, and vocal cord palsies are additional abnormalities but only in the most severely affected patients. The CSF protein rises to more than 100 mg. Death may occur in the first 10 days as a result of cardiac arrest. The early onset of painful paresthesias, sensory loss, and pain localized to joints, back, and chest, as well as rapid loss of hair (after a week or two), all serve to differentiate this neuropathy from GBS, porphyria, and other acute polyneuropathies. Relative preservation of reflexes is noteworthy and rapidly evolving complete alopecia is a striking feature. Patients with lesser degrees of intoxication may recover completely within weeks or months. Thallium salts act like potassium and a high intake of potassium chloride hastens the excretion of thallium. Chelating agents are of unproven value but are usually included in treatment.
Some cases of arsenical and possibly mercurial polyneuropathy may also develop acutely. More often these conditions evolve subacutely, for which reason they are discussed further on. As alluded to earlier and in Chap. 43, certain other toxic neuropathies, such as those related to organophosphate or diethylene chloride (Sterno) poisoning, may have an acute onset and progress over days.
In regard to this category of polyneuropathy, many instances are imputed to toxins by both patients and unskeptical physicians with little substantiation. Before making such an attribution, it is useful to ask whether the clinical features are compatible with the known neurotoxicity of an environmental agent or drug; whether the severity of symptoms is consistent with degree of presumed exposure (real or imagined); whether the associated systemic signs of an intoxication are present; if other individuals similarly exposed are affected; and whether symptoms stabilize or improve once the patient is removed from the presumed source of exposure. Failure to satisfy these precepts generally signifies some other disorder.
On occasion, a vasculitic polyneuropathy as an isolated process or associated with lupus erythematosus, polyarteritis nodosa, and related disorders may develop as rapidly as GBS and careful clinical and electrophysiologic testing are needed to distinguish them. Three of our patients with polyarteritis and one with Churg-Strauss disease became completely paralyzed within a week and one died of intestinal perforation. However, most cases of neuropathy caused by vasculitis evolve more slowly, with the syndrome assuming an asymmetrical and multifocal distribution, for which reason it is described in the next section. There is no doubt that paraneoplastic neuropathies, discussed further in the subacute category, can evolve more rapidly than is typical for this process and thereby simulate GBS.
We have observed a few patients with alcoholism, occult carcinoma, Hodgkin disease, and renal transplantation develop an acute polyneuropathy, as rapid in its evolution as GBS, and acute episodes of this type have also been described in patients with Refsum disease.
Since the first description of such a case by Young and colleagues and Adams and associates, a number of others have been recorded and summarized by Low and colleagues. The condition, probably a type of postinfectious polyneuropathy in the category of GBS, is described in detail in Chap. 26. Some success has been achieved by treatment with IVIg. A subacute and more chronic form, also immune in nature, is described later under “Idiopathic Autonomic Neuropathy” and a paraneoplastic variety is known.
Syndrome of Subacute Sensorimotor Paralysis from Peripheral Neuropathy
Placed in this category are neuropathies that evolve over several weeks to months and, after reaching their peak of severity, tend to persist for a variable period. Admittedly, the dividing line between such cases and those that evolve over somewhat shorter or longer periods is indistinct; there are many diseases of nerve that overlap both the acute and the early chronic categories. In contrast to the acute polyneuropathies, however, most that are subacute have prominent sensory features and are of axonal type. The main exception is a subacute inflammatory–demyelinative type, essentially a slow form of GBS, evolving over 4 to 8 weeks, as described by Hughes and coworkers. Similarly, some instances of diphtheritic neuropathy evolve subacutely. Despite these qualifications, in the end, a symmetrical polyneuropathy syndrome of subacute type most often proves to be caused by nutritional deficiency, (often complicated by alcoholism) by a remote effect of cancer (paraneoplastic, as described later), by poisoning with arsenic, lead, or by the toxic effects of any number of drugs used for therapeutic purposes (cisplatin, nitrofurantoin, isoniazid, etc.). Occasionally other drugs, metals, and industrial solvents are incriminated; these are discussed in Chap. 43.
In the Western world, nutritional polyneuropathy is usually associated with chronic alcoholism. As indicated in earlier discussions, all data point to the identity or at least close relationship between alcoholic neuropathy and neuropathic beriberi. A nutritional factor is responsible for both, although in any given case it remains unclear whether the deficiency is one of thiamine, nicotinic acid, pyridoxine, pantothenic acid, folic acid, or a combination of these B vitamins. Our colleague M. Victor, who devoted considerable attention to this subject, was never persuaded of the existence of a form of polyneuropathy attributable solely to the toxic effect of alcohol, although claims of such an entity continue to be made and the perception persists among most physicians that alcohol is directly damaging to nerves. Nutritional neuropathy and other neurologic complications of deficiency disorders (Strachan syndrome, pellagra, vitamin B12 deficiency, and malabsorption syndromes) are described fully in Chap. 41. A predominantly sensory neuropathy with burning pain is typical of most forms of severe nutritional deprivation.
