Chapter 12 Circadian Clock Genes
Abstract
The Mammalian Cellular Circadian Clock
Several lines of evidence pointed to the suprachiasmatic nucleus (SCN) as the site of the master circadian pacemaker beginning in the 1970s. Destruction of the SCN abolishes circadian oscillations in the plasma concentration of cortisol1 and in locomotion and drinking.2 These oscillations are independent of inputs from the eye,1 although an autonomous circadian clock has been demonstrated to exist within the eye that controls, among other functions, the shedding of rod outer segment disks.3 Normal circadian rhythms can be restored to an SCN-lesioned animal by transplantation of fetal SCN tissue but not by transplantation of fetal tissue from other regions of the brain.4 Transplant into an SCN-lesioned animal of fetal tissue from the SCN of a circadian mutant animal confers the short period of the donor,5 indicating that the properties of the rhythm are determined by the SCN rather than other tissues or brain regions. Thus, several lines of evidence point to the SCN as the site driving or controlling circadian behavior for mammals.
Recent studies of rhythms in gene expression have indicated that persistent rhythms can be observed in tissues throughout the organism, even in tissue explants kept in culture for extended periods of time.6,7 The phase of these peripheral tissue rhythms differs from that of the SCN, but nonetheless it appears to be coordinated by the SCN. In SCN-lesioned animals, these peripheral rhythms persist but no longer exhibit the consistent phase seen in un-lesioned animals.7 Some environmental manipulations, such as temperature cycles or restricted feeding, can alter the phase of peripheral rhythms.8,9 In addition, studies confirm the presence of oscillations in gene expression throughout the body, with different phases in different tissues.10–13 Loss of many of these rhythms is reported with SCN lesions. However, these studies cannot discriminate between a loss of rhythmicity by individual animals and a loss of synchronicity among the individuals. Thus the roles of the SCN and of the peripheral oscillators in the mammalian circadian system continue to be defined.
Circadian Clock Properties and Clock Genes
Half a century ago, the formal properties of the circadian clock function had been well defined. Included among the 16 “empirical generalizations about circadian rhythms” defined by Colin Pittendrigh14 were that circadian rhythms are ubiquitous in living systems; they are endogenous; they are innate, they are not learned from or impressed by the environment; they occur autonomously at both cell and whole-organism levels of organization; the free-running period of circadian rhythms are so slightly temperature-dependent that it is proper to emphasize their near independence of temperature; and they are surprisingly intractable to chemical perturbation. These six observations already suggested what we now know to be the case: A cell-autonomous program of gene expression makes up the mammalian circadian clock that produces these rhythms.
Indeed, it appears that the synthesis of proteins by each oscillatory cell is central to the mechanism for the generation of 24-hour rhythms. The initial evidence for this is that application of protein synthesis inhibitors in the region of the SCN shifts the circadian phase of activity of animals by an amount and in a direction that depends upon the time when the inhibition is imposed.15,16 A similar shift in the phase of vasopressin release from explanted SCN also results from inhibition of protein synthesis.17 Thus, gene expression is central to the generation of circadian oscillations.
Gene expression profile studies, in which expression levels are sampled at regular time points in constant darkness (free-running conditions) focusing on the SCN and on peripheral tissues reveal that approximately one third of the transcriptome is rhythmically expressed, even in peripheral tissues.10–13 Reporter gene constructs combining genes known to be rhythmically expressed with luciferase (thus allowing visualization of expression in culture via the luciferase’s glow) demonstrated in rats and in mice that peripheral tissues are capable of self-sustained rhythms.6,7 With so many genes exhibiting circadian expression, and competent oscillators present in such a variety of tissues, one cannot assume that either rhythmic expression or expression in the SCN are valid criteria for a clock gene. Identification of which genes are central to the generation and maintenance of circadian cycles thus represents a challenge.
