15
Clinical Neurophysiology: Future Role in Pediatric Neurologic Disorders
Clinical neurophysiology (CNP) offers a unique method of analyzing the nervous system. Though the anatomy can be visualized in many different ways, the best and most common way of evaluating the function of the nervous system is with CNP. Most often, neurophysiologic procedures are used to help diagnose neurologic diseases. Though neurophysiologic abnormalities are often nonspecific, neurophysiologists are called upon to help confirm suspected diagnoses or suggest elusive ones. The field is growing rapidly, as evidenced by the increase in the number of publications each year (1).
The future role of CNP in pediatric (and adult) neurologic diseases will most certainly evolve from where it is today. Advances in neuroimaging and molecular and genetic testing have allowed much more specific diagnoses than allowed by CNP procedures. This coupled with advances in technology and the availability of new procedures is making the future of CNP more exciting than its past was. In this chapter, some ways in which CNP will evolve and the factors responsible for it are discussed. The discussion is not meant to be comprehensive, and many innovative CNP techniques are not reviewed due to space limitations. However, some of the more significant ways that CNP will be used in the future are noted.
HISTORICAL PERSPECTIVE
CNP has evolved much in the last 75 years. In the middle of the last century, though much had already been learned about electrical activity in the nervous system, clinical applications of neurophysiologic techniques were still in their infancy. Early neurophysiologists used machines and electrodes they made themselves (2,3). Oscilloscopes were used to display signals, and special paper was used to create hard copies (4). In the early and mid-20th century, the true utility of diagnostic CNP had yet to be realized. At the time, electroencephalography (EEG) and electromyography (EMG) were still research tools available only in large universities. That these tools could be used to diagnose many neurologic conditions had not yet been fully appreciated. Indeed, some of the initial diagnostic applications ended up not being as useful as originally thought; early investigators thought EEG would be helpful in determining the etiology of various psychopathologies (5).
In the latter part of the last century, CNP matured as a discipline and its value in the diagnosis of many neurologic conditions became well established. It was the primary method of objectively viewing the nervous system. The use of evoked potentials (EPs) allowed neurophysiologists the opportunity to analyze parts of the nervous system that were difficult to evaluate with other available methods, such as the spinal cord. Specialized training became necessary to properly interpret these tests so that accurate diagnostic information could be obtained from them. Certification examinations were created to test practitioners’ proficiencies (6).
With maturity came the recognition that there were limits to the diagnostic utility of neurophysiologic testing. Despite their increasing popularity and utility, it was recognized that abnormalities detected by neurophysiologic tests were often nonspecific. This was particularly true for abnormalities detected with EPs (7). Moreover, in some situations, the sensitivity was limited as well. This was demonstrated by the relatively low sensitivity of a single EEG in diagnosing epilepsy (8). Another less well-recognized example is the low sensitivity and specificity of the multiple sleep latency test (MSLT) in the diagnosis of narcolepsy (9). These limitations were not unique to CNP; all tests used in medical diagnosis have limitations of sensitivity and specificity.
The improvements in electronics and integration of computers in medical testing allowed additional remarkable advances in CNP. Better amplifiers and commercially available equipment brought standardization to the field. Computers allowed the ability to digitize CNP data that were previously analog. Instead of paper, digital methods of displaying and storing data became available. Not only did this allow neurophysiologic tests to be done over longer periods of time, it reduced costs as well (10). The digitization of data also allowed better analysis and quantification.
Even as CNP matured and its vital place in neurologic diagnosis became established, there was evolution in its use. With the introduction and rapid development of neuroimaging, there became available another method of visualizing the nervous system. While neurophysiologic tests demonstrated physiologic injury, neuroimaging visualized anatomical changes. One example of now CNP was affected by neuroimaging was in the field of EPs. In the 1970s, EPs were the primary method of “visualizing” abnormalities in multiple sclerosis (MS) and detecting silent lesions (7). With the introduction of magnetic resonance imaging (MRI), the value of EPs diminished, and MRI along with cerebrospinal fluid analysis became the standard for investigating and diagnosing MS (11). Other advances have also had an impact on CNP. The development and availability of molecular and genetic testing has allowed many conditions to be precisely diagnosed. While CNP tests have proved to be sensitive, they are rarely specific for a particular condition. Many genetic and metabolic neurologic disorders could only be suggested by neurophysiologic tests, while molecular and genetic testing have made specific diagnoses possible. The utility of EEG and EMG in the diagnosis of these disorders has evolved (12,13).