Although capable of producing diverse clinical presentations, most often the remote effect of cancer takes the form of a predominantly distal, symmetrical sensory, or sensorimotor polyneuropathy. Weakness and atrophy, ataxia, and sensory loss of the limbs may advance over several weeks or months to the point where the patient is confined to a wheelchair or bed; usually the CSF protein concentration is mildly elevated. All these symptoms may occur months or even a year or longer before a malignant tumor is found, although usually the tumor is apparent and most often is a lung cancer.
In most series, a mixed sensorimotor polyneuropathy has been 4 to 5 times more frequent than a purely sensory one. However, the latter is a more specific syndrome identified with lung cancer (described originally by Denny-Brown); it is characterized by a loss of all modalities of sensation spreading from the distal to the proximal segments of the limbs and eventually to the trunk and face. There is loss of tendon reflexes, but motor power may be retained. It has also been appreciated that the sensory loss in the beginning may have a multifocal distribution. Another variety is characterized by initial sensory ataxia, similar to that discussed in the earlier section “Acute Sensory Neuronopathy (Sensory Ganglionopathy).” The illness reaches its peak in a few weeks or months and in a very few instances the development has been as rapid as that of GBS.
The pathologic changes are those of an inflammatory and destructive sensory neuropathy and neuronopathy (ganglionitis) and are sometimes part of a more widespread disorder of the nervous system related to the anti-Hu antibody (also termed antinuclear neuronal antibody type 1 (ANNA-1); see Chap. 31). This polyneuropathy has proven to be most typical of small cell cancer of the lung. In a series of 71 patients with paraneoplastic sensory neuronopathy reported by Dalmau and colleagues, more than half were associated with symptomatic inflammatory lesions in the temporal lobes (limbic encephalitis), the brainstem, and, rarely, the anterior horn neurons of the spinal cord. Other distinctive paraneoplastic syndromes such as cerebellar degeneration and Lambert-Eaton myasthenic syndrome were combined with polyneuropathy in isolated cases and there were signs of dysautonomia in 28 percent. Our experience has been that most cases of the Lambert-Eaton syndrome have occurred in isolation, but there are many cases that exist in parallel with various neoplasms, including some of our patients with lymphomas of various types.
The CSF protein is mildly elevated but usually acellular. Sensory potentials are usually absent in all nerves after a few weeks, but may be spared early on. The localization of anti-Hu antibody to the several affected regions of the nervous system and to the tumor itself has led to speculation that the lung tumors are typically small or inevident because the antibody suppresses tumor growth. Almost all cases of paraneoplastic sensory neuropathy and a proportion of the more nondescript sensory predominant or sensorimotor paraneoplastic polyneuropathies also demonstrate anti-Hu antibodies, making this testing useful in distinguishing paraneoplastic varieties of sensory neuropathy and neuronopathy from those caused by postinfectious or immune disorders such as Sjögren syndrome and HIV infection. The finding of high antibody titers should lead to chest imaging and, in appropriate cases, bronchoscopic or positron emission tomography (PET) examinations to detect an underlying cancer. A rare vasculitic mononeuropathy multiplex that occurs with cancer is discussed further on.
An unusual assortment of polyneuropathies has been associated with non-Hodgkin lymphomas of both T- and B-cell types and with several related conditions, such as Castleman disease (angiofollicular lymphoid hyperplasia), intravascular T-cell lymphoma (and the related lymphomatoid granulomatosis; see Chap. 31), hypersensitivity lymph node hyperplasia (angioimmunoblastic or immunoblastic lymphadenopathy), and Kimura disease (lymphoid hyperplasia with eosinophilia mainly involving skin). In most of these neuropathies, particularly the one associated with Castleman disease, there is a paraproteinemia, often polyclonal, thereby relating this group to the paraproteinemic neuropathies and to osteosclerotic myeloma, discussed later. In several of our patients, the neuropathic manifestations appeared simultaneously with lymph node enlargement in the groin, axilla, or thorax. Clinically, the illness may take the form of GBS, chronic demyelinating polyneuropathy, subacute motor polyneuropathy or anterior horn cell disease, lumbar and brachial plexopathy, or a polyradiculopathy—each occurring as a paralymphomatous condition clearly separable from cases of meningeal and neural infiltration by tumor. Corticosteroids have been helpful in some of our patients with the lymphoid diseases; in others, the neuropathy resolves spontaneously or with radiation of the lymph nodes but otherwise progresses for months. Vallat and colleagues have summarized their experience with the more conventional types of neuropathy accompanying non-Hodgkin lymphoma. Intravascular lymphoma, a widespread neoplastic and vascular disease (described in Chap. 31), may infiltrate the peripheral nerves in a multiple mononeuropathy pattern.