A potential solution to the challenge of identification of clock genes (as distinct from clock-controlled genes) is to refocus attention on the formal properties of the circadian system. Arnold Eskin and others promoted this conceptual framework in the late 1970s by characterizing rhythm properties as arising from the input pathway, the clock itself, or the output pathway (Fig. 12-1).18 As articulated for pharmacologic approaches (but equally valid for genetic ones), manipulations that produce phase-dependent shifts in the rhythm (a phase-response curve), or changes in the phase-response curve or the free-running period are likely to be affecting the clock, or at least not affecting an output process.19 Applying this logic, a genetic perturbation that alters the free-running period in constant darkness, or the phase-response curve to light pulses, or the persistence of rhythmicity in constant conditions is likely to be a perturbation in a clock gene, although no one alteration alone is necessarily a clock change (rather than input or output).
Zatz and others20,21 proposed more restrictive criteria: Null mutations should abolish rhythmicity, the gene’s protein product level or activity should oscillate and be reset by light pulses, changes in amount or activity should result in phase shifts, and prevention of oscillation of protein levels or activity should result in loss of rhythmicity.21 In the parlance of the field, these criteria would define a state variable—a rate-limiting element that itself defines the phase of the core oscillation. A self-sustaining clock would require at least two state variables,22 although more are clearly possible. To date, no single gene in the mammalian system has satisfied all the criteria for a state variable. Indeed a hallmark of the mammalian circadian clock seems to be the multiple homologues of many genes that appear to play related but nonredundant roles (Fig. 12-2). It may thus be that “state variable” status is actually shared by related groups of genes in the mammalian system.
Positive Elements
Clock
In the early 1990s, no genes in mammals had been identified as even possible candidate circadian clock genes, leading us to undertake a mutagenesis and screening in mice in an effort to identify mammalian circadian clock genes. For this, we used the C57BL/6J mouse strain, in which wild-type mice show robust entrainment to a light–dark cycle and have a circadian period between 23.6 and 23.8 hours under free-running conditions in constant darkness (DD). In a screen for mutations of more than 300 progeny of mutagen-treated mice, we found one animal that had a free-running period of about 24.8 hours, more than six standard deviations longer than the mean.23 In the homozygous condition this mutation results in a dramatic lengthening of the period to about 28 hours, which is usually followed by the eventual loss of circadian rhythmicity (i.e., arrhythmicity) after about 1 to 3 weeks in DD. The affected gene was mapped to mouse chromosome 5 and named Clock.23,24
We cloned the Clock gene by a combination of genetic rescue and positional cloning techniques. Clock/Clock mutant mice were phenotypically rescued by a bacterial artificial chromosome (BAC) transgene that contained the Clock gene, allowing functional identification of the gene.25 The Clock gene encodes a transcriptional regulatory protein having a basic helix-loop-helix DNA-binding domain, a PAS dimerization domain, and a Q-rich transactivation domain. The mutant form of the CLOCK protein (CLOCK Δ19) lacks a portion of the activation domain found in wild-type protein, and thus, although it is capable of protein dimerization, transcriptional activation is diminished or lost. The PAS domain is so named because of the genes originally identified with this protein dimerization domain, per, ARNT, and sim. Clock mRNA is expressed in the SCN as well as other tissues, but it has not been found to oscillate in a circadian fashion.26
Bmal1
The presence of the PAS dimerization domain in CLOCK protein suggested that it might form a heterodimer similar to that of PER and the protein product of another Drosophila clock gene, TIM.27 A screen for potential partners for the CLOCK protein using the yeast two-hybrid system revealed that a protein of unknown function, BMAL1 (Brain and Muscle ARNT-Like 1), was able to dimerize with the CLOCK protein.28 Creation of mice harboring a null allele of Bmal1 (also referred to as MOP3) demonstrated the critical role of this gene in generating circadian rhythm. These mutant mice, which display light–dark responsive differences in activity level, become arrhythmic immediately upon release in constant darkness.