BETTER TECHNIQUE, BETTER ANALYSIS, BETTER INTERPRETATION
As the available equipment for performing neurophysiologic tests improves, the techniques for performing these tests have matured as well. Digital EEG acquisition techniques have allowed obtaining of data from many more electrodes in better detail. Better amplifiers have allowed improved sampling and signal resolution that was unheard of previously. Instead of 21 electrodes usually placed on the scalp, the dense array EEG uses 125 or more electrodes to generate data to help with the localization of seizure foci (source localization) (14,15). Further refinement of these techniques will lead to even better localization of ictal onset zones, which in turn will lead to better treatment for patients with epilepsy.
Concurrently, invasive EEG recordings have also improved. This type of EEG provides more than diagnosis—it aids in treatment as well. Rather than the conventional strip and grid electrodes, microelectrodes that are placed closer together are able to acquire data from much smaller areas of the cortex (16). Better electrode quality and recording characteristics also are allowing the detection of ultra-high and infra-slow frequency EEG activity. The correlation of these brain waves to pathology is being defined. Stereo-EEG is yet another advancement in invasive EEG recordings. Several stereotactically implanted depth electrodes can reach areas of the brain that are not accessible to subdural electrodes (17). These electrodes can localize epileptic zones more precisely. More widespread use of such techniques will lead to better treatment outcomes.
New methods are being used in EMG as well. Surface EMG was previously thought to add no additional value to needle analysis (18). However, with improved techniques and equipment, a more recent study found value in this technique, which offers a painless way of analysis of nerves and muscles (19). High-density surface EMG represents further enhancement of this technique. High-density analysis allows assessment of muscle fiber physiology that previously was not possible (20). While the diagnostic utility of this is not fully defined, continued research into noninvasive ways of neuromuscular assessment will lead to better diagnostic tools that will be more readily accepted by patients.
Many people believed that EPs had been supplanted by other imaging modalities. However, once again, with improved stimulation and recording techniques, EPs are now being applied for more refined assessment of the nervous system and are also finding utility in the diagnosis of nonneurologic conditions. Multifocal visual evoked potentials (mfVEPs) involve stimulation of small sectors of the visual field. This allows a more precise analysis of the optic nerve (21). Though still a research tool, mfVEP may prove to be a sensitive way to assess for optic neuritis in MS and various ophthalmologic disorders. Event-related potentials (ERPs) have long been thought of as useful in studying cognitive processing. Recent studies are showing utility of these diagnostic tests in neuropsychological disorders (21). More refinement of the various types of ERPs may lead to their adoption in diagnostic testing and monitoring therapeutic intervention in these conditions.
QUANTIFICATION OF DATA
The availability of digital acquisition of neurophysiologic data has allowed its quantification. Quantification involves processing the data through various algorithms to generate maps that reveal characteristics of the underlying data that are not evident on visual analyses of raw data. This has best been applied to EEG. Quantitative EEG has different meaning for different neurophysiologists. One way quantitative EEG has been used is to create “brain maps” of EEG data that has been processed. These brain maps change, based on various cognitive processes and can be measured when performing various tasks. In this way, it can also help monitor the effects of various therapeutic or other interventions (22). Brain maps will likely become a more valuable way of assessing higher cognitive functioning.
One of the most clinically useful applications of quantitative EEG has been in the analysis of long-term or continuous EEG (cEEG). Hours of data can be reviewed expeditiously by analyzing the quantitative EEG. This is particularly true of pediatric cEEG studies (23). Though many neurophysiologists continue to feel that review of the raw EEG is essential, the quantitative analysis certainly adds another dimension to interpretation. As the number of cEEG studies increases, algorithms for quantitative analysis improve, and the reviewers for raw EEG are limited, the need for and desirability of quantitative EEG will increase in the future.
Algorithms are also being used to quantify the number of functioning motor axons. This is process is known as motor unit number estimation (MUNE). Though MUNE has been done for many decades, recently, decomposition-enhanced spike-triggered averaging MUNE has been developed that uses computer algorithms. These quantitative assessments will prove to be valuable in studying disease evolution and response to treatment.