The various forms of paraneoplastic polyneuropathy are manifest clinically in 2 to 5 percent of patients with malignant disease. The figures are higher if one includes the neuropathies accounted for by malnutrition and pressure palsies that occur in the later stages of cancer and those identified by EMG in asymptomatic patients (Henson and Urich). Carcinoma of the lung accounts for approximately 50 percent of the cases of paraneoplastic sensorimotor polyneuropathy and for 75 percent of those with pure sensory neuropathy (Croft and Wilkinson); nevertheless, these neuropathies may be associated with neoplasms of all types.
Although anti-Hu binds to the peripheral nerve, the immunopathology of the paraneoplastic polyneuropathies has not been completely defined. In the purely sensory type, there is not only a loss of nerve cells in the dorsal root ganglia but also an inflammatory reaction (Horwich et al)—much the same changes as occur with the sensory neuronopathy of Sjögren syndrome. In the mixed sensorimotor polyneuropathy, degeneration is greater in the distal than it is in the proximal segments of the peripheral nerves, but it extends into the roots in advanced cases. Dorsal root ganglion cells may be reduced in number in both types. If the histologic examination is performed early in the course of the neuropathy, sparse infiltrates of lymphocytes are observed distributed in foci around blood vessels. No tumor cells are seen in the nerves or spinal ganglia, unlike the rare instances of carcinomatous and lymphomatous mononeuropathy multiplex, in which tumor cells actually infiltrate nerves. Degeneration of the dorsal columns and chromatolysis of anterior horn cells are secondary to changes in the peripheral nerves and roots.
The prognosis of the paraneoplastic neuropathies is poor. Even though the polyneuropathy may stabilize or even remit to some extent on its own or with therapy, most patients succumb to the underlying tumor within a year.
If the tumor can be treated effectively, the neuropathy may improve, the exception being pure sensory neuronopathy, which rarely does so. Treatment with plasma exchange, gamma globulin, or immunosuppression has had only a minimal effect, but there are anecdotal reports of success with each of these treatments applied early in the course. In the report by Uchuya and colleagues, only 1 of 18 patients with a subacute sensory neuropathy improved and another became dependent for sustained improvement on immune globulin; most of the others stabilized or worsened and the authors concluded that treatment was of doubtful value. Corticosteroids have not been tested in a systematic way for paraneoplastic neuropathy and there is little clinical evidence to support their use.
Of the neuropathies caused by metallic poisoning, that caused by arsenic is particularly well characterized. In cases of chronic poisoning, the neuropathic symptoms develop rather slowly, over a period of several weeks or months and have the same sensory and motor distribution as the nutritional polyneuropathies. Gastrointestinal symptoms, the result of ingestion of arsenic compounds, may precede the polyneuropathy, which is nearly always associated with anemia, jaundice, brownish cutaneous pigmentation, hyperkeratosis of palms and soles, and later with white transverse banding of the nails (Mees lines). The disease is accompanied by an excess of arsenic in the urine and hair. Pathologically, this form of arsenical neuropathy is categorized as of the dying-back (axonal degeneration) type.
In patients who survive the ingestion of a single massive dose of arsenic, a more rapidly evolving polyneuropathy may appear after a period of 8 to 21 days as discussed earlier. Diagnosis and treatment of arsenical poisoning are discussed further in Chap. 43. Here it is emphasized that the ingestion of fish in many areas of the industrialized world gives high levels of blood and urine arsenic, but the metal is in the form of arsenobetaine, which has low toxicity and does not cause neuropathy.
This is an uncommon disorder. In adults, it occurs following chronic exposure to lead paint or fumes (from smelting industries or burning batteries) or from ingestion of liquor distilled in lead pipes. Its most characteristic presentation is a motor mononeuropathy in the distribution of the radial nerves (wrist and finger drop). In a few personally observed patients this was the main abnormality, but there was also a sensory loss in the radial territory of the hand. Less commonly, there is foot-drop occurring alone or in combination with weakness of the proximal arm and shoulder girdle muscles. As pointed out in Chap. 43, lead neuropathy seldom occurs in children, in whom poisoning usually results in an encephalopathy. Although the neuropathy has been known since ancient times, details of the pathobiology are still obscure. Axonal degeneration with secondary myelin change and swelling and chromatolysis of anterior horn cells has been described. Lead accumulates in the nerve and may be toxic to Schwann cells or to endothelial capillary cells, causing edema.