Additional actions of the CLOCK:BMAL1 heterodimer have become clear. Although Clock mRNA does not oscillate, its protein’s nuclear versus cytoplasmic localization does.29 By studying the intracellular localization of CLOCK and BMAL1 in fibroblasts of mouse embryos with mutations in different clock genes, and ectopically expressing the proteins, it was found that nuclear accumulation of CLOCK depended on formation of the CLOCK:BMAL1 dimer, as was phosphorylation of the complex and its degradation.29 Other PAS domain–containing proteins failed to affect the localization of CLOCK, indicating that these posttranslational events are specific to the CLOCK:BMAL1 dimer.
Negative Elements
Period Genes
As described in Chapter 13, the first identified gene (defined as a mendelian gene as opposed to a sequenced, cloned gene) that encodes a clock component, period, denoted with the symbol per, was discovered in 1971 in Drosophila using a forward genetic approach consisting of chemically inducing random mutations in the genome and then detecting the mutations that affect circadian rhythms by screening the progeny of the mutagenized individuals for altered rhythmicity.30 This approach has the advantage that no assumptions are made about the nature of the genes or gene products involved, but it is based on the presumption that there exist genes that, when mutated, will alter rhythms in a detectable manner. At the time, this presumption of the existence of genes that regulate a complex behavior was considered radical, but it has proved to have been a field-defining moment.
Initially, three alleles of the per gene were identified by the process of mutagenesis and screening. Flies carrying these alleles had either no apparent rhythm in eclosion (emergence from the pupal case) or locomotion, or they had either long (e.g., 29 hours) or short (e.g., 19 hours) periods for the rhythms of eclosion and locomotor activity.30 The finding of three alleles with three different phenotypes made it possible to have confidence in the conclusion that the per gene encodes a protein that is a clock component. Had only an arrhythmic mutant been found, then the alternative explanation could be proposed that the lack of circadian behavior was secondary to another primary defect that did not lie in a clock component. The approach of mutagenesis and screening has also been successful in identifying circadian clock genes in other organisms such as Neurospora crassa,31 plants31 and cyanobacteria.33
Confirmation of the importance of the per gene as a central circadian clock component was the rescue of the mutant phenotype after introduction of the wild-type allele of the per gene into mutant flies.34,35 The level of the mRNA transcript encoded by the per gene was shown to oscillate in a circadian fashion36 as a result of transcriptional regulation,37 and the levels of the PER protein were shown to lag the per mRNA levels.38 In fact, shifts in the circadian phase can be evoked by the induction of PER protein under the control of a noncircadian promoter.39 Thus, many lines of evidence indicate that the per gene encodes a protein that is a clock component. Three orthologues of the per gene—mPer1, mPer2, and mPer3—have now been identified in the mouse, and the levels of their mRNA have also been shown to oscillate with a circadian period.40–44
Following the identification of CLOCK:BMAL1 dimerization, the ability of this heterodimer to regulate transcription was tested using a reporter construct based on the upstream regulatory elements of the per gene. The per gene of Drosophila contains an upstream regulatory element, the clock control region, within which is contained a sequence needed for positive regulation of transcription, the E-box element (CACGTG).45 CLOCK-BMAL1 heterodimers were found to activate transcription of the mPer gene in a process that requires binding to the E-box element.28 However, CLOCK Δ19 mutant protein was not able to activate transcription, consistent with the finding that exon 19, which is skipped in Clock mutant animals,26 is necessary for transactivation. Thus, CLOCK protein interacts with the regulatory regions of the per gene to allow transcription of the per mRNA and eventual translation of PER protein. A similar activation of transcription of the tim gene by the CLOCK-BMAL1 heterodimer also occurs in flies.46 However, this positive regulation alone does not produce an oscillation in per mRNA levels, which is known to be responsible for the oscillation in PER protein levels.37
Findings that the Clock mutation dramatically decreases per genes’ expression also confirms the positive regulation of CLOCK:BMAL1 on per transcription in situ.47,48 Mice with null mutations of mPer1, mPer2, or mPer3 alone display altered circadian periods,49,50 and mice with both mPer1 and mPer2 null mutations lose rhythmicity. mPer3 null mutant mice exhibit only a subtle alteration in rhythmicity, and mPer1/mPer3 or mPer2/mPer3 double mutants are not substantially distinct from the mPer1 or mPer3 single mutants. These findings suggest there may be some compensation of function among the different mammalian per genes, and raise the question of the significance of mPer3 for the generation of mammalian circadian rhythms.