IMPROVED TEMPORAL RESOLUTION
Neurophysiologic studies traditionally have been done for short periods of time. Routine EEGs are typically 20 to 30 minutes, while neonatal EEGs are usually 60 minutes. EMGs provide neurophysiologic assessment of the peripheral nervous system at a finite time. Outside of video EEG (vEEG) monitoring, polysomnography (PSG) used to be the longest neurophysiologic test, lasting several hours. In the last 15 years, the utility of cEEG has become increasingly clear. Many more critically ill patients are found to be seizing when long-term EEG testing is performed as compared to 30- to 60-minute tracings (24–26). Investigations are suggesting that there may be features that are evident within the initial few minutes of the EEG that determine the likelihood of finding seizures later (27). cEEG tracings are often continued for 24 to 48 hours when a suspicion for seizures is present and longer if seizures are found. Pediatric and adult practitioners are realizing the need for cEEG monitoring, and it is rapidly becoming necessary for large hospitals to offer this type of monitoring. In addition to detecting more seizures, this improved temporal resolution is also revealing EEG patterns not appreciated before, such as stimulus-induced rhythmic, periodic, or ictal discharges (SIRPIDs) (28). The need for cEEG monitoring will continue to increase, and neurophysiologists will discover new patterns of EEG, the significance of which will have to be investigated.
The need for long-term analysis is also being realized in the assessment of sleep. While PSGs are multihour studies, they still only typically evaluate one night of sleep. Often a single night in a sleep laboratory does not reveal typical sleep. Long-term sleep-wake assessments in the form of actigraphy are becoming more popular. Not only can this test reveal abnormalities in circadian physiology, it can be useful to document hours of sleep over a multiweek period. This type of information may be necessary in various diagnostic evaluations, such as to determine the amount of sleep obtained before an MSLT (29). The need for assessing the sleep-wake schedule over several days to weeks is being recognized for various pediatric behavioral sleep disorders as well. The importance of sleep assessment can be realized by the popularity of commercially available devices that track exercise and sleep times (eg, FitBit®, others). The true sensitivity and utility of long-term sleep analysis will be further clarified in the future.
Other neurologic diseases with fluctuating signs and symptoms could also benefit from long-term neurophysiologic analysis. One such condition is myasthenia gravis. Though several neurophysiologic tests are available for diagnosis and determining the response to treatment, these tests are typically done at a given moment in time. Neurophysiologists may want to evaluate this and similar disorders over hours to days to get a better understanding of disease fluctuations that may not be evident clinically. Subtle subclinical changes may become evident that foretell clinical decompensation and allow early intervention.
EXPANDING THE SPHERE OF CLINICAL NEUROPHYSIOLOGY
The future of CNP will include an expanding sphere of how the nervous system is evaluated neurophysiologically. This will involve using traditional neurophysiological procedures and using them in new ways and using innovative techniques for evaluation. An example of an innovative use of a traditional neurophysiological test is the use of EPs in neurophysiologic intraoperative monitoring (NIOM). The application of EPs in NIOM enabled neurophysiologists to reduce the morbidity of many surgical procedures (30–32). NIOM continues to expand, and neurophysiologists are called upon to participate in many types of surgeries in which the nervous system is at risk. This field will continue to expand, limited only by the number of neurophysiologists wanting to pursue it (33).
Noninvasive brain stimulation is another exciting area of growth for neurophysiologists. Transcranial magnetic stimulation (TMS), a type of noninvasive brain stimulation, has evolved from being a research technique to being both a diagnostic and therapeutic tool. Moreover, it brings together and enhances collaboration between researchers from many different disciplines. TMS has allowed neurophysiologic assessment of pathways that were previously not possible in awake subjects (34). It has increased the pathophysiologic understanding of many neurologic disorders (35). Recalcitrant psychiatric disorders have also been treated with TMS (36). Moreover, it can also be used in the assessment of efficacy of drugs. This exciting neurophysiologic modality is just beginning to be integrated into clinical practice, and the future will see it increasing in popularity.