The diagnosis is established by the history of lead exposure, the predominant and restricted motor involvement, associated medical findings (anemia, basophilic stippling of red blood cell precursors in the bone marrow, a “lead line” along the gingival margins, colicky abdominal pain, and constipation), and the urinary excretion of lead and coproporphyrins. Blood lead levels of more than 70 μg/dL are always abnormal. In patients with lower levels, doubling of the 24-h urinary lead excretion following an infusion of the chelating agent CaNa2 ethylenediaminetetraacetic acid (EDTA) indicates a significant degree of lead intoxication. Coproporphyrin in the urine is abnormal in any amount, but it may also be found in porphyria, alcoholism, iron deficiency, and other disorders as well as in lead intoxication.
Treatment consists of terminating the exposure to lead and eliminating lead from the bloodstream and the bones by chelation as discussed in Chap. 43. For this purpose, penicillamine, which is generally safe and can be administered orally, is preferable to dimercaprol (British anti-Lewisite [BAL]) or EDTA.
Chronic poisoning with thallium and sometimes with lithium, gold, mercury, and platinum (in the antineoplastic agents cisplatin and carboplatin as discussed further on) produces a sensorimotor polyneuropathy; these intoxications are discussed in Chap. 43 and the acute form was addressed earlier in the chapter. A predominantly motor neuropathy is induced by occupational exposure to metallic mercury and mercury vapor but any connection to the mercury content in dental amalgam has little credibility. Exposure to manganese, bismuth, antimony, zinc, and copper may give rise to systemic signs of poisoning; some of them affect the central nervous system (CNS) but one cannot be certain that any of them specifically involves peripheral nerves. The devastating encephalopathy of organic mercury toxicity does not, to our knowledge, cause neuropathy.
As mentioned in Chap. 43, a predominantly motor polyneuropathy has been reported as a rare complication of gold therapy for rheumatoid arthritis. Most often the cumulative dose of gold had exceeded 1 g but in a few instances the neuropathy occurred with 0.5 g. Painful distal burning is the initial complaint with weakness and wasting following. The onset of weakness, although usually insidious, can be abrupt enough to simulate GBS. There have been trigeminal, facial, and oculomotor palsies. One of the unusual features, not shared with most other toxic neuropathies, is a marked rise in CSF protein concentration.
A distal, symmetrical sensorimotor (predominantly sensory) neuronopathy may follow exposure to certain hexacarbon industrial solvents. These include n-hexane (found in contact cements, thus affecting “glue sniffers” who inhale the vapors); methyl n-butyl ketone (used in the production of plastic-coated and color-printed fabrics); dimethylaminopropionitrile (DMAPN), used in the manufacture of polyurethane foam); the fumigant methyl bromide; and the gas sterilant ethylene oxide. Operating room nurses may be affected by the latter when the agent is absorbed through the skin, leaving a characteristic rash at exposed sites (usually the wrists, where a surgical gown ends). A mild peripheral neuropathy and CNS changes of memory loss and headaches have been reported from this agent by Brashear and colleagues. Nurses are also subject to a risk of nitrous oxide neurotoxicity and this usually takes the form of a myelopathy similar to that seen with cobalamin deficiency. Most cases are caused by repeated use of the gas to induce euphoria. As with vitamin B-12 deficiency, the syndrome may be mistaken for a neuropathy but nerve conduction studies fail to demonstrate one. The associated macrocytic anemia is reversed by the administration of B12, but the neurologic illness may be less responsive as discussed in Chap. 41.
Triorthocresyl phosphate and acrylamide are potent peripheral nerve toxins. Both of these drugs cause a dying-back polyneuropathy with axonal degeneration and have been used experimentally to produce this effect. Vacor, a phenylnitrosourea rodenticide, taken as a suicidal agent, gives rise to a profound sensory and autonomic neuropathy with abdominal pain and hyperglycemia caused by acute pancreatitis.
Detailed accounts of the clinical and experimental neurotoxicology of these agents can be found in the monograph by Spencer and colleagues.
A large number of medications are potential sources of polyneuropathy of predominantly sensory type. Most are dose-dependent and are therefore more or less predictable after large cumulative doses of the drug have been given (e.g., in cancer chemotherapy) or after prolonged administration for other reasons. A more complete list than can be compiled here can be found in the review by England and Asbury.
(See also Chap. 43.) Among chemotherapeutic agents in current use, particularly cisplatin, carboplatin, and bortezomib, are known to evoke a dose-dependent, predominantly sensory polyneuropathy, which begins several weeks after completion of therapy in at least half of the patients. Proprioception and vibratory sensation are most severely impaired. Some patients develop acrodynia and episodic color changes in the fingertips and toes suggesting that autonomic nerves are also involved; in severe cases there is sensory ataxia and pseudoathetosis. The severity of histopathologic changes in the peripheral nervous system corresponds to the concentration of platinum in these tissues, the highest being found in dorsal root ganglia. Secondary degeneration in the posterior columns is the basis for a Lhermitte symptom reported by some patients.