Cryptochromes
Cryptochromes are blue light–responsive flavoprotein photopigments related to photolyases, so named because their function was cryptic when first identified. In mammals, two cryptochrome genes, Cry1 and Cry2, have been identified. They were found to be highly expressed in the ganglion cells and inner nuclear layer of the retina as well as the SCN,51 and their mRNA expression levels oscillate in these tissues. Targeted mutant mice lacking Cry2 exhibit a lengthened circadian period, and mice lacking Cry1 have shortened circadian period; mice with both mutations have immediate loss of rhythmicity upon transfer to constant darkness.52–54 Thus, like the mammalian period genes, the cryptochrome genes appear to have both distinct (given their opposite effects on circadian period) and compensatory (given that either gene can sustain rhythmicity in the absence of the other) functions.
Because of their expression pattern, the cryptochromes were thought to be the long-unidentified mammalian circadian photoreceptors (see later), and thus light responses were examined in characterizing the null mutants. Cry2 mutant mice exhibit altered phase shifting responses to light pulses.52 Cry1/Cry2 double mutants exhibit impaired light induction of mPer1 in the SCN, but light induction of mPer2 in double mutants remains.53,55 Neither mPer1 nor mPer2 exhibit persistent oscillations in expression in the SCN in constant conditions in Cry1/Cry2 double mutants.53,55 Thus, although the cryptochromes are not the mammalian circadian photoreceptor, they do appear to play a central role in the generation of circadian signals.
Further evidence for a central clock function is the finding that the cryptochromes appear to share a number of regulatory features with the period genes. In Clock mutant mice, the mRNA levels of Cry1 and Cry2 are reduced in the SCN and in skeletal muscle,56 suggesting that the cryptochromes also are induced by CLOCK:BMAL1 transactivation. Using mammalian (NIH 3T3 or COS7) cell lines, CRY1 and CRY2 were found by co-immunoprecipitation to interact with mPER1, mPER2, and mPER3, leading to nuclear localization of the CRY:PER dimer as indicated by co-transfection assays with epitope-tagged proteins.56 Luciferase assays indicate that CRY:CRY or CRY:PER complexes were capable of inhibiting CLOCK:BMAL1 transactivation of mPer1 or vasopressin transcription.56 Thus, the CRYs as well as the PERs are capable of a negative feedback function, inhibiting CLOCK:BMAL1-induced transcription.