Magnetoencephalography (MEG) is another technique that has been available for decades, but more recently, its utility in evaluating patients for epilepsy surgery has become better defined. It serves as a useful complement to EEG in localizing neocortical epileptic zones (37). There are other uses for MEG as well. It has been used to study cognitive processes in fetuses and infants. Detection of problems can lead to earlier and more effective early intervention programs (38). The clinical availability of commercial MEG has certainly fueled its progress. Future neurophysiologists will be poised to further exploit this technology to help patients.
New techniques are also becoming available to investigate muscles and nerves. Electrical impedance myography (EIM) uses electricity to image muscles. Though currently still a research tool, EIM may lead to better diagnostic testing and longitudinal assessment of neuromuscular disorders (39). More research in the future will enable this technology to become integrated into clinical practice.
NEW DISEASES, BETTER ASSESSMENTS
The future of CNP will see neurophysiologists using their tools to assess conditions that have not traditionally been evaluated with neurophysiologic methods. TMS offers one of the best ways to study the pathophysiology of movement disorders like Parkinson’s disease (PD) and dystonia (35). This technique has allowed investigators to study various symptoms of these conditions in detail, which hopefully will lead to better and more targeted therapies.
Various pain syndromes lend themselves well for analysis by neurophysiologic methods. Cortical hyperexcitability and hypoexcitability, also referred to as “dysexcitability,” has been studied with EPs and TMS (40). Though conflicting results have been found, several theories about pathogenesis have emerged. Further investigations into this and other pain syndromes with newer techniques may bring these disease states into the clinical domain of the neurophysiologist.
Psychiatric conditions such as depression can be treated with TMS. Evaluation of some psychiatric conditions with TMS and other types of neurophysiologic tests may reveal clues to their pathophysiology. Conversion disorder and hysteria have been studied with a variety of neurophysiologic procedures, including cognitive EPs, EEGs, and MEGs. These studies have led to several theories that explain the cause of hysterical paralysis (41). More studies of this type will yield insights into the pathophysiology of not only neurologic but psychiatric conditions as well.
NEUROPHYSIOLOGY AS A BIOMARKER
Perhaps one of the most exciting advances in CNP has been the use of various neurophysiologic procedures as biomarkers of disease progression and severity. Though seemingly a natural fit, EEG has not been used much as a biomarker in treatment trials for seizures until recently. This is not surprising given the uncertain relationship between interictal abnormalities, epilepsy disease severity, and seizure control. With the availability of cEEG monitoring and the realization of frequent seizures in critically ill patients, the role of EEG as a biomarker has become possible. Recently a multicenter, randomized trial comparing the efficacy of antiepileptic drugs (AEDs) in the treatment of nonconvulsive seizures was completed (42). This was the first study to use EEG as a biomarker to evaluate the relative effectiveness of AEDs in critically ill patients. Other trials are already underway using EEG as a biomarker for treatment response of super refractory status epilepticus (SRSE) (43). SRSE is a diagnosis based on EEG, so it is certainly logical that treatment trials would use EEG as a measure of treatment success. The use of EEG is not without challenges, however. Interpretation of this test is still largely subjective; at times, consensus on what constitutes pathology (seizures) may be elusive. The use of EEG as a biomarker in epilepsy and status epilepticus studies has just started. The future hopefully will see many more similar clinical trials.
EPs have also found utility as biomarkers in clinical trials (44). MS/optic neuritis trials have used pattern reversal VEP to assess myelination of the optic nerve (45). Optic nerve conduction (VEP P100 latency) changes were used in a clinical trial as an objective way to compare the degree of remyelination with active drug versus placebo (46).
TMS has also been used as a biomarker. It has been combined with EMG and EEG to study the effect of drugs on muscles and the brain (47). TMS-EMG and TMS-EEG studies are also being used to predict which patients may respond to a particular therapy. This field is in its infancy, and in the future, it may enable physicians to better select medications for certain diseases.
The role of CNP in clinical trials is just being appreciated. Neurophysiologic tests offer an objective method of assessing symptoms that are currently assessed only subjectively. This may help remove a lot of noise from clinical trials and enable demonstration of therapeutic effect more clearly and with fewer subjects. Neurophysiologists will be well served in pursuing the use of neurophysiologic procedures as biomarkers in the assessment of therapy for neurologic and psychiatric disorders.