The taxanes paclitaxel and the more potent docetaxel, both cited as inhibitors of the depolymerization of neurotubules, are used mainly in the treatment of ovarian cancer. They produce a sensory polyneuropathy similar to that of cisplatin. The nerve lesion regresses slowly with a reduction in dosage. Pathologic studies have shown a neuronopathy and distal axonopathy affecting mainly large fibers.
For decades it has been known that peripheral neuropathy commonly complicates the use of vincristine, an antineoplastic agent most widely used in treatment of the lymphomas and leukemia. Paresthesias are the most common early symptom, and loss of ankle jerks is an early sign. Some degree of weakness usually precedes objective sensory loss; the extensor muscles of the fingers and wrists are affected; later the dorsiflexors of the toes and feet causing foot-drop or, more often in our experience, foot-drop may appear first. With the dose regimens currently used, the weakness is usually mild, but in the past, some patients became quadriparetic and bedbound. Adults are more severely affected than are children, as are persons with preexisting polyneuropathies. The neuropathy is strictly dose-related and reduction in dosage is followed by improvement of neuropathic symptoms although this may take several months. Many patients are then able to tolerate vincristine in low dosage, such as 1 mg every 2 weeks, for many months. Thalidomide produces a similar sensory neuropathy. It is finding use in the treatment of inflammatory conditions such as Behçhet disease, graft versus host reactions, erythema nodosum, lepromatous eruptions, aphthous stomatitis in AIDS patents and highly vascular tumors certain tumors such as renal cell cancer.
As mentioned in Chap. 43, isoniazid (INH)-induced polyneuropathy was a common occurrence in the early 1950s when this drug was first used for the treatment of tuberculosis. Symptoms of neuropathy appeared between 3 and 35 weeks after treatment was begun and affected approximately 10 percent of patients receiving therapeutic doses in the upper range (10 mg/kg daily). The initial symptoms are symmetrical numbness and tingling of the toes and feet spreading, if the drug is continued, to the knees and occasionally to the hands. Aching and burning pain in these parts then becomes prominent. In addition to sensory loss, examination usually discloses a loss of tendon reflexes and weakness in the distal muscles of the legs. Severe degrees of weakness and loss of deep sensation are observed only rarely.
Isoniazid produces its effects on the peripheral nerves by interfering with pyridoxine metabolism, perhaps by inhibiting the phosphorylation of pyridoxine (the collective name for the B6 group of vitamins) and decreasing the tissue levels of its active form, pyridoxal phosphate. The administration of 150 to 450 mg of pyridoxine daily in conjunction with the isoniazid completely prevents the neuropathy. The same mechanism is probably operative in the neuropathies that occasionally complicate the administration of the isoniazid-related substances such as ethionamide, used sometimes in the treatment of tuberculosis and the now little-used antihypertensive agent hydralazine. Paradoxically, the taking of extremely high doses of pyridoxine over a prolonged period may actually cause a disabling, predominantly sensory ganglionopathy (Schaumburg et al, 1983).
A relatively mild sensory neuropathy (acral paresthesia) associated with optic neuropathy occasionally complicates chloramphenicol therapy. The chronic administration of metronidazole may have the same effect (and can produce lesions in the deep cerebellum). The newer antimicrobial, linezolid, has been associated with a fairly severe sensory neuropathy in a few cases after prolonged use. A predominantly motor neuropathy has been reported with the chronic administration of dapsone, a sulfone used to treat leprosy and certain dermatologic conditions. Stilbamidine, used in the treatment of kala azar, may also induce a purely sensory neuropathy with a propensity to affect the trigeminal nerves.
The introduction, in 1952, of nitrofurantoin for the treatment of bladder infections was soon followed by reports of neurotoxicity attributable to the drug. The earliest symptoms are pain and tingling paresthesias of the toes and feet, followed shortly by similar sensations in the fingers. If the drug is not discontinued, the disorder progresses to a severe, symmetrical sensorimotor polyneuropathy. Patients with chronic renal failure are particularly prone to neurotoxicity from nitrofurantoin because of diminished drug excretion resulting in high tissue levels. To make matters more complex, the uremic state itself may be responsible for a polyneuropathy so that the distinction between uremic and nitrofurantoin neuropathy may be impossible. The neuropathologic studies of Lhermitte and colleagues disclosed an axonal degeneration in peripheral nerves and sensory roots.