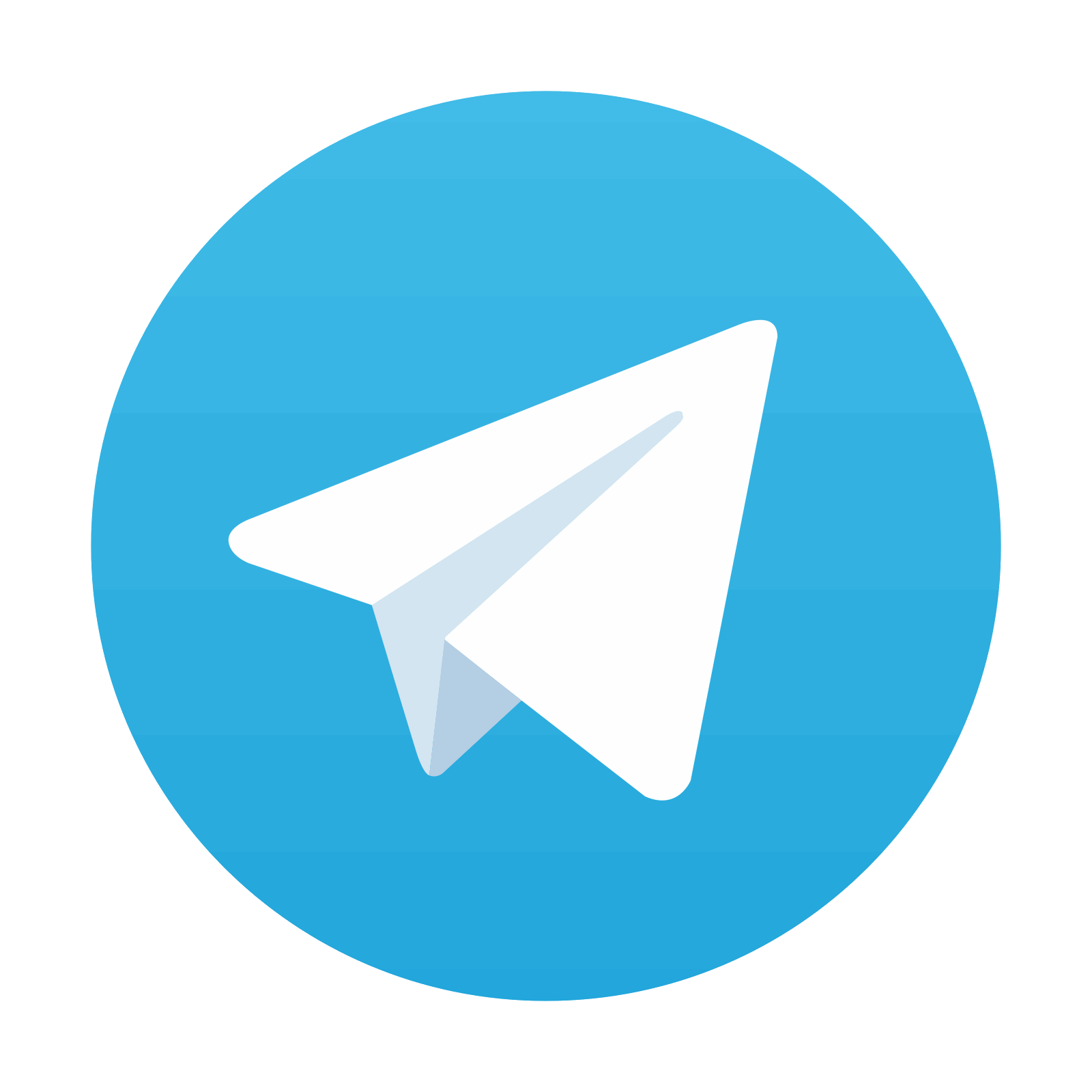
Stay updated, free articles. Join our Telegram channel

Full access? Get Clinical Tree
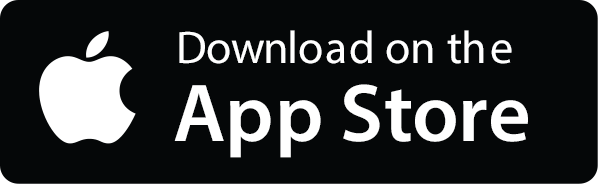
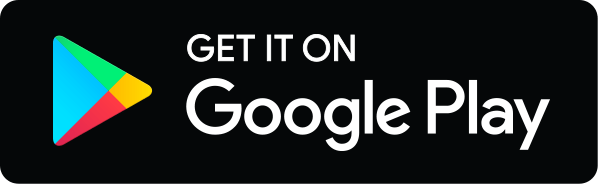