NONSCIENTIFIC INFLUENCES
Like any other medical discipline, nonscientific issues will also affect the future of CNP. Foremost among these influences are economic issues. Reimbursements for neurophysiologic procedures unfortunately affect how and how often they are performed. Favorable reimbursements may see an overuse of various procedures, while cuts may limit their availability. The last several years have seen government regulators reduce reimbursements for EMG/NCS (nerve conduction studies) and NIOM (48,49). How much this will affect patient care remains to be seen. It will undoubtedly have an immeasurable effect on students considering a career in CNP. These economic issues are most acute in the United States, but other practice settings are not immune to this either. Clearly, these issues will affect the future of CNP, but exactly how is unclear.
Naturally, clinical training in CNP will affect the future of the field as well. Though countries vary on how training is imparted, most developed countries have an organized process by which trainees can obtain specialization in this field. In the United States, formal fellowship programs provide the requisite training that is overseen by regulatory agencies. However, many training programs provide education in the more established disciplines in CNP, such as EEG and EMG. Few focus on fields such as NIOM or TMS (33). Professional trade organizations also provide education in CNP, and recently they have recognized the value in having programs in emerging CNP disciplines. More education in all areas of CNP and in research methods will be critical in ensuring further development of this field.
Education and training are important for not only physicians but also technologists. CNP technology has become too complex for the physicians to also serve as technologists, as was the case in the era of the pioneers of the field (50). Though board certification/registration examinations at basic and advanced levels are available for technologists, formal training programs are few and the number of graduates produced insufficient to maintain and grow CNP departments. Such is the situation in the United States; elsewhere in the world, the shortage of qualified technologists is even more acute. Training individuals on the job to perform these highly complex procedures often fills this void. While this helps ameliorate an acute shortage, it is not a viable long-term solution. Neurophysiologists will have to direct their attention to this problem urgently. Appropriate training programs, perhaps at a bachelor’s or master’s level, will be important to create and encourage a constant stream of qualified, professional technologists. Without this support staff, further progress in CNP will be greatly hampered.
CONCLUSIONS
The era of using CNP simply to diagnose neurologic disorders is coming to a close. No longer will neurophysiologists be content with providing a differential diagnosis for a condition or even diagnosing a disease; they will be using their procedures in many other ways. More traditional CNP tests will be used in novel ways to more accurately diagnose diseases. Long-term use of these tests will provide longitudinal data on disease progression. New neurophysiologic procedures are opening new chapters in diagnosis, management, and treatment of neurologic and psychiatric diseases that had not previously been evaluated with CNP. Abnormalities detected on neurophysiologic tests are being used as biomarkers of disease; these can be used in clinical trials and other ways to monitor the progression of a disease or response to treatment. Nonscientific influences will also affect CNP. The future of CNP is bright, and there remain many exciting opportunities for research, education, and teaching for young neurophysiologists.
REFERENCES
1. IFCN. Research & guidelines. http://www.ifcn.info/showcontent.aspx?MenuID=1137. Cited December 24, 2014.
2. Haas LF. Hans Berger (1873–1941), Richard Caton (1842–1926), and electroencephalography. J Neurol Neurosurg Psychiatry. 2003;74(1):9.
3. Kazamel M, Province P, Alsharabati M, Oh S. History of electromyography (EMG) and nerve conduction studies (NCS): a tribute to the founding fathers. Neurology. 2013;80(Meeting abstracts 1):P05.259.
4. Ladegaard J. Story of electromyography equipment. Muscle Nerve Suppl. 2002;11:S128–S133.
5. Schirmann F. “The wondrous eyes of a new technology”-a history of the early electroencephalography (EEG) of psychopathy, delinquency, and immorality. Front Hum Neurosci. 2014;8:232.
6. ABPN. Mission and history. http://www.abpn.com/mission_and_history.html. Cited December 26, 2014.
7. Aminoff MJ. The clinical role of somatosensory evoked potential studies: a critical appraisal. Muscle Nerve. 1984;7(5):345–354.
8. Hoefnagels WA, Padberg GW, Overweg J, et al. Syncope or seizure? The diagnostic value of the EEG and hyperventilation test in transient loss of consciousness. J Neurol Neurosurg Psychiatry. 1991;54(11):953–956.