Amiodarone, a drug used for treating recalcitrant ventricular tachyarrhythmias, induces a motor-sensory neuropathy in about 5 percent of patients after several months of treatment. It may also cause a toxic myopathy. Perhexiline maleate for the treatment of angina pectoris may also cause a generalized, predominantly sensory polyneuropathy in a small proportion of patients. Hydralazine as a neurotoxic agent has already been mentioned. Affected persons show a striking neuronal lipidosis. Patients taking niacin to lower blood cholesterol levels may experience distal and truncal paresthesias, but an associated neuropathy has been identified.
The development of a sensorimotor neuropathy similar to that produced by INH may be associated with the chronic use of disulfiram in the treatment of alcoholism. Its neurotoxic effects have been attributed to the action of carbon disulfide, which is produced during the metabolism of the drug, and is known to cause polyneuropathy and sometimes an optic neuropathy in workers in the viscose rayon industry. Pathologic data, although scant, tend to discredit this notion, insofar as disulfiram evokes a wallerian type of axonal degeneration, whereas carbon disulfide neuropathy is characterized by swollen (giant) axons that are filled with neurofilaments (Bouldin et al).
Some patients who have taken phenytoin for decades may lose ankle and patellar reflexes and acquire mild distal symmetrical impairment of sensation, slowed conduction velocity in the peripheral nerves of the legs and rarely, weakness of the distal musculature. The mechanism and frequency of this complication are not clear. The cholesterol-lowering statin drugs have been tentatively implicated in a painful, paresthetic distal axonal polyneuropathy with retained reflexes (Gaist et al). More often the problem with statins is one of a toxic myopathy. The frequency of polyneuropathy is low but, if no other explanation is identified, it may be advisable to discontinue the drug. Colchicine has long been known to cause a myopathy, but a few cases of predominantly axonal sensory neuropathy have also been reported (neuromyopathy).
Among various other agents that cause neuropathy are hydroxychloroquine and colchicine are known to cause a toxic neuropathy. The anesthetic agent trichloroethylene, as with the aforementioned stilbamidine, has a predilection for cranial nerves, particularly the fifth. The neurotoxicity is apparently caused by dichloroacetylene, formed as a product of trichloroethylene. The neuropathic potential of nitrous oxide has already been mentioned. Most of the group of TNF-alpha (TNF-α) inhibitors may cause a polyneuropathy, but this category of agents do not appear to have a direct toxic effect on nerves but instead alter immune function in some way that results in a process that simulates chronic inflammatory demyelinating polyneuropathy (see further on).
Residual effects of polyneuropathy were seen in patients with the toxic eosinophilia-myalgia syndrome; the problem was traced to the ingestion of adulterated L -tryptophan, which had been used in nonprescription drugs for insomnia. One patient under our care suffered permanent areflexic quadriplegia. There may be an eosinophilic infiltrate in nerves, but the neuropathy is probably the result of a direct toxic mechanism. A sensory neuropathy, resulting from excessive pyridoxine ingestion alluded to earlier, is still seen among individuals who take huge doses of vitamin supplements. Amitriptyline is capable of producing paresthesias, but the effect seems to be idiosyncratic and infrequent.
Diabetes mellitus is the most common cause of polyneuropathy in general clinical practice, for which reason it is accorded a separate section. We are referring mainly to a generalized, predominantly sensory syndrome, but several focal or regional forms of peripheral nerve disease also result from diabetes and for convenience of exposition, are included here. In recent years, attention has also been directed to a possible association between a nondescript sensory polyneuropathy and impaired glucose tolerance, even without manifest diabetes, persistent hyperglycemia, or an elevation of hemoglobin A1c. The survey by Sumner and colleagues makes a case for such an association, but we remain uncertain about the relationship between glucose intolerance alone and polyneuropathy. By statistically adjusting for relevant factors such as glycemic control and glycosylated hemoglobin, Tesfaye and colleagues have suggested that some cardiovascular risk factors subsumed under the term “metabolic syndrome” (triglyceride levels, body mass, hypertension) are themselves risk factors for diabetic polyneuropathy.
Approximately 15 percent of patients with diabetes have symptoms and signs of polyneuropathy, but nearly 50 percent of cross-sectional population samples have evidence of peripheral nerve damage as judged by nerve conduction abnormalities. The duration of diabetes is perhaps the most important factor. Fewer than 10 percent of patients have clinically evident polyneuropathy at the time of discovery of diabetes, but this figure rises to 50 percent after 25 years. The presence of diabetic retinopathy is associated with higher incidences of neuropathy. It is not surprising, therefore, that neuropathy is most common in diabetics older than 50 years; it is infrequent in those younger than age 30 years and is rare in childhood. Dyck and colleagues (1993) studied diabetics in Rochester, Minnesota, and found that 54 percent with type 1 (insulin-deficient) and 45 percent with type 2 (insulin-resistant) had polyneuropathy. The percentages were lower when patients were selected on the basis of clinical symptoms alone rather than on the presence of changes in nerve conduction; close to 15 percent at the time of diagnosis in both groups. In the syndromes described further on, both type 1 and type 2 diabetic patients are susceptible, the duration of diabetes being a major factor.