9. Goldbart A, Peppard P, Finn L, et al. Narcolepsy and predictors of positive MSLTs in the Wisconsin Sleep Cohort. Sleep. 2014;37(6):1043–1051.
10. Husain AM, Hope VT, Mebust KA, et al. Cost analysis of analog versus digital electroencephalography. Am J Electroneurodiagnostic Technol. 1998;11:144–147.
11. McDonald WI, Compston A, Edan G, et al. Recommended diagnostic criteria for multiple sclerosis: guidelines from the International Panel on the diagnosis of multiple sclerosis. Ann Neurol. 2001;50(1):121–127.
13. Swash M. What does the neurologist expect from clinical neurophysiology? Muscle Nerve Suppl. 2002;11:S134–S138.
14. Holmes MD, Tucker DM, Quiring JM, et al. Comparing noninvasive dense array and intracranial electroencephalography for localization of seizures. Neurosurgery. 2010;66(2):354–362.
15. Lantz G, Grave de Peralta R, Spinelli L, et al. Epileptic source localization with high density EEG: how many electrodes are needed? Clin Neurophysiol. 2003;114(1):63–69.
16. Schevon CA, Ng SK, Cappell J, et al. Microphysiology of epileptiform activity in human neocortex. J Clin Neurophysiol. 2008;25(6):321–330.
17. Panzica F, Varotto G, Rotondi F, et al. Identification of the Epileptogenic Zone from Stereo-EEG Signals: A Connectivity-Graph Theory Approach. Front Neurol. 2013;4:175.
18. Haig AJ, Gelblum JB, Rechtien JJ, Gitter AJ. Technology assessment: the use of surface EMG in the diagnosis and treatment of nerve and muscle disorders. Muscle Nerve. 1996;19(3):392–395.
19. Meekins GD, So Y, Quan D. American Association of Neuromuscular & Electrodiagnostic Medicine evidenced-based review: Use of surface electromyography in the diagnosis and study of neuromuscular disorders. Muscle Nerve. 2008;38(4):1219–1224.
20. Drost G, Stegeman DF, van Engelen BG, Zwarts MJ Clinical applications of high-density surface EMG: A systematic review. J Electromyogr Kinesiol. 2006;16(6):586–602.
21. Grover LK, Hood DC, Ghadiali Q, et al. A comparison of multifocal and conventional visual evoked potential techniques in patients with optic neuritis/multiple sclerosis. Doc Ophthalmol. 2008;117(2):121–128.
22. Michel CM, Murray MM. Towards the utilization of EEG as a brain imaging tool. Neuroimage. 2012;61(2):371–385.
23. Stewart CP, Otsubo H, Ochi A, et al. Seizure identification in the ICU using quantitative EEG displays. Neurology. 2010;75(17):1501–1508.
24. Jette N, Claassen J, Enerson RG, Hirsch LJ. Frequency and predictors of nonconvulsive seizures during continuous electroencephalographic monitoring in critically ill children. Arch Neurol. 2006;63(12):1750–1755.
25. Shafi MM, Westover MB, Cole AJ, et al. Absence of early epileptiform abnormalities predicts lack of seizures on continuous EEG. Neurology. 2012;79(17):1796–1801.
26. Claassen J, Mayer SA, Kowalski, et al. Detection of electrographic seizures with continuous EEG monitoring in critically ill patients. Neurology. 2004;62(10):1743–1748.
27. Swisher CB, Shah D, Sinha SR, Husain AM. Baseline EEG pattern on continuous ICU EEG monitoring and incidence of seizures. J Clin Neurophysiol. 2015;32(2):147–151.
28. Hirsch LJ, Claassen J, Mayer SA, Enmerson RG. Stimulus-induced rhythmic, periodic, or ictal discharges (SIRPIDs): a common EEG phenomenon in the critically ill. Epilepsia. 2004;45(2):109–123.
29. Baumann CR, Mignot E, Lammers GJ, et al. Challenges in diagnosing narcolepsy without cataplexy: a consensus statement. Sleep. 2014;37(6):1035–1042.
30. Nuwer MR, Dawson EG, Carlson LG, et al. Somatosensory evoked potential spinal cord monitoring reduces neurologic deficits after scoliosis surgery: results of a large multicenter survey. Electroencephalogr Clin Neurophysiol. 1995;96(1):6–11.
31. Radtke RA, Erwin CW, Wilkins RH. Intraoperative brainstem auditory evoked potentials: significant decrease in postoperative morbidity. Neurology. 1989;39(2 Pt 1):187–191.
32. Nuwer MR, Emerson RG, Galloway G, et al. Evidence-based guideline update: intraoperative spinal monitoring with somatosensory and transcranial electrical motor evoked potentials: report of the Therapeutics and Technology Assessment Subcommittee of the American Academy of Neurology and the American Clinical Neurophysiology Society. Neurology. 2012;78(8):585–589.
33. Husain AM, Emerson RG, Nuwer MN. Emerging subspecialties in neurology: neurophysiologic intraoperative monitoring. Neurology. 2011;76(15):e73–e75.
34. Galloway GM, Dias BR, Brown JL, et al. Transcranial magnetic stimulation–may be useful as a preoperative screen of motor tract function. J Clin Neurophysiol. 2013;30(4):386–389.
35. Hallett M, Rothwell J. Milestones in clinical neurophysiology. Mov Disord. 2011;26(6):958–967.
36. Slotema CW, Blom JD, Hoek HW, Sommer IE. Should we expand the toolbox of psychiatric treatment methods to include Repetitive Transcranial Magnetic Stimulation (rTMS)? A meta-analysis of the efficacy of rTMS in psychiatric disorders. J Clin Psychiatry. 2010;71(7):873–884.
38. Sheridan CJ, Matuz T, Draganova R, et al. Fetal Magnetoencephalography – Achievements and Challenges in the Study of Prenatal and Early Postnatal Brain Responses: A Review. Infant Child Dev. 2010;19(1):80–93.
39. Rutkove SB. Electrical impedance myography: Background, current state, and future directions. Muscle Nerve. 2009;40(6):936–946.
40. Cosentino G, Fierro B, Brighina F. From different neurophysiological methods to conflicting pathophysiological views in migraine: A critical review of literature. Clin Neurophysiol. 2014;125(9): 1721–1730.
41. Crommelinck M. Neurophysiology of conversion disorders: A historical perspective. Neurophysiol Clin. 2014;44(4):315–321.
42. Husain AM. Treatment of Recurrent Electrographic Nonconvulsive Seizures (TRENdS) study. Epilepsia. 2013;54 Suppl 6:84–88.
43. ClinicalTrials.gov. Study to evaluate SAGE-547 as adjunctive therapy for the treatment of super-refractory status epilepticus (SRSE). https://clinicaltrials.gov/ct2/show/NCT02052739?term=sage+547&rank=3. Cited December 26, 2014.
44. Kraft GH. Evoked potentials in multiple sclerosis. Phys Med Rehabil Clin N Ame. 2013;24(4):717–720.
45. Fernández O. Integrating the tools for an individualized prognosis in multiple sclerosis. JNeurol Sci. 2013;331(1–2):10–13.
46. ClinicalTrials.gov. 215ON201 BIB033 in acute optic neuritis (AON) (RENEW). https://clinicaltrials. gov/ct2/show/NCT01721161?term=lingo&rank=1. Cited December 26, 2014.
47. Ziemann U, Reis J, Schwenkreis P, et al. TMS and drugs revisited 2014. Clin Neurophysiol. 2014; pii: S1388-2457(14)00837-2.
48. Emerson RG, Husain AM. Blurring of local and remote practice models threatens IOM’s future. Neurology. 2013;80(12):1076–1077.
49. Penz K. Medicare cuts impact access to care. http://www.aanem.org/About-Us/News/Reimbursement-Cuts-Impact-Access-to-Care.aspx. Cited December 26, 2014.