Several fairly distinct clinical syndromes of diabetic neuropathy have been delineated: (1) the most common as noted is a distal, symmetrical, primarily sensory polyneuropathy affecting feet and legs in a chronic, slowly progressive manner; the others are (2) acute ophthalmoplegia that affects the third, and less often the sixth, cranial nerve on one side; (3) acute mononeuropathy of limbs or trunk including a painful thoracolumbar radiculopathy; (4) an acute or subacute painful, asymmetrical, predominantly motor, multiple neuropathy affecting the upper lumbar roots and the proximal leg muscles (“diabetic amyotrophy”); (5) a more symmetrical, proximal motor weakness and wasting, usually without pain and with variable sensory loss, pursuing a subacute or chronic course; and (6) an autonomic neuropathy involving bowel, bladder, sweating and circulatory reflexes. These forms of neuropathy often coexist or overlap, particularly the autonomic and distal symmetrical types and the subacute proximal neuropathies.
Most of the syndromes listed here are likely to be a result of ischemia or infarction of nerves or nerve fascicles, because of a diabetic microvasculopathy. All but the first are special types of mononeuropathy multiplex. The polyneuropathy is associated with occlusion of small endoneurial blood vessels (vasonervorum) but possibly also incorporate a poorly understood metabolic abnormality; however, other theories of causation abound. In recent years, an inflammatory process has been postulated as yet another mechanism of peripheral nerve damage. These aspects are discussed further on.
The distal, symmetrical, primarily sensory form of polyneuropathy is the most common type. It is usually a chronic process, sometimes unnoticed by the patient. The main complaints are persistent and often distressing numbness and tingling, usually confined to the feet and lower legs and worse at night. The ankle jerks are absent and, sometimes, the patellar reflexes as well. As a rule, sensory loss is confined to the distal parts of the lower extremities, but in severe cases the hands are involved and the sensory loss may even spread to the anterior trunk, simulating a sensory level of spinal cord disease (Said et al, 1983). Trophic changes in the form of deep ulcerations and neuropathic degeneration of the joints (Charcot joints) are encountered in the most severe and long-standing cases, presumably as a result of sensory analgesia, trophic changes, and repetitive injury. (Foot ulcerations are more common simply as a result of the microvascular disease of skin in diabetic patients.) Muscle weakness is usually mild, but in some patients a distal sensory neuropathy is combined with a proximal weakness and wasting of the types mentioned earlier. Treatment of the acral pain may be a major problem and is discussed further on.
In another group of patients with diabetic polyneuropathy the clinical picture may be dominated instead by loss of deep sensation, ataxia, and atony of the bladder, with only slight weakness of the limbs, in which case it resembles tabes dorsalis (hence the term diabetic pseudotabes). The similarity to tabes dorsalis is even closer if lancinating pains in the legs, unreactive pupils, abdominal pains, and neuropathic arthropathy are present.
Among these, diabetic ophthalmoplegia is a common occurrence, usually in a patient with well-established diabetes. It commonly presents as isolated, painful third nerve palsy with sparing of pupillary function. In the first autopsied patient reported by Dreyfus and colleagues, there was an ischemic lesion in the center of the retroorbital portion of the third nerve. Subsequently, a similar case was described by Asbury and colleagues (1970). Slightly less often, the sixth nerve on one side is involved. The disorder was described earlier in Chap. 14.
Isolated involvement of practically all the major peripheral nerves has been described in diabetes, but the ones most frequently affected are the femoral, sciatic, and peroneal nerves, in that order. Rarely is a nerve in the upper extremity affected. As mentioned, the acute mononeuropathies, both cranial and peripheral, are presumably a result of infarction of the nerve, but it is only in pathologic studies of the third nerve that this basis has been established. Recovery is the rule but may take many months.
Diabetic Multiple Mononeuropathies and Radiculoplexus Neuropathy (Diabetic Lumbar Plexopathy; Diabetic Amyotrophy; Garland Syndrome)
This category overlaps with the mononeuropathies. A syndrome of painful unilateral or asymmetrical multiple neuropathies tends to occur in older patients with relatively mild or even unrecognized diabetes. Multiple nerves are affected in a random distribution (mononeuropathy multiplex). The mononeuropathies often emerge during periods of transition in the diabetic illness, for example, after an episode of hyper- or hypoglycemia, when insulin treatment is initiated or adjusted, or when there has been rapid weight loss.