50. ASET. Competencies. http://www.aset.org/i4a/pages/index.cfm?pageid=3612. Cited December 26, 2014.
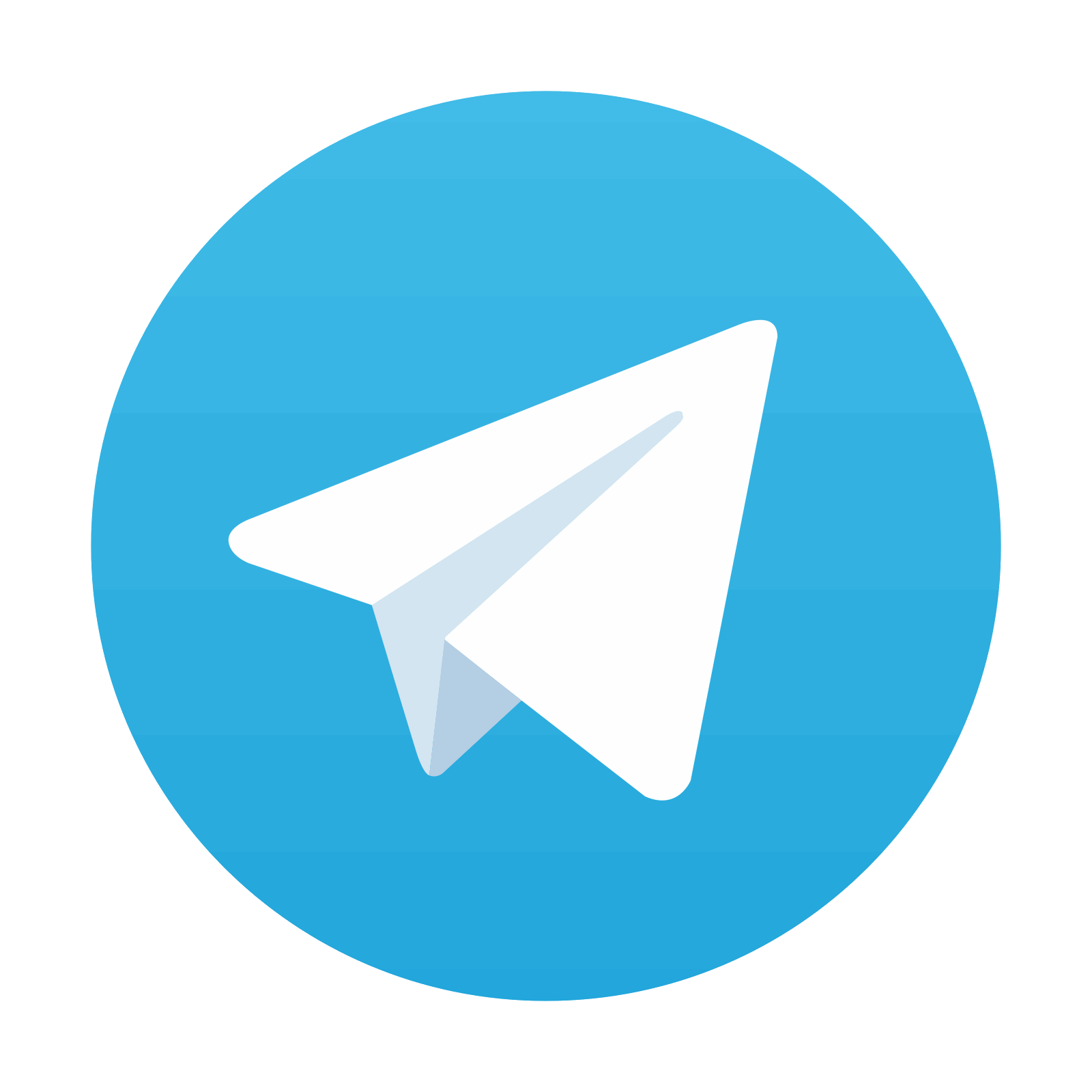
Stay updated, free articles. Join our Telegram channel

Full access? Get Clinical Tree
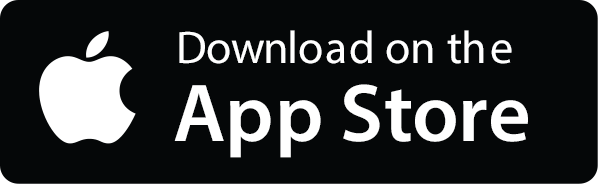
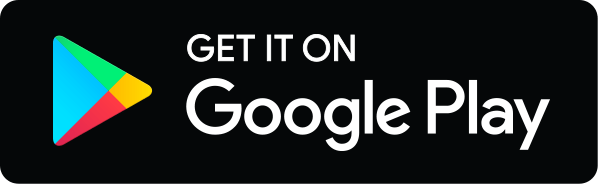