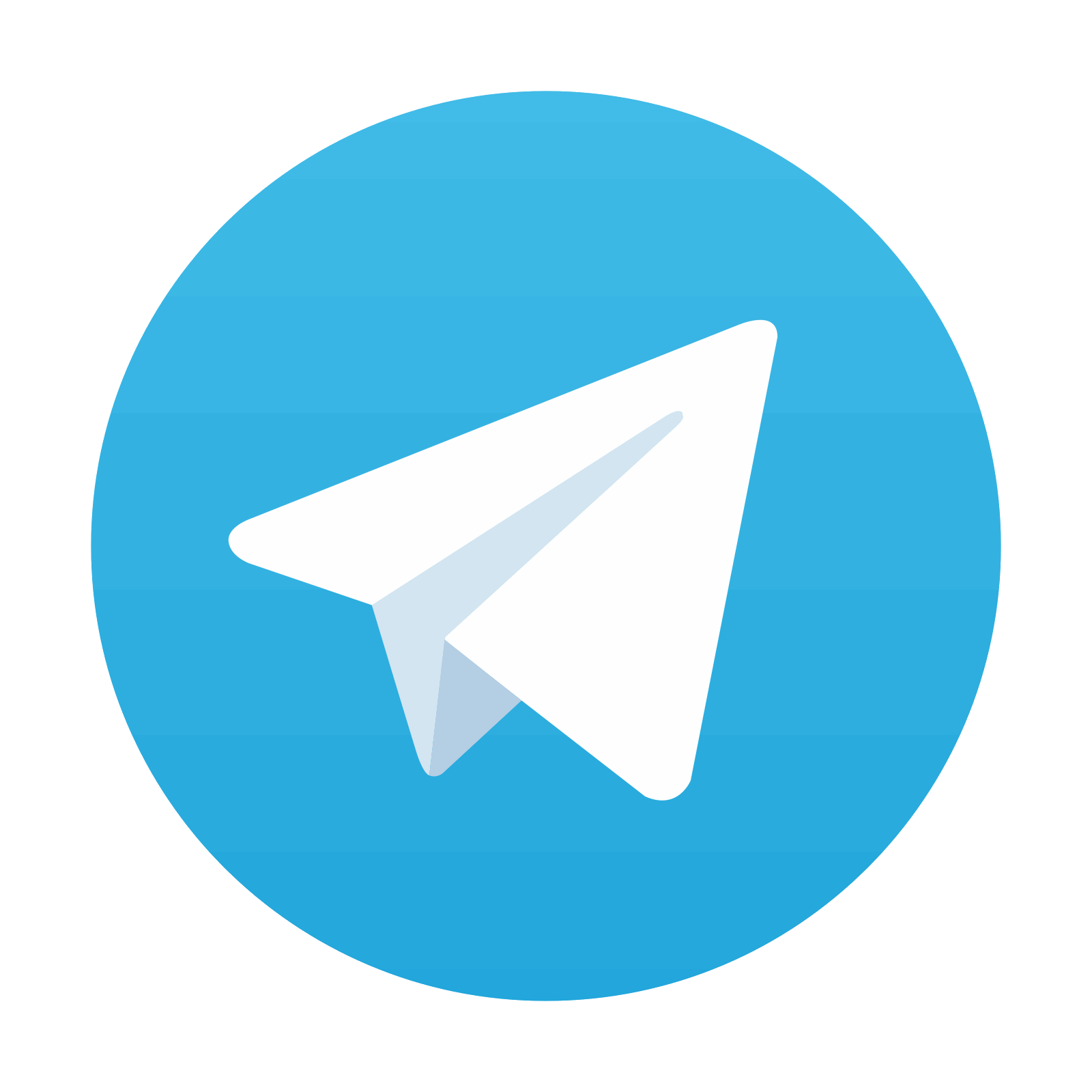
Stay updated, free articles. Join our Telegram channel

Full access? Get Clinical Tree
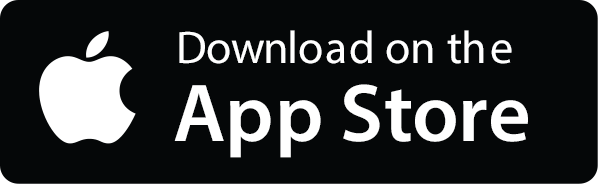
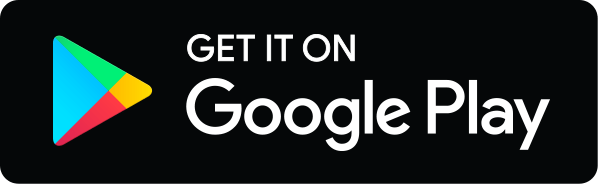